Pharmacological Regulation of Cardiac Function PDF - 2022
Document Details
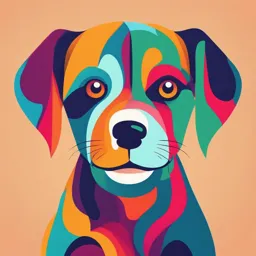
Uploaded by FruitfulIntegral
Wayne State University
2022
Rodrigo Andrade
Tags
Summary
These are lecture notes covering the pharmacological regulation of cardiac function, including excitation-contraction coupling, sympathetic regulation, calcium channels, and treatment of heart failure. The notes cover various pharmacological mechanisms and their effects on the heart and its contractility.
Full Transcript
Pharmacological regulation of cardiac function Rodrigo Andrade, 3108 Scott Hall. October 2022 Objectives To understand the mechanisms by which sympathetic stimulation increases cardiac contractility and their pharmacology To learn the prototypical calcium channel blockers and u...
Pharmacological regulation of cardiac function Rodrigo Andrade, 3108 Scott Hall. October 2022 Objectives To understand the mechanisms by which sympathetic stimulation increases cardiac contractility and their pharmacology To learn the prototypical calcium channel blockers and understand their effects and differences. To understand the mechanism of action and pharmacology of cardiac glycosides. To become familiar with the concept of calcium sensitizers To learn the fundamental pharmacological used to treat heart failure. Lecture outline (lecture timing marks) Introduction (00:00) I. Excitation-contraction coupling in the heart (00:18) II. Sympathetic regulation of cardiac calcium channels (04:07) III. Pharmacological regulation of heart function. Adrenergic Mechanisms (10:53) Calcium channel blockers (14:29) Cardiac glycosides (20:37) Beyond calcium (27:00) IV. Treatment of Heart Failure (29:30) End (41:15) Introduction In the previous lecture we learned about the cardiac action potential and the many pharmacological avenues available to regulate the electrical activity of the heart. But, of course, the electrical activity is only part of the story; we need to also consider the contractility of the heart. This is most important for the treatment of heart failure, when the heart’s ability to pump blood becomes compromised. I. Excitation-contraction coupling in the heart A key aspect of the cardiac action potential is the influx of calcium through L-type calcium channels, mostly during phase 2 and 3. In this context we considered calcium as a charge carrying ion, but calcium is also an important “second messenger” that links events at the cell membrane with a variety of intracellular processes. In the case of the heart, calcium links membrane depolarization with contraction. Figure 1. The cardiac action potential. As action potentials spread through the heart, they travel along the surface of the myocytes, invade the T tubules (Fig. 2) and activate L type calcium channels. This leads to an influx of calcium into the myocyte (trigger calcium) which activates calcium release channels (Ryanodine receptors) on the sarcoplasmic reticulum, and results in the release of calcium from sarcoplasmic reticulum (intracellular calcium stores) (Fig. 2). This process is generally referred to as calcium-induced calcium release (CICR) and leads to a large transient increase in intracellular calcium concentration. This in turn activates Troponin C and generates muscle Figure 2. Excitation-contraction coupling in the heart contraction. Calcium is then sequestered back into the sarcoplasmic reticulum by an ATP powered calcium pump (SERCA, sarco-endoplamic reticulum calcium ATPase) and secondarily extruded from the cell by an electrogenic sodium-calcium exchange pump (NCX). II. Sympathetic regulation of cardiac calcium channels Sympathetic stimulation strongly regulates this process to increase the strength of contraction (inotropic effect). This complements the effects on the pacemaker current to increase heart rate (chronotropic effect) and increases cardiac output. Figure 3. β-adrenergic facilitation of L type calcium channel function in the heart Figure 4. Sympathetic stimulation facilitates IKs and shortens the duration of the action potential. (from Terrenoise et al., Circ Res 18:e25-2e34, 2005) Sympathetic stimulation activates β-adrenergic receptors, which act via Gs and adenylate cyclase, to elicit a rise in intracellular cAMP and activate Protein Kinase A (PKA). This kinase in turn phosphorylates the L type calcium channel to produce and increase in the calcium current (Fig. 3). In the SA and AV nodes this results in an increase the rate of rise of the action potential and also the conduction velocity of the action potential. Importantly, these effects are balanced by a concomitant upregulation of IKs which shorten the action potential, again supporting an increase in heart rate and conduction (Fig. 4). Figure 5. Sympathetic regulation of excitation contraction coupling in the heart In terms of the inotropic effects, the phosphorylation of L type calcium channels leads to an increase in calcium influx which facilitates CICR. This in turn results in an increase in the intracellular calcium rise triggered by the action potential and therefore an increase in the force of contraction (Fig 5). PKA however also phosphorylates phospholamban, an inhibitor of SERCA, and also Troponin I which regulates the calcium sensitivity of Troponin C. The phosphorylation of phospholamban suppresses its ability to inhibit SERCA, thus increasing the rate of calcium reuptake into the SR. This results in an increase in the speed and efficiency with which SERCA sequesters cytoplasmic calcium. The phosphorylation of Troponin I impairs its interaction with Troponin C thus leading to a decreased calcium sensitivity for the myofilament. Combined these two effects increase the rate of relaxation of the myocytes, again supporting the increased heart rate. III. Pharmacological regulation of heart function. Adrenergic Mechanisms. Mimicry and antagonism of sympathetic signaling in the heart offer an effective avenue for the regulation of cardiac contractility (inotropic effect) as well as firing rate (chronotropic effect). Receptor subtype nonselective β-adrenergic agonists such as isoproterenol, or selective 1- adrenergic receptors agonists such as dobutamine will induce not only an increase in heart rate but also an increase in the strength of contraction (positive inotropic effect). Conversely, administration of non-selective β-adrenergic blockers such as propranolol, or selective 1- adrenergic blockers such as atenolol or metoprolol will induce a negative chronotropic as well as a negative inotropic effect by antagonizing ongoing sympathetic stimulation of the heart. β-adrenergic receptors, including those of the β1 subtype, act through Gs to activate adenylate cyclase, and increase intracellular cAMP. cAMP has a limited lifetime in the cytoplasm as it is hydrolyzed by phosphodiesterases (PDEs). Thus, PDEs control the concentration (and probably sculpt the subcellular distribution) of cAMP in cardiac myocites. PDEs constitute a large family of proteins capable of hydrolyzing cAMP, cGMP or both. In the heart and the vasculature, the PDE isozyme PDE3 plays an important role regulating cAMP levels. Thus, PDE inhibitors constitute an alternative way to bypass the beta receptor to Figure 6. Milrinone inhibits PDE3 in the heart regulate heart rate and contractility (Fig. 6). Milrinone is a PDE3/PDE4 inhibitor that potentiates the effects downstream from beta receptor stimulation and is sometimes used in the treatment of advanced cardiac failure. Calcium channels blockers Calcium channel blockers such as Verapamil, Diltiazem and the dihydropyridines (e.g. Nifedipine, Amlodipine) target L type calcium channels on the plasma membrane (Fog. 7). As such they can reduce the contribution of the calcium current to the action potential duration and also reduce calcium influx, thus limiting the force of contraction. These drugs tend to have only minimal effects on T type calcium channels. Figure 7. Calcium channel Blockers Verapamil, Diltiazem and the dihydropyridines have limited in vitro pharmacological selectivity for different L-type calcium channels. However, at therapeutic doses the dihydropyridines preferentially target the vasculature and therefore we refer to them as “vascular selective” (Fig. 8). The exact reason for this selectivity is not completely understood. The L type calcium channels are heterogeneous and include three distinct isoforms. These different L-type calcium channels differ somewhat in their voltage dependence, and they are also differentially spliced in heart and vascular smooth muscle, so that could contribute to their differential regulation by on atrial appendage Figure 8.vasorum on vasa Vascular felodipine selectivity of dihydropyridines nifedipine amlodipine verapamil dihydropyridines. Alternatively, verapamil and diltiazem block the reflex sympathetic activation that follows the dihydropyridine-induced vasodilation (Fig. 9) and that could also account for the observed difference. Calcium channel blockers, due to their cardiac and vascular effects, are used to treat a variety of conditions including angina, hypertension and some arrhythmias (e.g. atrial fibrillations). Figure 9. Differential effects of dihydropyridines vs verapamil and diltiazem Cardiac glycosides The strength of contraction is determined by the concentration of intracellular calcium, and therefore one potential avenue for increasing the strength of contraction is to increase the rise in intracellular calcium triggered by the action potential. Calcium extrusion relies in part on the sodium-calcium exchanger (NCX) which uses the transmembrane sodium gradient to pump calcium across the plasma membrane and out of the cell. Cardiac glycosides such as Digoxin are potent inhibitors of the Na/K ATPase and thus lead to intracellular sodium accumulation (Fig 10). This reduces the driving force for sodium and thus the ability of NCX to pump calcium out of the cell. This in turn leads to higher calcium loading of the intracellular calcium stores, larger calcium transients and consequently an increase in the strength of contraction. Digoxin was used for many years in the treatment of heart failyre but its clinical use is now very limited. As expected for a Na+/K+ ATPase inhibitor, Digoxin exhibits a very narrow therapeutic index. Serum therapeutic concentrations range from 0.5 to 1.5 ng/ml while toxicity may appear at concentrations as low as 2 ng/ml. Digoxin binds the potassium binding site of the Na+/K+ ATPase and thus its potency (and toxicity) are magnified by hypokalemia. One particularly serious adverse effect of the cardiac glycoside emerges from their effect on intracellular calcium stores. Since NCX is electrogenic (it translocates one calcium ion for every three sodium ions), calcium release from intracellular stores result in the development of an inward current. This is usually of little functional significance. However, in the presence of cardiac glycosides the sarcoplasmic reticulum calcium stores become overfilled, which makes them much more likely to release calcium into the cytoplasm not only during the action potential but also Figure 10. Effect of Digoxin on NCX and intracellular calcium afterwards with a delay (Fig 9). The resulting activation of NCX , as it pumps calcium out of the cell, induces the appearance of a inward current that mediates delayed afterdepolarizations (DADs) and produce a triggered arrhythmias (Fig. 11). Figure 11. Activation of NCX can trigger delayed afterdepolarizations. Beyond calcium. The inotropes (drugs used to induce a positive inotropic effect) addressed above all work by mobilizing intracellular calcium. This is associated with a number of limitations including increased oxygen demands due to the need to pump the additional calcium, the danger of inducing arrythmias, and facilitation of cardiac remodeling (see below). It has been suggested that one way to get around these problems would be to use drugs capable of potentiating the effect of calcium on the contractile apparatus (calcium sensitizers). Three current drugs fall into this class, although none of them is approved for human use in the US. Pimovendan is an inhibitor of PDE3 that also sensitizes cardiac myofilaments to intracellular calcium. This drug was initially shown to reduce hospitalizations and improve quality of life, but in subsequent clinical trials was found to increase mortality and drug development in the US was discontinued. Pimovenda, however, is currently approved for clinical use in Japan. Levosimendan increases cardiac contractility by binding troponin C and also activated KATP potassium channels. This drug has been shown to improve hemodynamics without increasing oxygen consumption and appears to be at least neutral and perhaps protective in term of mortality. It is currently approved for clinical use in Europe and Latin America but not in the US. Omacamtiv mecarbil is a cardiac “myosin activator” that facilitates the generation of force in the heart. Importantly, a large randomized clinical study in 2021 showed modest but significant clinical benefits from this drug. Omacamtiv mecarbil is currently being considered for approval by the FDA for the treatment of heart failure. IV. Treatment of Heart Failure Heart failure is a complex syndrome where the heart fails to pump blood at the rate required by the body. We recognize to main types forms of heart failure, Heart Failure with reduced Ejection Fraction (HFrEF, aka systolic heart failure) and Heart Failure with preserved Ejection Fraction (HFpEF, aka diastolic heart failure). In HFrEF the ability of the left ventricle to pump blood is compromised and consequently the left ventricular ejection fraction, which is normally of the order of the 50% to 70%, is reduced to 40% or less. In contrast, in HFpEF blood outflow from the heart is reduced secondarily to a decrease in filling volume, while the left ventricular ejection fraction remains in the normal range (≥50%). HFrEF responds reasonably well to pharmacological management, while HFpEF respond less well. Inotropes would appear to be well suited for treating these conditions. In fact these drugs- especially cardiac glycosides- were for many years the treatment of choice for heart failure. However, it is now clear thatthese drugs, while providing some symptomatic relief for heart failure patients, and also being useful supporting organ perfusion in critical cases, failed to increase survival. The reason for this failure is a the development of a process now widely known as cardiac remodeling. Figure 12. Pathological remodeling of the heart In a nutshell, an acute drop of blood pressure or organ perfusion leads to the activation of the sympathetic nervous system (SNS), the renin-angiotensin-aldosterone (RAAS) system, atrial natriuretic peptide release, and others feedback signals that together orchestrate a series of physiological changes across multiple organ systems aimed to compensate for the drop in organ perfusion. While these changes serve a very useful function in allowing our bodies to respond to acute perturbations in blood pressure/perfusion, when these processes are activated on a chronic basis -for example in response to tissue loss due to myorcardial infarction or a chronic rise in vascular resistance, these changes become maladaptative (Fig. 12). Thus, the main aim of heart failure therapy is to stop this pathological remodeling. Modern Pharmacological treatment HFrEF do not target the heart contractility directly. Rather, it aims to block the remodeling process and thus relies on drugs primarily targeting the vasculature and the kidneys. They include four main pharmacological approaches: Angiotensin Converting Enzyme inhibitors (ACE inhibitors), Angiotensin Receptor Blockers (ARBs) and especially the combination of an ARB with an inhibitor of neprilysin, the enzyme that degrades natriuretic peptides (ARNi). Beta Blockers (bisoprolol, carvedilol, and sustained release metoprolol) Mineralocorticoid Receptor Antagonists (MRAs) Sodium-glucose cotransporter 2 inhibitors (SGLT2i). A detailed discussion of these drugs is outside the scope of the current lecture and you will learn more about these drugs in the upcoming vascular pharmacology lecture, and also in the kidney and endocrine Pharmacology lectures that will follow later. The mechanisms thought to underlie the action of the first 3 drug classes is sketched in figure 12 and generally target known mechanisms involved in the regulation of blood pressure. Less is known about the mechanisms by which SGLT2is induce their therapeutic response. SGLT2is are used in the treatment of diabetes, and their impressive effectiveness in the treatment of heart failure was discovered serendipitously during their initial clinical trials for the treatment of diabetes. Subsequent work confirmed that their effectiveness was independent of the presence of the diabetes or sugar level control. SGLT2is are the only drug class recommended for the treatment of HFpEF in the latest treatment recommendations of the AHA1. 1. Heidenreich et al., AHA/ACC/HFSA CLINICAL PRACTICE GUIDELINE- 2022 AHA/ACC/HFSA Guideline for the Management of Heart Failure: A Report of the American College of Cardiology/American Heart Association Joint Committee on Clinical Practice Guidelines. Circulation. 2022;145:e895–e1032. DOI: 10.1161/CIR.0000000000001063