Pharm L2 PDF
Document Details
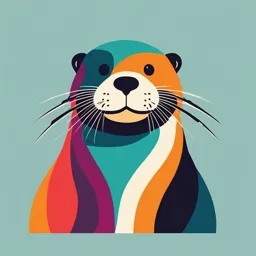
Uploaded by CelebratedCosine
Columbia University School of Nursing
Tags
Summary
This document provides an overview of neuropharmacology, focusing on the peripheral and central nervous systems. It discusses different types of neurons, neurotransmitters, and their corresponding receptors. The document also categorizes drugs that act on the nervous system and describes their effects on various bodily functions.
Full Transcript
Week 2: PNS Neuropharmacology Any drugs that alter process controlled by nervous systems (suppression or excitation of neuronal Let’s Get Organized Central Nervous System (CNS) Components: Brain and spinal cord. Function: The CNS processes information and is the control center fo...
Week 2: PNS Neuropharmacology Any drugs that alter process controlled by nervous systems (suppression or excitation of neuronal Let’s Get Organized Central Nervous System (CNS) Components: Brain and spinal cord. Function: The CNS processes information and is the control center for the body. Peripheral Nervous System (PNS) Components: All nerve cells outside the CNS. Function: Connects the CNS to the limbs and organs, relaying information to and from the brain and spinal cord. Subdivisions of the PNS 1. Somatic Nervous System (SNS) ○ Function: Controls voluntary movements. ○ Components: Motor neurons that control skeletal muscles. 2. Autonomic Nervous System (ANS) ○ Function: Regulates involuntary body functions such as heart rate, digestion, respiratory rate, and reflexes. ○ Components: Divided into the sympathetic and parasympathetic nervous systems. Divisions of the Autonomic Nervous System 1. Sympathetic Nervous System (SNS) ○ Function: Prepares the body for stressful or emergency situations (fight or flight response). ○ Effects: Increases heart rate, dilates airways, and releases adrenaline. 2. Parasympathetic Nervous System (PNS) ○ Function: Conserves energy and restores the body to a state of calm (rest and digest response). ○ Effects: Decreases heart rate, stimulates digestion, and promotes relaxation. Diagram Representation 1. Nervous System ○ CNS: Brain and spinal cord ○ PNS: All other nerve cells 2. PNS: ○ Somatic Nervous System: Controlled, voluntary actions ○ Autonomic Nervous System: Involuntary actions Sympathetic Nervous System: Fight or flight Parasympathetic Nervous System: Rest and digest Basic Principles Drugs That Act on the Peripheral Nervous System (PNS) Somatic Nervous System (SNS) Function: Controls voluntary movements of skeletal muscles. Drug Types: ○ Neuromuscular Blockers: Used during surgeries to induce muscle paralysis. Examples: Succinylcholine, rocuronium. ○ Muscle Relaxants: Used to relieve muscle spasms. Examples: Baclofen, diazepam. Autonomic Nervous System (ANS) Function: Regulates involuntary body functions. Subdivisions: 1. Sympathetic Nervous System (SNS) Function: Prepares the body for stressful or emergency situations (fight or flight response). Effects: Increases heart rate, dilates airways, releases adrenaline. Drug Types: Adrenergic Agonists: Stimulate sympathetic activity. Examples: Epinephrine, albuterol. Adrenergic Antagonists (Beta Blockers): Reduce sympathetic activity. Examples: Propranolol, metoprolol. 2. Parasympathetic Nervous System (PNS) Function: Conserves energy and restores the body to a state of calm (rest and digest response). Effects: Decreases heart rate, stimulates digestion, promotes relaxation. Drug Types: Cholinergic Agonists: Stimulate parasympathetic activity. Examples: Bethanechol, pilocarpine. Cholinergic Antagonists (Anticholinergics): Reduce parasympathetic activity. Examples: Atropine, scopolamine. The parasympathetic nervous system performs seven regulatory functions that have particular relevance to drugs. Specifically, stimulation of appropriate parasympathetic nerves causes Slowing of heart rate Increased gastric secretion Emptying of the bladder Emptying of the bowel Focusing the eye for near vision Constricting the pupil Contracting bronchial smooth muscle When we are faced with an acute stress-inducing situation, the sympathetic nervous system orchestrates the fight-or-flight response, which consists of Increasing heart rate and blood pressure Shunting blood away from the skin and viscera and into skeletal muscles Dilating the bronchi to improve oxygenation Dilating the pupils (perhaps to enhance visual acuity) Mobilizing stored energy, thereby providing glucose for the brain and fatty acids for muscles Drugs That Act on the Central Nervous System (CNS) Key Consideration: Blood-Brain Barrier (BBB) Definition: A selective barrier that protects the brain from toxins and pathogens. Requirement for CNS Drugs: Must be able to cross the BBB to be effective. Drug Types: ○ Analgesics: Pain relievers. Examples: Morphine, ibuprofen. ○ Antidepressants: Used to treat depression. Examples: Fluoxetine, sertraline. ○ Antipsychotics: Used to manage psychosis. Examples: Haloperidol, clozapine. ○ Antiepileptics: Used to control seizures. Examples: Phenytoin, valproate. Summary 1. PNS Drugs: ○ Somatic: Neuromuscular blockers, muscle relaxants. ○ Autonomic: SNS: Adrenergic agonists and antagonists. PNS: Cholinergic agonists and antagonists. 2. CNS Drugs: Must cross the BBB. Includes analgesics, antidepressants, antipsychotics, and antiepileptics. This structured overview provides a foundation for understanding the pharmacology of drugs affecting different parts of the nervous system. For further reading and more detailed examples, refer to resources like Khan Academy and Mayo Clinic. Use Next 3 Slides to Answer Study Q #1 Slide Pic 1 Neuronal Classification by Neurotransmitter and Receptor Type 1. Cholinergic Neurons ○ Neurotransmitter: Acetylcholine (ACh) ○ Receptors: Nicotinic ACh Receptors: Ligand-gated Na+/K+ channels Subtypes: NN: Found in preganglionic efferent fibers synapsing in autonomic ganglia. NM: Found in somatic motor fibers synapsing on neuromuscular junctions. Muscarinic ACh Receptors: G-protein coupled receptors Subtypes: M1, M2, M3, M4, M5 2. Adrenergic Neurons ○ Neurotransmitter: Epinephrine (Epi) and Norepinephrine (NE) ○ Receptors: Typically G-protein coupled receptors Specific receptors: α-adrenergic receptors (α1 and α2) β-adrenergic receptors (β1, β2, β3) 3. Dopaminergic Neurons ○ Neurotransmitter: Dopamine (D1 and D2 receptors) Functional Divisions Central Nervous System (CNS): Includes the brain and spinal cord. Autonomic Nervous System (ANS): Controls involuntary actions. ○ Parasympathetic Division: Uses ACh as neurotransmitter (muscarinic receptors). ○ Sympathetic Division: Uses NE (norepinephrine) and Epi (epinephrine) as neurotransmitters (adrenergic receptors). Somatic Nervous System: ○ Controls voluntary movements via ACh (nicotinic receptors on skeletal muscle). Receptor Specifics Nicotinic ACh Receptors: ○ Found in autonomic ganglia (NN subtype) and neuromuscular junctions (NM subtype). Muscarinic ACh Receptors: ○ Found in various tissues and organs, including cardiac muscle, smooth muscle, and gland cells. The neurons that go from the spinal cord to the parasympathetic ganglia are called preganglionic neurons, whereas the neurons that go from the ganglia to effector organs are called postganglionic neurons. Summary This classification helps in understanding how different neurons and neurotransmitters influence various bodily functions, whether involuntary (autonomic) or voluntary (somatic). The receptors' specific locations and subtypes determine the physiological responses to neurotransmitter release, affecting everything from muscle contraction to glandular secretion and cardiovascular function. Transmitters and Their Specific Sites Junction/Neuron Type Transmitter Released All preganglionic neurons of the parasympathetic and sympathetic Acetylcholine nervous systems All postganglionic neurons of the parasympathetic nervous system Acetylcholine Most postganglionic neurons of the sympathetic nervous system Norepinephrine Postganglionic neurons of the sympathetic nervous system that Acetylcholine innervate sweat glands Adrenal medulla Epinephrine All motor neurons to skeletal muscles Acetylcholine Explanation 1. All preganglionic neurons of the parasympathetic and sympathetic nervous systems release acetylcholine as their transmitter: This means that the first neuron in the autonomic pathway, whether it is parasympathetic or sympathetic, releases acetylcholine at the ganglionic synapse. 2. All postganglionic neurons of the parasympathetic nervous system release acetylcholine as their transmitter: This indicates that the second neuron in the parasympathetic pathway releases acetylcholine to act on the target organs. 3. Most postganglionic neurons of the sympathetic nervous system release norepinephrine as their transmitter: This signifies that the second neuron in the sympathetic pathway typically releases norepinephrine at the target organs. 4. Postganglionic neurons of the sympathetic nervous system that innervate sweat glands release acetylcholine as their transmitter: This is an exception in the sympathetic system where acetylcholine is used instead of norepinephrine. 5. Epinephrine is the principal transmitter released by the adrenal medulla: The adrenal medulla releases epinephrine (and some norepinephrine) directly into the bloodstream, acting as a hormone. 6. All motor neurons to skeletal muscles release acetylcholine as their transmitter: This means that the somatic nervous system, which controls voluntary movements, uses acetylcholine to stimulate skeletal muscle contraction. Slide Pic 2 Cholinergic Receptors Cholinergic receptors are receptors that specifically bind to acetylcholine (ACh). These receptors can be broadly categorized into two types: 1. Nicotinic Cholinergic Receptors: ○ These receptors are ligand-gated ion channels (specifically Na+/K+ channels) that are activated by acetylcholine. ○ Found in both the central nervous system (CNS) and the peripheral nervous system (PNS). ○ Subtypes include NN (found in autonomic ganglia) and NM (found at the neuromuscular junction). 2. Muscarinic Cholinergic Receptors: ○ These receptors are G-protein coupled receptors (GPCRs) that respond to acetylcholine. ○ Found in various tissues and organs, including smooth muscle, cardiac muscle, and gland cells. ○ Subtypes include M1, M2, M3, M4, and M5, each with specific distributions and functions in different tissues. Muscarinic Receptors Muscarinic receptors specifically refer to the subtype of cholinergic receptors that are G-protein coupled receptors (GPCRs) and are activated by acetylcholine. These receptors mediate a variety of physiological responses depending on their subtype and location in the body. The five subtypes of muscarinic receptors (M1 through M5) each have distinct distributions and functions: M1: Found in CNS neurons and peripheral tissues; involved in memory and learning processes. M2: Predominantly found in the heart; involved in regulating cardiac function (slowing heart rate, etc.). M3: Found in smooth muscle and glandular tissue; involved in contraction of smooth muscle and secretion from glands. M4 and M5: Less studied but believed to have roles in CNS functions and possibly in modulating neurotransmitter release. Summary Cholinergic receptors encompass both nicotinic and muscarinic receptors. Nicotinic receptors are ionotropic (ion channel-linked) and are activated by acetylcholine, while muscarinic receptors are metabotropic (GPCRs) and also respond to acetylcholine. Muscarinic receptors specifically refer to the GPCR subtype within the broader category of cholinergic receptors. Each subtype of muscarinic receptor has distinct roles and distributions in the body, influencing various physiological processes depending on their location. Parasympathetic Nervous System Activation of Muscarininc Receptors, Which are located on target organs of the parasympathetic nervous system, elicits: Activation of muscarinic receptors, which are located on target organs of the parasympathetic nervous system, elicits the following responses: 1. Increased glandular secretions: ○ Muscarinic receptors stimulate glands such as salivary glands, lacrimal glands (tear production), and mucous glands in the respiratory tract and digestive system, leading to increased secretion (M3) 2. Contraction of smooth muscle in the bronchi and GI tract: ○ Muscarinic receptors on smooth muscle in the bronchi (airways) and gastrointestinal tract (GI) cause contraction. This can lead to bronchoconstriction in the lungs and increased motility in the digestive system (M3) 3. Slowing of the heart rate (negative chronotropy): ○ Muscarinic receptors on the heart (specifically M2 receptors) mediate a decrease in heart rate by slowing the rate of spontaneous depolarization in the sinoatrial (SA) node (M2) 4. Contraction of the sphincter muscles of the iris, resulting in miosis (reduction in pupillary diameter): ○ Muscarinic receptors in the eye's iris sphincter muscle cause contraction, leading to constriction of the pupil (miosis). 5. Contraction of the ciliary muscles of the eye, causing lens accommodation for near vision (presbyopia): ○ Muscarinic receptors in the ciliary muscle of the eye contract, allowing for adjustment of the lens shape to focus on near objects (accommodation). 6. Dilation of blood vessels and lowering of blood pressure (vasodilation): ○ Activation of muscarinic receptors does not typically lead to vasodilation. Instead, it generally has a minor effect on blood vessels or may cause mild vasodilation through indirect mechanisms. 7. Voiding of the urinary bladder: ○ Muscarinic receptors on the bladder wall (specifically M3 receptors) stimulate contraction of the detrusor muscle and relaxation of the internal urethral sphincter, facilitating bladder emptying (voiding). These responses collectively illustrate the diverse effects of muscarinic receptor activation in the parasympathetic nervous system, influencing various physiological processes including glandular secretions, smooth muscle contraction, cardiac function, and eye function. 3.5 Image of Neuron Different Points in Synapse Where a Drug May Work Here's an outline of the different points in the synaptic process where drugs may act: 1. Synthesis: Drugs can potentially affect the synthesis of neurotransmitters within the presynaptic neuron. For example, drugs that alter the availability of precursor molecules or enzymes involved in neurotransmitter synthesis can influence neurotransmitter levels. 2. Storage: Once synthesized, neurotransmitters are stored in vesicles within the presynaptic terminal. Drugs could interfere with this storage process, affecting the packaging of neurotransmitters into vesicles or the integrity of vesicular membranes. 3. Release: When an action potential reaches the presynaptic terminal, neurotransmitters are released into the synaptic cleft. Drugs can modulate neurotransmitter release by altering the voltage-gated calcium channels responsible for triggering vesicle fusion and exocytosis. 4. Binding: Neurotransmitters released into the synaptic cleft bind to specific receptors on the postsynaptic neuron or other target cells. Drugs can interfere with neurotransmitter binding by acting as agonists (mimicking neurotransmitter action) or antagonists (blocking neurotransmitter binding). 5. Termination: ○ 5a) Reuptake back into axon terminal: After neurotransmitter binding, some neurotransmitters are actively transported back into the presynaptic neuron (reuptake). Drugs can affect this reuptake process, either inhibiting reuptake (leading to increased neurotransmitter availability in the synaptic cleft) or enhancing reuptake (reducing neurotransmitter levels). ○ 5b) Diffusion: Some neurotransmitters may diffuse away from the synaptic cleft and be metabolized or cleared by surrounding tissues. Drugs may affect this diffusion process, though it's less common as a target compared to reuptake or enzymatic breakdown. ○ 5c) Broken down by acetylcholinesterase: Many neurotransmitters, including acetylcholine, are rapidly broken down by specific enzymes like acetylcholinesterase (AChE). Drugs can inhibit these enzymes, leading to increased neurotransmitter levels and prolonged effects of neurotransmitter signaling. Summary Each stage of the synaptic process presents opportunities for pharmacological intervention. Drugs that target neurotransmitter synthesis, storage, release, binding to receptors, or termination mechanisms (reuptake or enzymatic breakdown) can have profound effects on neurotransmission and overall neural function. Understanding these points of intervention is crucial for developing therapeutic strategies to treat neurological and psychiatric disorders effectively. Match Receptor with Drug Class Cholinergic Drugs 1. Muscarinic Agonist ○ Receptor: Muscarinic receptors (M1, M2, M3, etc.) ○ Examples: Pilocarpine, Bethanechol 2. Muscarinic Antagonist (Anticholinergic) ○ Receptor: Muscarinic receptors (M1, M2, M3, etc.) ○ Examples: Atropine, Scopolamine 3. Cholinesterase Inhibitors (acts similar to muscarinic agonist) ○ Mechanism: Inhibit acetylcholinesterase, increasing acetylcholine at synapses ○ Examples: Donepezil, Neostigmine 4. Neuromuscular Blockers (act at NicotinicM skeletal muscle receptors) - Paralyzing agents ○ Receptor: NicotinicM receptors ○ Examples: Succinylcholine, Vecuronium Adrenergic Drugs 1. Alpha and Beta Adrenergic Receptors Agonist ○ Receptor: Alpha (α1, α2) and Beta (β1, β2, β3) receptors ○ Examples: Epinephrine (non-selective), Albuterol (β2 selective), Phenylephrine (α1 selective) 2. Alpha and Beta Adrenergic Antagonist ○ Receptor: Alpha (α1, α2) and Beta (β1, β2, β3) receptors ○ Examples: Propranolol (non-selective beta antagonist), Atenolol (β1 selective antagonist), Prazosin (α1 selective antagonist) These classes outline the primary groups of drugs acting on cholinergic and adrenergic receptors. Good Practice Questions to Ask 1) What type of receptor is this drug working through 2) What is the normal response to activation at the receptor(s) 3) What drug does to receptor function Mechanism Effect on Receptor Activation Agonist drugs Increase receptor activation Antagonist drugs Decrease receptor activation Drugs that bind to receptors and enhance the actions of the Increase receptor natural transmitter at the receptor activation Drugs that block transmitter reuptake Increase receptor activation Drugs that inhibit transmitter degradation Increase receptor activation Explanation Agonist drugs: These drugs mimic the action of the natural neurotransmitter, binding to receptors and activating them, leading to an increase in receptor activation. Antagonist drugs: These drugs bind to receptors but do not activate them. Instead, they block the natural neurotransmitter from binding and activating the receptor, leading to a decrease in receptor activation. Drugs that bind to receptors and enhance the actions of the natural transmitter at the receptor: These drugs increase the effect of the natural neurotransmitter, leading to an increase in receptor activation. Drugs that block transmitter reuptake: By preventing the reuptake of neurotransmitters back into the presynaptic neuron, these drugs increase the amount of neurotransmitter available in the synaptic cleft, leading to increased receptor activation. Drugs that inhibit transmitter degradation: These drugs prevent the breakdown of neurotransmitters, resulting in more neurotransmitter being available to bind to receptors and thus increasing receptor activation. Cholinergic Drugs And Their Receptors Here's a table summarizing cholinergic drugs and their receptors, including receptor subtypes, locations, effects of activation, and examples of agonists, antagonists, and indirect-acting cholinomimetics: Receptor Receptor Effects of Receptor Receptor Indirect-Acting Subtype Location Receptor Agonists Antagonists Cholinomimeti Activation cs Muscarini Sweat glands, ↓ Heart Bethanech Atropine Cholinesterase c Blood vessels, rate, ↑ ol inhibitors: All organs Gland Physostigmine, regulated by secretion, Neostigmine, the Smooth and others parasympatheti muscle c nervous contraction system Nicotinic All ganglia of Promotes Nicotine Mecamylamin Cholinesterase N the autonomic ganglionic e inhibitors: nervous transmissio Physostigmine, system n Neostigmine, and others Nicotinic Neuromuscular Skeletal Nicotine d-Tubocurarin Cholinesterase M junctions muscle e, inhibitors: (NMJs) contraction Succinylcholin Physostigmine, e Neostigmine, and others Explanation 1. Muscarinic Receptors: ○ Location: Found in sweat glands, blood vessels, and all organs regulated by the parasympathetic nervous system. ○ Effects of Activation: Decrease in heart rate, increase in gland secretion, and smooth muscle contraction. ○ Receptor Agonists: Bethanechol (used to treat urinary retention). ○ Receptor Antagonists: Atropine (used to increase heart rate, decrease saliva production, etc.). ○ Indirect-Acting Cholinomimetics: Cholinesterase inhibitors like Physostigmine and Neostigmine increase acetylcholine levels, indirectly activating muscarinic receptors. 2. NicotinicN Receptors: ○ Location: Found in all ganglia of the autonomic nervous system. ○ Effects of Activation: Promote ganglionic transmission. ○ Receptor Agonists: Nicotine (mimics acetylcholine at nicotinicN receptors). ○ Receptor Antagonists: Mecamylamine (used to block ganglionic transmission). ○ Indirect-Acting Cholinomimetics: Cholinesterase inhibitors like Physostigmine and Neostigmine increase acetylcholine levels, indirectly activating nicotinicN receptors. 3. NicotinicM Receptors: ○ Location: Found at neuromuscular junctions (NMJs). ○ Effects of Activation: Cause skeletal muscle contraction. ○ Receptor Agonists: Nicotine (though not commonly used for this purpose). ○ Receptor Antagonists: d-Tubocurarine (non-depolarizing blocker), Succinylcholine (depolarizing blocker, used for muscle relaxation during surgery). ○ Indirect-Acting Cholinomimetics: Cholinesterase inhibitors like Physostigmine and Neostigmine increase acetylcholine levels, indirectly activating nicotinicM receptors. This table provides a comprehensive overview of the types of cholinergic receptors, their locations, the effects of their activation, and the drugs that can act as agonists, antagonists, or indirect activators. Bethanechol Overview Bethanechol Overview Prototype for: Muscarinic Agonists Brand Names: Urecholine, Duvoid Mechanism of Action Direct-acting muscarinic agonist Binds reversibly to muscarinic cholinergic receptors to cause activation Selective for muscarinic receptors; little or no effect on nicotinic receptors Pharmacologic Effects Heart: Causes bradycardia (decreased heart rate) Exocrine glands: Increases sweating, salivation, bronchial secretions, and gastric acid secretion Smooth muscles: ○ Lungs: Constriction of bronchi ○ GI tract: Increased tone and motility ○ Bladder: Contraction of detrusor muscle and relaxation of trigone and sphincter (bladder emptying) ○ Vascular: Relaxation (vasodilation → hypotension) Eyes: ○ Miosis (pupillary constriction) ○ Contraction of ciliary muscle (accommodation for near vision) Pharmacokinetics Administration: Oral Onset of effects: 30-60 minutes Duration: About 1 hour Absorption: Poorly absorbed due to being a quaternary ammonium compound Therapeutic Uses Approved for: ○ Urinary retention (postoperative, postpartum, neurogenic atony of the bladder) Investigational GI Uses: ○ Gastroesophageal reflux (off-label) ○ Disorders associated with GI paralysis (adynamic ileus, gastric atony, postoperative abdominal distention) Contraindications Cardiovascular: Patients with low blood pressure or low cardiac output Gastrointestinal: ○ Gastric ulcers ○ Intestinal obstruction ○ Recent bowel surgery Urinary Tract: ○ Urinary tract obstruction ○ Weakness of the bladder wall Respiratory: Asthma (latent or active) Endocrine: Hyperthyroidism Adverse Effects Cardiovascular: Hypotension, bradycardia Gastrointestinal: ○ Excessive salivation ○ Increased gastric acid secretion ○ Abdominal cramps ○ Diarrhea ○ Involuntary defecation (at higher doses) Urinary Tract: Potential hazard for patients with urinary tract obstruction or bladder wall weakness Respiratory: Bronchoconstriction Others: Dysrhythmias in hyperthyroid patients Preparations, Dosage, and Administration Formulations: Tablets (5, 10, 25, 50 mg) Dosage: 10-50 mg orally, 3-4 times a day Administration advice: 1 hour before meals or 2 hours after to avoid nausea and vomiting Additional Notes Effects of muscarinic activation: Knowing these can help predict the effects of Bethanechol on various organ systems. Precaution: Bedpan or urinal should be readily available when treating patients with urinary retention. This summary organizes the detailed information about Bethanechol into distinct categories for easier understanding and study. Atropine Overview Atropine General Information Names: Atropine [AtroPen, others] Type: Muscarinic antagonist Mechanism of Action Action: Competitive blockade at muscarinic receptors Effect: Prevents receptor activation by endogenous acetylcholine and muscarinic agonists Selectivity: Selective blockade at therapeutic doses, potential nicotinic receptor blockade at high doses Pharmacologic Effects Heart: Increases heart rate Exocrine Glands: Decreases secretion from salivary, bronchial, sweat, and gastric glands Smooth Muscle: ○ Bronchi: Relaxation ○ Bladder: Decreased tone of detrusor ○ GI Tract: Decreased tone and motility Eyes: Mydriasis (pupil dilation), cycloplegia (ciliary muscle relaxation) CNS: Mild excitation at therapeutic doses, hallucinations, and delirium at toxic doses Pharmacokinetics Administration: Topical (eye), IM, IV, subQ Absorption: Rapid Distribution: All tissues, including CNS Elimination: Hepatic metabolism and urinary excretion Half-Life: Approximately 3 hours Therapeutic Uses Preanesthetic Medication: Prevents bradycardia, reduces respiratory secretions Eye Disorders: Mydriasis and ciliary muscle paralysis for examinations and surgery Bradycardia: Accelerates heart rate Intestinal Hypertonicity and Hypermotility: Decreases tone and motility Muscarinic Agonist Poisoning: Antidote for muscarinic poisoning Other Uses: Peptic ulcer disease, asthma, biliary colic (combined with analgesics) Adverse Effects Xerostomia (Dry Mouth): Inhibits salivation Vision: Blurred vision, photophobia, increased intraocular pressure Urinary: Urinary retention GI Tract: Constipation Sweating: Anhidrosis (lack of sweating), risk of hyperthermia Cardiac: Tachycardia Respiratory: Thickened bronchial secretions, risk of bronchial plugging in asthma patients Drug Interactions Interactions: Enhanced effects with antihistamines, phenothiazine antipsychotics, tricyclic antidepressants Dosage and Administration Systemic Therapy: Solution (0.05 to 1 mg/mL) for IM, IV, subQ AtroPen for Cholinesterase Inhibitor Poisoning: Prefilled auto-injector, dosage based on weight and symptom severity Ophthalmic Use: Various formulations for eye conditions 4o ChatGPT can make mistakes. Check Neostigmine Overview Neostigmine Overview Neostigmine, marketed under the name Bloxiverz, is a prototype reversible cholinesterase inhibitor prominently used for managing myasthenia gravis and reversing nondepolarizing neuromuscular blockade post-surgery. Chemistry Neostigmine is characterized by a quaternary nitrogen atom, which means it carries a positive charge. This structure restricts its ability to cross membranes such as the GI tract, blood-brain barrier, and placenta, leading to poor absorption orally and minimal central nervous system effects. Mechanism of Action Neostigmine functions by inhibiting cholinesterase, the enzyme responsible for breaking down acetylcholine. By binding to cholinesterase, neostigmine reduces the enzyme's availability to hydrolyze acetylcholine, thus increasing acetylcholine levels at synapses and enhancing cholinergic transmission. Pharmacologic Effects The primary effect of neostigmine is the amplification of acetylcholine action at muscarinic and nicotinic receptors, which leads to: Muscarinic Responses: Similar to direct-acting muscarinic agonists, causing effects like bradycardia, bronchoconstriction, increased glandular secretions, and enhanced GI motility. Neuromuscular Effects: At therapeutic doses, it increases muscle contraction strength, while toxic doses can lead to reduced muscle force due to constant depolarization and resultant neuromuscular blockade. CNS Effects: Mild stimulation at therapeutic levels and depression at toxic levels, although significant CNS effects are rare due to the drug’s poor penetration of the blood-brain barrier. Pharmacokinetics Neostigmine is administered intramuscularly, intravenously, or subcutaneously due to poor oral absorption. It predominantly acts at the neuromuscular junction and peripheral muscarinic receptors, with a duration of action ranging from 2 to 4 hours. The drug is metabolized enzymatically by cholinesterase. Therapeutic Uses Myasthenia Gravis: Neostigmine enhances communication between nerves and muscles, alleviating symptoms. Reversal of Nondepolarizing Neuromuscular Blockade: Useful postoperatively to counteract drugs like pancuronium. Requires careful monitoring and sometimes concurrent use of atropine to manage muscarinic side effects. Adverse Effects Excessive Muscarinic Stimulation: Including excessive salivation, gastric secretion, GI tract hyperactivity, urinary urgency, bradycardia, sweating, and miosis. Atropine can counteract these effects. Neuromuscular Blockade: High doses can lead to dangerous depolarizing blockade and respiratory paralysis, necessitating emergency interventions. Precautions and Contraindications Neostigmine should be used cautiously or avoided in patients with: GI or urinary tract obstructions Peptic ulcer disease Asthma Coronary insufficiency Hyperthyroidism Those receiving succinylcholine, as neostigmine can exacerbate its effects. Drug Interactions Muscarinic Antagonists: Neostigmine can counteract the effects of atropine, and vice versa. Competitive Neuromuscular Blockers: It can reverse their muscle-relaxing effects. Depolarizing Neuromuscular Blockers: Neostigmine should not be used to reverse their effects as it may intensify neuromuscular blockade. Acute Toxicity Overdose results in a cholinergic crisis characterized by excessive muscarinic stimulation and respiratory depression. Immediate medical intervention is critical. By understanding the detailed mechanism and effects of neostigmine, healthcare providers can effectively leverage its benefits while minimizing potential risks and adverse effects. Physostigmine Overview Physostigmine Overview Physostigmine, unlike neostigmine, readily crosses membranes due to its lack of a quaternary ammonium group, which means it does not carry a charge. This property allows physostigmine to cross the blood-brain barrier and other membranes with ease. Mechanism of Action Similar to neostigmine, physostigmine inhibits cholinesterase, thereby preventing the breakdown of acetylcholine and increasing its levels at cholinergic synapses. This action enhances cholinergic transmission. Pharmacologic Effects Physostigmine’s effects are analogous to those of neostigmine, impacting muscarinic and nicotinic receptors, but with the added ability to affect the central nervous system due to its membrane-crossing capability. Therapeutic Uses Myasthenia Gravis: Like neostigmine, physostigmine can enhance neuromuscular transmission in patients with myasthenia gravis. Antimuscarinic Poisoning: Physostigmine is the preferred treatment for poisoning by atropine and other muscarinic antagonists, including antihistamines and phenothiazine antipsychotics. It is not used for tricyclic antidepressant poisoning due to risks of seizures and cardiotoxicity. Advantages over Neostigmine Physostigmine's ability to cross the blood-brain barrier makes it more effective in reversing central nervous system effects of antimuscarinic poisoning, providing a significant advantage over neostigmine. Edrophonium and Pyridostigmine Overview Edrophonium and pyridostigmine share similar pharmacological effects with neostigmine, with some differences in duration and specific uses. Edrophonium Short Duration: Edrophonium has a very brief duration of action. Diagnostic Use: Primarily used for diagnosing myasthenia gravis, although it is seldom used now due to more accurate testing methods. Pyridostigmine Therapeutic Use: Used similarly to neostigmine for the management of myasthenia gravis and reversal of nondepolarizing neuromuscular blockade. Cholinesterase Inhibitors for Alzheimer’s Disease Three cholinesterase inhibitors—donepezil, galantamine, and rivastigmine—are approved for Alzheimer’s disease management. Mechanism of Action These drugs inhibit cholinesterase in the central nervous system, increasing acetylcholine levels and enhancing cholinergic transmission in the brain, which can help alleviate symptoms of Alzheimer’s disease. Approved Uses Donepezil (Aricept): Used for Alzheimer's disease. Galantamine (Razadyne): Used for Alzheimer's disease. Rivastigmine (Exelon): Used for Alzheimer's disease and dementia associated with Parkinson's disease. Summary of Cholinesterase Inhibitors Neostigmine: Poor membrane crossing, used for myasthenia gravis and reversing neuromuscular blockade. Physostigmine: Crosses membranes, used for myasthenia gravis and antimuscarinic poisoning. Edrophonium: Short-acting, used for diagnosing myasthenia gravis. Pyridostigmine: Used for myasthenia gravis and neuromuscular blockade reversal. Donepezil, Galantamine, Rivastigmine: Used for Alzheimer’s disease (Rivastigmine also for Parkinson’s disease dementia). Categories of Cholinergic Drugs 1) Muscarinic Agonist – Bethanechol (chp 16) 2) Muscarinic Antagonist (atropine) (chp 17) 3) Cholinesterase Inhibitors (pyridostigmine) (chp 18) 4) Neuromuscular Blockers (succinylcholine) chp 19 AcetylCholine (Ach) Acetylcholine (ACh) Overview Where Do We Find Acetylcholine? Acetylcholine is a neurotransmitter found in both the central and peripheral nervous systems. It is predominantly located at: Neuromuscular Junctions: Where it facilitates communication between motor neurons and muscle fibers, leading to muscle contraction. Autonomic Nervous System: In both the sympathetic and parasympathetic branches. In the parasympathetic system, ACh is the primary neurotransmitter at both preganglionic and postganglionic synapses. In the sympathetic system, it is found at preganglionic synapses. Central Nervous System: In various brain regions, including the cerebral cortex and hippocampus, where it plays a role in attention, memory, and arousal. What Does Acetylcholine Do? Acetylcholine is involved in several critical physiological functions: Muscle Contraction: At neuromuscular junctions, ACh binds to receptors on muscle cells, causing muscle contraction. Autonomic Functions: In the parasympathetic nervous system, ACh promotes rest-and-digest activities, such as slowing the heart rate, increasing glandular secretions, and stimulating digestive processes. Cognitive Functions: In the brain, ACh is involved in promoting wakefulness, learning, and memory. It helps modulate synaptic plasticity and cognitive functions. Why Can't We Just Take ACh as a Drug? Administering acetylcholine directly as a drug is impractical for several reasons: 1. Rapid Breakdown: Acetylcholine is quickly degraded by the enzyme acetylcholinesterase (AChE) present in synapses and the bloodstream. This rapid breakdown prevents ACh from maintaining therapeutic levels in the body. 2. Poor Absorption: Acetylcholine is a charged molecule, which makes it poorly absorbed from the gastrointestinal tract and unable to cross the blood-brain barrier effectively. 3. Non-Specific Effects: ACh acts on both muscarinic and nicotinic receptors throughout the body, leading to widespread and potentially dangerous effects, such as severe bradycardia (slow heart rate), hypotension (low blood pressure), and excessive glandular secretions. Alternatives to Direct ACh Administration To circumvent the issues associated with direct ACh administration, various alternative approaches are used: Cholinesterase Inhibitors: Drugs like neostigmine, physostigmine, and donepezil inhibit acetylcholinesterase, the enzyme that breaks down ACh, thereby increasing the levels and duration of action of ACh at synapses. Direct-Acting Cholinergic Agonists: These drugs, such as bethanechol and pilocarpine, mimic the action of ACh by directly stimulating muscarinic receptors. Indirect-Acting Cholinergic Agents: These include drugs that either enhance the release of ACh or inhibit its reuptake. By using these approaches, it is possible to enhance cholinergic transmission in a controlled manner, targeting specific receptor subtypes or increasing ACh levels where needed, while avoiding the rapid breakdown and non-specific effects associated with direct ACh administration. Myasthenia Gravis Myasthenia Gravis: Pathophysiology Myasthenia gravis (MG) is an autoimmune disorder characterized by: Muscle Weakness: Fluctuating weakness of skeletal muscles, worsened with activity and improved with rest. Symptoms: Common manifestations include ptosis (drooping eyelids), difficulty swallowing (dysphagia), and weakness in limbs and respiratory muscles. Autoimmune Process: The immune system produces antibodies that attack nicotinic receptors on skeletal muscle cells, reducing the number of functional receptors at the neuromuscular junction (NMJ) by 70% to 90%. This reduces the ability of nerves to stimulate muscle contraction, leading to weakness and fatigue. Treatment with Cholinesterase Inhibitors Mechanism: Reversible cholinesterase inhibitors (e.g., neostigmine) are the primary treatment. They work by inhibiting acetylcholinesterase, the enzyme that breaks down acetylcholine (ACh) at the NMJ. By preventing its breakdown, these drugs increase the concentration of ACh available to stimulate muscle contraction, thereby improving muscle strength. Symptomatic Relief: Cholinesterase inhibitors do not cure MG but provide symptomatic relief. Patients typically require lifelong therapy to manage symptoms effectively. Management Considerations Assessment: Before administering oral medications to hospitalized MG patients, assess their ability to swallow with a small sip of water. If swallowing is impaired, parenteral medication should be considered. Side Effects: Cholinesterase inhibitors can lead to excessive muscarinic stimulation due to increased ACh levels at muscarinic receptors throughout the body. Atropine may be used to counteract these effects when necessary, although routine use is discouraged due to its potential to mask early signs of cholinesterase inhibitor overdose. Dosage Adjustment: Finding the optimal dosage is critical and often requires titration based on individual response. Monitoring fatigue patterns, muscle strength, and signs of muscarinic stimulation helps in determining the correct dose. Patient Education: Patients should be educated to recognize signs of undermedication (e.g., worsening weakness) and overmedication (e.g., excessive salivation). They may need to adjust their medication dose in anticipation of activities that require increased muscle function. Myasthenic Crisis and Cholinergic Crisis Myasthenic Crisis: Occurs due to inadequate medication leading to severe muscle weakness, especially in respiratory muscles. Treatment involves administering a cholinesterase inhibitor (e.g., neostigmine) to increase ACh levels at NMJs and alleviate symptoms. Cholinergic Crisis: Results from cholinesterase inhibitor overdose, causing severe muscle weakness and excessive muscarinic stimulation. Treatment includes respiratory support and atropine administration to counteract muscarinic effects. The offending cholinesterase inhibitor should be withheld until symptoms resolve. Differentiation: It's crucial to distinguish between myasthenic crisis and cholinergic crisis because their treatments differ significantly. History, clinical signs, and sometimes a challenge with edrophonium (a short-acting cholinesterase inhibitor) can aid in making the diagnosis. Medical Alert Identification Importance: Due to the potential for life-threatening crises, patients with MG should wear a medical alert bracelet or carry identification to inform healthcare providers of their condition. This ensures appropriate and timely management during emergencies. In summary, myasthenia gravis is characterized by autoimmune-mediated muscle weakness, primarily managed with cholinesterase inhibitors to enhance neuromuscular transmission. Effective treatment requires careful dosage adjustment, patient education, and vigilance for both myasthenic and cholinergic crises, emphasizing the need for proactive medical identification. Normal Effects of Ach In The Body Parasympathetic Effects of Acetylcholine (Muscarinic Receptor Activation) 1. Cardiovascular System: ○ Vasodilation: ACh causes vasodilation in some vascular beds, particularly in the skin and skeletal muscles, leading to increased blood flow to these areas. ○ Decreased Heart Rate (Negative chronotropic effect): ACh acts on muscarinic receptors in the sinoatrial (SA) node of the heart, reducing the firing rate of the SA node and thus decreasing heart rate. ○ Decreased Blood Pressure: Due to vasodilation and reduced heart rate, ACh can lead to a decrease in blood pressure. 2. Respiratory System: ○ Bronchoconstriction: ACh causes constriction of bronchial smooth muscle, leading to narrowing of the airways. 3. Urinary System: ○ Bladder Contraction: ACh stimulates muscarinic receptors in the bladder wall, causing detrusor muscle contraction. This promotes bladder emptying (voiding). 4. Gastrointestinal System: ○ Increased Motility: ACh increases the tone and motility of smooth muscle in the gastrointestinal (GI) tract, enhancing peristalsis and facilitating movement of food through the digestive system. ○ Increased Secretions: ACh stimulates secretory glands in the GI tract (e.g., salivary glands, gastric glands), increasing the production of saliva, gastric acid, and other digestive enzymes. 5. Other Effects: ○ Lacrimal Secretion: ACh stimulates muscarinic receptors on lacrimal glands, increasing the production of tears (lacrima secretion). Muscarinic Agonist (AKA Cholinergic agonist) This drug is trying to mimic acetylcholine. This is called the prototype drug for this class. Very similar to structure & function to Ach Use: Recovery from surgery, being under general anesthesia, bladder paralysis, bethanechol can restimulate GI (paralytic ileus) and bladder activity. - Expect to see urine by about 6 hours post-op, BM can take 2-4 days. Side Effects of Bethanechol Bethanechol is a muscarinic receptor agonist used therapeutically to stimulate smooth muscle, particularly in the urinary bladder, to promote voiding. Here are the details regarding its receptor, therapeutic effects, and possible side effects: Receptor: Bethanechol primarily acts as a direct agonist at muscarinic receptors, specifically the M2 and M3 subtypes. These receptors are located in various tissues throughout the body, including smooth muscle in the bladder, gastrointestinal tract, and blood vessels. Therapeutic Effect: The primary therapeutic effect of bethanechol is to stimulate muscarinic receptors in the bladder, leading to detrusor muscle contraction. This action helps in the treatment of urinary retention by promoting bladder emptying. Possible Side Effects: Since bethanechol mimics the effects of acetylcholine on muscarinic receptors, it can induce a range of cholinergic side effects, primarily reflecting parasympathetic (rest and digest) nervous system activation: 1. Cardiovascular System: ○ Bradycardia (Low Heart Rate): Bethanechol can stimulate M2 receptors in the heart, leading to a decrease in heart rate. 2. Gastrointestinal System: ○ Increased Salivation: Stimulation of muscarinic receptors in salivary glands can increase saliva production. ○ Increased GI Motility: Bethanechol enhances peristalsis and can lead to increased motility of the GI tract, which may result in cramping and diarrhea. 3. Urinary System: ○ Increased Urination: By stimulating M3 receptors in the bladder wall, bethanechol promotes detrusor muscle contraction, facilitating urine voiding. 4. Dermatological System: ○ Flushing and Diaphoresis (Sweating): Bethanechol can cause vasodilation and sweating through activation of muscarinic receptors in the skin. 5. Respiratory System: ○ Bronchoconstriction: Stimulation of bronchial smooth muscle M3 receptors can lead to narrowing of the airways, which can exacerbate asthma symptoms in susceptible individuals. Clinical Consideration: Due to its potential to induce bronchoconstriction, bethanechol should be used cautiously in patients with severe asthma or chronic obstructive pulmonary disease (COPD). These patients are at risk of exacerbating their respiratory symptoms if exposed to agents that increase bronchoconstriction, such as muscarinic agonists like bethanechol. Pilocarpine The medication described sounds like pilocarpine, which is a muscarinic receptor agonist used primarily to decrease intraocular pressure in conditions such as glaucoma. Here are the details regarding its mechanism of action, selectivity, side effects, and pharmacokinetics: Mechanism of Action: Pilocarpine acts as a direct agonist at muscarinic receptors, particularly the M3 subtype, located in the eye. By stimulating these receptors, pilocarpine causes contraction of the ciliary muscles and the iris sphincter muscle. Contraction of Ciliary Muscles: This contraction allows for the drainage of aqueous humor from the eye, thereby reducing intraocular pressure. Pupil Constriction (Miosis): Activation of muscarinic receptors in the iris sphincter muscle leads to pupil constriction (miosis), which can affect vision by reducing the amount of light entering the eye. Selectivity: Compared to non-selective muscarinic agonists like bethanechol, pilocarpine is more selective for certain muscarinic receptor subtypes, particularly those found in the eye. This selectivity helps to minimize systemic side effects while targeting its therapeutic effects locally in the eye. Side Effects: Given its mechanism of action and systemic absorption, pilocarpine can induce various cholinergic side effects, which typically include: Increased Salivation: Pilocarpine stimulates muscarinic receptors in salivary glands, leading to increased saliva production (ptyalism). Increased Sweating: Activation of muscarinic receptors in sweat glands can result in increased sweating (diaphoresis). Vision Effects: Pupil constriction (miosis) can limit vision, particularly in dim lighting conditions. Patients may be advised to avoid driving at night or in low light situations due to reduced pupil size. Pharmacokinetics: Metabolism: Pilocarpine is metabolized primarily in the liver by various enzymatic pathways. Excretion: After metabolism, pilocarpine and its metabolites are excreted mainly through the kidneys via urine, and to some extent through bile. Clinical Considerations: Fluid Intake: Due to increased salivation and sweating, patients taking pilocarpine should be encouraged to maintain adequate fluid intake to prevent dehydration. Sunlight and Night Driving: Pupil constriction induced by pilocarpine can lead to sensitivity to bright light and difficulty with night vision. Patients should be advised accordingly to avoid prolonged exposure to sunlight and consider the implications for night driving. In summary, pilocarpine is a selective muscarinic agonist used in the treatment of glaucoma by reducing intraocular pressure. While effective locally in the eye, it can cause systemic cholinergic side effects that necessitate patient education and monitoring. Careful management of its use, particularly in relation to fluid intake and environmental conditions affecting vision, is essential to optimize therapeutic outcomes and minimize adverse effects. ABCDs of Anticholinergic Effects The "ABCDs of Anticholinergic Effects" refer to common side effects associated with drugs that exert anticholinergic activity, which blocks the action of acetylcholine at muscarinic receptors. Here's a breakdown of each letter: A for Anorexia: Anticholinergic drugs can lead to decreased appetite or anorexia. This occurs because the blockade of muscarinic receptors inhibits gastrointestinal motility and reduces the secretion of digestive enzymes and gastric acid, which can contribute to feelings of fullness or decreased appetite. B for Blurry Vision (D for Dilation): Anticholinergic drugs cause pupil dilation (mydriasis) by blocking muscarinic receptors in the iris sphincter muscle. This dilation results in increased light entering the eye, which can cause blurry vision, particularly at near distances. C for Constipation: Anticholinergic drugs decrease gastrointestinal motility by inhibiting muscarinic receptors in the gastrointestinal tract. This reduction in motility can lead to constipation, as food moves more slowly through the digestive system. D for Dry Mouth: Anticholinergic drugs reduce salivary gland secretion by blocking muscarinic receptors on these glands. This reduction in saliva production results in dry mouth, which can lead to discomfort, difficulty swallowing, and an increased risk of dental caries due to reduced saliva's protective functions. These effects are collectively referred to as the "ABCDs" because they encompass common symptoms associated with the anticholinergic activity of medications. It's important for healthcare providers to be aware of these potential side effects when prescribing or administering anticholinergic drugs, as they can impact patient comfort and adherence to treatment. At Least 600 meds are known to have anticholinergic Effects The mnemonic "Mad as a hatter, Blind as a bat, Dry as a bone, Hot as a hare, Bloated as a toad, The heart runs alone, Full as a flask, Red as a beet" is used to remember the diverse range of symptoms associated with anticholinergic effects. Here's an explanation of each: 1. Mad as a hatter (delirium): Anticholinergic drugs can cause confusion, memory disturbances, hallucinations, and delirium, which are often likened to the mental state historically associated with mercury poisoning (hatters used to use mercury in hat-making). 2. Blind as a bat (ocular symptoms): Anticholinergic drugs can lead to pupil dilation (mydriasis), blurry vision, and difficulty focusing, hence the comparison to the impaired vision of a bat. 3. Dry as a bone (dry mouth, dry skin): Anticholinergic drugs reduce saliva production (dry mouth) and can decrease sweat gland activity (dry skin), leading to overall dryness. 4. Hot as a hare (fever): Anticholinergic drugs can interfere with the body's ability to regulate temperature, leading to an increased risk of hyperthermia. 5. Bloated as a toad (constipation): Anticholinergic drugs slow down gastrointestinal motility, which can result in constipation and bloating. 6. The heart runs alone (tachycardia): Anticholinergic drugs can cause an increase in heart rate (tachycardia) due to their effect on cardiac muscarinic receptors. 7. Full as a flask (urinary retention): Anticholinergic drugs can relax the bladder sphincter and decrease bladder muscle contraction, leading to difficulty urinating and urinary retention. 8. Red as a beet (cutaneous vasodilation)/Flushing to face: Anticholinergic drugs can cause vasodilation in the skin, particularly in the face, resulting in flushing and redness. Clinically, the most concerning feature of anticholinergic effects, especially in the elderly, is delirium. Elderly patients are particularly susceptible due to age-related changes in drug metabolism, increased sensitivity to medications, and the cumulative effects of multiple medications with anticholinergic properties. Delirium can significantly impair cognitive function, increase the risk of falls, and lead to longer hospital stays. Healthcare providers must be cautious when prescribing medications with anticholinergic effects, especially in older adults, and consider alternative treatments or dosage adjustments to minimize these adverse effects while still achieving therapeutic goals. Atropine The prototype for a muscarinic antagonist, which blocks acetylcholine from binding to muscarinic receptors, is atropine. Receptor: Atropine acts by competitively blocking muscarinic acetylcholine receptors, thereby preventing acetylcholine from exerting its effects at these sites. Effect: Atropine's blockade of muscarinic receptors leads to a range of physiological responses that mimic sympathetic activation: Increase in heart rate (HR): Atropine causes tachycardia by blocking the parasympathetic influence on the heart, specifically the vagal (cholinergic) tone that normally slows the heart rate. This effect makes atropine particularly useful in the treatment of symptomatic bradycardia (heart rate less than 60 beats per minute with associated symptoms like fatigue, dizziness, or hypotension). Other effects of atropine include: Bronchodilation: Atropine relaxes bronchial smooth muscle, making it useful in treating bronchoconstriction in conditions like asthma or during anesthesia. Decreased GI motility and secretions: Atropine reduces gastric acid secretion and inhibits gastrointestinal motility, which can help in certain gastrointestinal disorders. Pupil dilation (mydriasis): Atropine causes pupil dilation by blocking muscarinic receptors in the iris sphincter muscle. This effect is used in ophthalmology for eye examinations and surgeries. Decreased salivation: Atropine reduces salivary and bronchial secretions, which can be beneficial in anesthesia to prevent airway obstruction and during surgery. Clinical Use: Atropine is employed in various clinical scenarios: Bradycardia: It is used to increase heart rate in symptomatic bradycardia when other measures are inadequate or when rapid intervention is needed. Antidote for muscarinic poisoning: Atropine is used to treat poisoning or overdose with muscarinic agonists (e.g., organophosphate insecticides or nerve agents) by blocking excessive muscarinic receptor stimulation. Ophthalmic procedures: Atropine is used in eye drops to dilate the pupil and temporarily paralyze accommodation (cycloplegia) for eye examinations and procedures. Atropine is a crucial medication in emergency medicine, anesthesia, and ophthalmology due to its rapid onset of action and potent effects on the autonomic nervous system. However, its use requires careful consideration of its potential side effects and contraindications, particularly in patients with certain cardiovascular conditions or glaucoma. Atropine Side effects: Atropine, as a muscarinic antagonist, exerts its effects by blocking the action of acetylcholine at muscarinic receptors throughout the body. This blockade leads to a variety of physiological changes that are generally opposite to the effects of parasympathetic nervous system activation. Regarding urination: Effect on the detrusor muscle: Atropine causes relaxation of the detrusor muscle of the bladder. Normally, the parasympathetic nervous system stimulates the detrusor muscle to contract, which facilitates bladder emptying (urination). By blocking muscarinic receptors on the detrusor muscle, atropine inhibits this contraction. Resulting effect on urination: With the detrusor muscle relaxed due to atropine, the bladder's ability to contract and expel urine is impaired. This can lead to urinary retention, where the bladder does not empty fully or efficiently. Consequently, less urination occurs. Therefore, one of the side effects of atropine is urinary retention, which can be problematic in certain clinical situations, especially in patients with pre-existing urinary issues or conditions that affect bladder function. It's important to note that while atropine's main therapeutic effects (e.g., increasing heart rate, dilating pupils) can be beneficial in specific medical contexts, its broader systemic effects on the autonomic nervous system necessitate careful consideration and monitoring to avoid adverse outcomes such as urinary retention. Scopolamine Patch The scopolamine patch is indeed a muscarinic antagonist primarily used to prevent motion sickness. Here are some key points about its use and administration: 1. Mechanism of Action: Scopolamine, when delivered via a transdermal patch, acts as a muscarinic antagonist. It binds to and blocks muscarinic receptors in the inner ear and other parts of the brain, which helps reduce motion-induced nausea and vomiting. 2. Administration: ○ Placement: The patch is typically applied behind the ear on a clean, hairless area of the skin. This location allows for absorption through the skin and into the bloodstream. ○ Timing: It's recommended to apply the patch approximately 4 hours before the anticipated onset of motion sickness. This allows sufficient time for the medication to be absorbed into the body and become effective. 3. Duration of Effect: The transdermal delivery system of the patch results in a slow onset of action but provides a longer-lasting effect compared to oral medications. This makes it convenient for preventing motion sickness over an extended period, such as during long journeys. 4. Safety Considerations: ○ Disposal: Used patches should be disposed of safely away from children and pets due to the potential residual medication. ○ Patch Replacement: When applying a new patch, it's important to remove the old one first to prevent accumulation of the drug. ○ Hand Hygiene: Always wash hands thoroughly with soap and water after handling the patch to avoid accidental exposure to scopolamine. 5. Side Effects: The transdermal patch delivery method is generally associated with fewer systemic side effects compared to oral administration. However, common side effects can include dry mouth, dizziness, drowsiness, and blurred vision. 6. Special Considerations: ○ Patients should be advised not to cut the patch or apply it to damaged or irritated skin. ○ It's important to follow the specific instructions provided with the patch regarding application, duration of use, and disposal. Overall, the scopolamine patch is an effective option for managing motion sickness, offering a controlled and sustained release of medication with minimized systemic side effects when compared to other routes of administration. Anticholinergic Respiratory Medication Ipratropium (Atrovent) and Tiotropium (Spiriva) are both anticholinergic medications used as bronchodilators, particularly in the treatment of chronic obstructive pulmonary disease (COPD) and sometimes asthma. Here's a detailed comparison: Ipratropium (Atrovent): Mechanism of Action: Acts as a muscarinic antagonist by blocking muscarinic receptors in the airways. Administration: Administered via inhalation. Typically requires multiple inhalations (2 inhalations, 4 times daily) for optimal effect. Onset of Action: Rapid onset, with effects seen within seconds to minutes after inhalation (usually within 30 seconds). Duration of Action: Relatively short duration of action, requiring frequent dosing throughout the day. Common Uses: Used for acute relief of bronchospasm in COPD and sometimes in asthma exacerbations. Systemic Effects: Minimal systemic absorption from the lungs due to its inhalational route, reducing the likelihood of systemic side effects. Tiotropium (Spiriva): Mechanism of Action: Also acts as a muscarinic antagonist, similar to ipratropium. Administration: Administered via inhalation, but differs in frequency from ipratropium. Tiotropium is typically administered once daily. Onset of Action: Slower onset compared to ipratropium, with effects usually noticeable within 30 minutes after inhalation. Duration of Action: Longer duration of action compared to ipratropium, providing sustained bronchodilation over 24 hours with once-daily dosing. Common Uses: Used for maintenance treatment in COPD to prevent bronchospasm and improve lung function over the long term. Systemic Effects: Like ipratropium, systemic absorption is minimal due to its inhalational route, which reduces the risk of systemic side effects. Comparison: Speed of Action: Ipratropium acts quickly to relieve acute bronchospasm, making it suitable for immediate relief. Tiotropium, while slower to act, provides sustained bronchodilation over a longer period, making it ideal for maintenance therapy. Frequency: Ipratropium requires more frequent dosing throughout the day (4 times daily) compared to tiotropium (once daily). Side Effects: Both medications generally cause minimal systemic side effects. Dry mouth is the most common side effect due to their anticholinergic action, but this is less pronounced with inhalational administration compared to oral medications. In summary, both ipratropium and tiotropium are effective bronchodilators used in the management of COPD and sometimes asthma. They differ in onset and duration of action, as well as dosing frequency, which allows for tailored treatment approaches based on the patient's needs and the severity of their condition. Overactive Bladder? Overactive bladder (OAB) is a condition characterized by the involuntary contraction of the detrusor muscle in the bladder, which leads to symptoms such as frequent urination, sudden urges to urinate (urgency), and sometimes urinary incontinence. Here's a detailed overview of oxybutynin, a medication commonly used to treat OAB: Oxybutynin: Mechanism of Action: Oxybutynin is a muscarinic antagonist that works by blocking muscarinic receptors on the detrusor muscle of the bladder. By inhibiting these receptors, oxybutynin reduces involuntary contractions of the bladder muscle, thereby decreasing urgency and urinary frequency associated with OAB. Administration: Oxybutynin is available in various formulations, including immediate-release tablets, extended-release tablets, transdermal patches, and topical gels. The choice of formulation depends on factors such as patient preference, tolerability, and dosing frequency. Pharmacokinetics: ○ Half-life: Oxybutynin has a relatively short half-life of approximately 2-3 hours. This short half-life necessitates frequent dosing to maintain therapeutic blood levels. ○ Blood-Brain Barrier (BBB): Oxybutynin can cross the blood-brain barrier, which increases the risk of central nervous system (CNS) side effects. Common CNS side effects include confusion, hallucinations, dizziness, and drowsiness. These effects are more pronounced in elderly patients due to age-related changes in drug metabolism and increased sensitivity to anticholinergic medications. Indications: Oxybutynin is primarily used to treat symptoms of overactive bladder, including urinary urgency, frequency, and urge incontinence. It helps improve bladder control by reducing the frequency and intensity of involuntary bladder contractions. Side Effects: ○ Anticholinergic Effects: Dry mouth is a frequent side effect of oxybutynin due to its blockade of muscarinic receptors. Other common anticholinergic side effects may include constipation, blurred vision, urinary retention, and decreased sweating. ○ CNS Effects: As mentioned, CNS side effects such as confusion, hallucinations, and dizziness are more prevalent with oxybutynin, particularly at higher doses or in susceptible individuals. Special Considerations: ○ Elderly Population: Caution is advised when prescribing oxybutynin to elderly patients due to their increased risk of experiencing anticholinergic side effects and CNS effects. Lower initial doses and slower titration may be necessary to minimize adverse effects while achieving therapeutic benefits. ○ Dosage Adjustment: Individualized dosing and regular monitoring are essential to optimize treatment efficacy and minimize side effects in all patient populations. In summary, oxybutynin is an effective medication for managing overactive bladder by reducing the frequency and intensity of bladder muscle contractions. However, its use requires careful consideration of potential anticholinergic and CNS side effects, especially in elderly patients. Healthcare providers should tailor the treatment regimen based on patient response and tolerability to achieve optimal outcomes in the management of overactive bladder. Cholinesterase Inhibitors Acetylcholinesterase (Cholinesterase) and Its Inhibitors Acetylcholinesterase (AChE): Definition: Acetylcholinesterase, often referred to simply as cholinesterase, is an enzyme responsible for breaking down the neurotransmitter acetylcholine (ACh) in the synaptic cleft. Role in Homeostasis: ○ Digestion of ACh: AChE hydrolyzes acetylcholine into acetic acid and choline, terminating its action at the synaptic cleft. ○ Prevention of Overstimulation: By breaking down acetylcholine, AChE helps prevent the overstimulation of cholinergic receptors, maintaining proper neural function and homeostasis. Cholinesterase Inhibitors: Mechanism of Action: ○ Blocking AChE: Cholinesterase inhibitors prevent AChE from breaking down acetylcholine, resulting in increased levels of ACh at the synaptic cleft. ○ Enhanced Transmission: The elevated acetylcholine levels enhance cholinergic transmission, which can help alleviate symptoms of conditions like Alzheimer’s disease by improving communication between neurons. Therapeutic Use: ○ Alzheimer’s Disease: Cholinesterase inhibitors, such as donepezil, rivastigmine, and galantamine, are commonly used to manage symptoms of Alzheimer’s disease. ○ Myasthenia Gravis: These inhibitors can also be used to improve muscle strength in patients with myasthenia gravis by enhancing neuromuscular transmission. ○ Other Uses: They may be used in the management of other conditions involving cholinergic deficits or disorders. Effects of Increased Acetylcholine: Cognitive Improvement: Enhanced levels of acetylcholine in the brain can lead to modest improvements in cognition, behavior, and function in Alzheimer’s patients. Muscle Contraction: Increased acetylcholine can improve muscle contraction in conditions like myasthenia gravis. Adverse Effects of Cholinesterase Inhibitors: Cholinergic Side Effects: ○ Gastrointestinal: Nausea, vomiting, diarrhea, and dyspepsia are common due to increased cholinergic activity in the GI tract. ○ Neurological: Dizziness and headache. ○ Respiratory: Bronchoconstriction, especially in patients with asthma or COPD. ○ Cardiovascular: Bradycardia, which can lead to fainting, falls, and fall-related fractures. Special Considerations: ○ Drug Interactions: Avoid concomitant use with drugs that block cholinergic receptors, such as anticholinergic agents, first-generation antihistamines, tricyclic antidepressants, and conventional antipsychotics, as these can reduce the efficacy of cholinesterase inhibitors. ○ Caution in Certain Populations: Use cautiously in patients with preexisting conditions that could be exacerbated by increased cholinergic activity. Patient Teaching Points: Side Effect Management: Educate patients about the potential side effects and the importance of reporting severe or persistent symptoms. Dosage and Administration: Ensure patients understand the importance of adhering to the prescribed dosage and not abruptly discontinuing the medication without consulting their healthcare provider. Monitoring: Regular follow-ups to monitor effectiveness and side effects are crucial, as well as periodic liver function tests if the medication is metabolized in the liver. By blocking the action of acetylcholinesterase, cholinesterase inhibitors increase the concentration of acetylcholine in the synaptic cleft, enhancing cholinergic transmission and providing therapeutic benefits in conditions like Alzheimer's disease and myasthenia gravis. However, the increased cholinergic activity can also lead to various side effects, necessitating careful monitoring and patient education. Pyridostigmine Cholinesterase inhibitors are medications used to treat conditions where increasing acetylcholine (ACh) levels at the neuromuscular junction (NMJ) is beneficial. Here's a detailed overview: Mechanism of Action: Increased ACh Effect: Cholinesterase inhibitors work by inhibiting the enzyme acetylcholinesterase, which normally breaks down acetylcholine. By inhibiting this enzyme, more acetylcholine is available at the NMJ. This increased acetylcholine enhances neuromuscular transmission, leading to improved muscle strength and function, particularly in conditions like myasthenia gravis. Indications: Myasthenia Gravis: This autoimmune disorder is characterized by antibodies attacking nicotinic M receptors on skeletal muscles, which results in muscle weakness and fatigue. Cholinesterase inhibitors like neostigmine and pyridostigmine help alleviate symptoms by prolonging the action of acetylcholine at the NMJ, thereby enhancing muscle contractions. Side Effects: Gastrointestinal Effects: Common side effects of cholinesterase inhibitors include diarrhea and cramping. This occurs because increased acetylcholine in the gut can stimulate excessive contractions of smooth muscle, leading to gastrointestinal disturbances. Muscle Twitching: Overactive stimulation of muscles due to increased acetylcholine levels at the NMJ can cause muscle twitching or fasciculations. This twitching is a result of excessive nerve impulses leading to involuntary muscle contractions. Considerations: Dosage Adjustment: Finding the optimal dosage of cholinesterase inhibitors is crucial to balancing therapeutic benefits with manageable side effects. Physicians typically start with low doses and gradually increase based on patient response and tolerance. Monitoring: Patients on cholinesterase inhibitors may require regular monitoring for both therapeutic response and adverse effects. Monitoring includes assessing muscle strength, gastrointestinal symptoms, and any signs of overstimulation such as muscle twitching. Summary: Cholinesterase inhibitors play a critical role in managing myasthenia gravis by enhancing neuromuscular transmission through increased acetylcholine levels at the NMJ. While effective, they can cause gastrointestinal disturbances such as diarrhea and cramping, as well as muscle twitching due to excessive muscle stimulation. Careful management and monitoring are essential to optimize treatment outcomes while minimizing side effects in patients receiving cholinesterase inhibitors for myasthenia gravis and related conditions. Diagram: 1. Action Potential Generation: ○ An action potential (electrical signal) travels down the motor neuron to the neuromuscular junction. 2. Release of Acetylcholine (ACh): ○ Arrival of the action potential at the presynaptic terminal (motor neuron terminal) triggers the opening of voltage-gated calcium channels. ○ Calcium ions (Ca++) influx into the presynaptic terminal causes synaptic vesicles containing acetylcholine to fuse with the presynaptic membrane. ○ Acetylcholine is released into the synaptic cleft (subneural space) between the presynaptic terminal and the motor end-plate (postsynaptic membrane of the muscle fiber). 3. Binding of ACh to Nicotinic Receptors: ○ Acetylcholine diffuses across the synaptic cleft and binds to nicotinicM receptors (nicotinic acetylcholine receptors) on the motor end-plate of the skeletal muscle fiber. ○ These receptors are ligand-gated ion channels that open upon ACh binding. 4. Depolarization of the Motor End-plate: ○ Activation of nicotinicM receptors leads to the influx of sodium ions (Na+) into the muscle fiber, while potassium ions (K+) exit the cell. ○ This influx of positive ions results in depolarization of the motor end-plate, generating an end-plate potential. 5. Propagation of Muscle Action Potential: ○ The end-plate potential triggers an action potential that spreads along the sarcolemma (muscle cell membrane) and into the T-tubules (invaginations of the sarcolemma). 6. Release of Calcium Ions (Ca++) from the Sarcoplasmic Reticulum: ○ The action potential in the T-tubules causes voltage-sensitive proteins (dihydropyridine receptors) to change shape, thereby activating calcium-release channels (ryanodine receptors) in the sarcoplasmic reticulum (SR). ○ Calcium ions are released from the SR into the sarcoplasm (cytoplasm of the muscle fiber). 7. Interaction of Calcium with Actin and Myosin: ○ Calcium ions bind to troponin C on the actin filaments of the sarcomere. ○ This binding causes a conformational change in the troponin-tropomyosin complex, exposing the myosin-binding sites on actin. 8. Contraction of the Muscle Fiber: ○ Myosin heads bind to the exposed binding sites on actin, forming cross-bridges. ○ ATP hydrolysis and release of energy allow myosin heads to pivot, pulling the actin filaments toward the center of the sarcomere (power stroke). ○ This process continues, causing sarcomere shortening and muscle fiber contraction. Somatic Nervous System 1. Neuromuscular Junction (NMJ): ○ The NMJ is the specialized synapse between a motor neuron and a skeletal muscle fiber. ○ It's where the motor neuron terminal releases acetylcholine (ACh) to communicate with the muscle fiber. 2. Release of Acetylcholine: ○ When an electrical impulse (action potential) reaches the motor neuron terminal, it triggers the release of synaptic vesicles containing ACh into the synaptic cleft (synapse) of the NMJ. 3. Nicotinic Receptors (Ionotropic Ach Receptors): ○ Nicotinic receptors on the motor end-plate of the skeletal muscle fiber are ligand-gated ion channels. ○ They are called ionotropic because they directly gate ion flow across the cell membrane in response to ACh binding. ○ Specifically, they open to allow the influx of sodium ions (Na+) and the efflux of potassium ions (K+), leading to depolarization of the muscle fiber membrane. 4. Contraction Mechanism: ○ ACh binding to nicotinic receptors at the NMJ initiates muscle contraction. ○ The depolarization of the muscle fiber membrane (end-plate potential) spreads across the sarcolemma and into the T-tubules, triggering the release of calcium ions (Ca2+) from the sarcoplasmic reticulum. ○ Calcium ions then bind to troponin C on actin filaments, initiating the sliding of actin and myosin filaments and ultimately causing muscle contraction. 5. Ionotropic Effect on Contractility: ○ The term "ionotropic" refers to receptors that directly control ion flow across the cell membrane. ○ In the context of cardiac muscle (as you mentioned with positive inotropic effect), ionotropic effects can influence the strength of muscle contraction. ○ In skeletal muscle, the ionotropic nicotinic receptors contribute to the rapid onset of muscle contraction upon ACh binding. Understanding these mechanisms is crucial for grasping how neuromuscular signaling leads to muscle contraction and how different drugs or conditions can affect these processes, influencing muscle function. At Cellular Level Nicotinic Receptors: 1. Type: Ionotropic receptors. 2. Structure: ○ Nicotinic receptors are pentameric ligand-gated ion channels. This means they are composed of five subunits arranged around a central pore. ○ Each subunit consists of four transmembrane domains (M1-M4) with the N-terminus located extracellularly and the C-terminus intracellularly. 3. Mechanism of Action: ○ When acetylcholine (ACh) binds to the nicotinic receptor at the neuromuscular junction (NMJ), the receptor undergoes a conformational change. ○ This change opens the central pore of the receptor, allowing the passage of ions across the cell membrane. ○ Specifically, sodium ions (Na+) flow into the muscle cell, and potassium ions (K+) flow out. This movement of ions generates an end-plate potential and initiates muscle fiber depolarization. 4. Function: ○ Nicotinic receptors are responsible for mediating fast excitatory neurotransmission at the NMJ. ○ They play a critical role in initiating muscle contraction by transmitting the signal from the motor neuron to the skeletal muscle fiber. Muscarinic Receptors: 1. Type: Metabotropic receptors. 2. Structure: ○ Muscarinic receptors belong to the family of G protein-coupled receptors (GPCRs). ○ They have seven transmembrane domains (M1-M7) that span the cell membrane. 3. Mechanism of Action: ○ When ACh binds to muscarinic receptors, it activates intracellular signaling pathways indirectly through G proteins. ○ The activation of muscarinic receptors leads to the dissociation of the G protein into its α, β, and γ subunits. ○ These subunits then modulate various intracellular effector systems such as adenylyl cyclase, phospholipase C, or ion channels, depending on the type of G protein associated with the receptor subtype. 4. Function: ○ Muscarinic receptors are found in various tissues and organs throughout the body, including smooth muscle, cardiac muscle, glands, and certain neurons. ○ They mediate a wide range of physiological responses, including regulation of heart rate, smooth muscle contraction, glandular secretion, and modulation of neurotransmitter release. Comparison: Activation: Nicotinic receptors directly open ion channels upon ACh binding, leading to rapid ion flux and membrane depolarization. Signal Transduction: Muscarinic receptors indirectly modulate cellular responses through intracellular signaling cascades involving G proteins and secondary messengers. Subunit Composition: Nicotinic receptors are pentameric (composed of 5 subunits), while muscarinic receptors are monomeric with 7 transmembrane domains. Understanding these differences is crucial in pharmacology and medicine, as drugs targeting nicotinic and muscarinic receptors can have distinct effects and therapeutic applications based on their mechanism of action and distribution in the body. Neuromuscular Blocking Agents Neuromuscular blocking agents (NMBAs) act at the nicotinic acetylcholine receptors (nAChRs) located at the neuromuscular junction (NMJ) of skeletal muscles. These receptors are specific to the somatic nervous system, which controls voluntary muscle movement. Here's a breakdown of their use and considerations: Types of Neuromuscular Blocking Agents: 1. Non-depolarizing NMBAs: ○ Examples: Rocuronium, Vecuronium, Atracurium, Pancuronium. ○ Mechanism: Competitively block nicotinic receptors at the NMJ, preventing the binding of acetylcholine (ACh) and thereby inhibiting muscle contraction. ○ Action: Induce muscle relaxation by preventing nerve impulses from reaching the muscles, leading to temporary paralysis. ○ Reversal: Can be reversed with acetylcholinesterase inhibitors like neostigmine and edrophonium, which increase ACh levels at the NMJ. 2. Depolarizing NMBA: ○ Example: Succinylcholine (Suxamethonium). ○ Mechanism: Initially binds and activates nicotinic receptors, causing depolarization and muscle fasciculations. However, it persistently activates these receptors, leading to a prolonged depolarizing blockade. ○ Action: Induces muscle paralysis rapidly, but with a longer duration compared to non-depolarizing agents. ○ Reversal: Cannot be effectively reversed with acetylcholinesterase inhibitors due to its unique mechanism. Uses of Neuromuscular Blocking Agents: Intubation: Facilitates endotracheal intubation by inducing muscle relaxation, which aids in the insertion of a breathing tube into the trachea for mechanical ventilation. This is typically done under anesthesia to prevent discomfort and ensure safe insertion. Surgery: Used during surgical procedures to achieve muscle relaxation, allowing surgeons to perform operations that require immobilization of skeletal muscles. Electroconvulsive Therapy (ECT): Administered to induce temporary muscle paralysis during ECT procedures, which are used in psychiatric treatment for severe depression and other mental health conditions. Considerations: Administration: NMBAs should be administered and monitored by trained medical personnel in controlled environments such as operating rooms or intensive care units. Reversibility: Non-depolarizing NMBAs can be reversed using acetylcholinesterase inhibitors after surgery or procedures to restore muscle function. However, succinylcholine's effects cannot be readily reversed using these agents. Safety: Due to their potential to cause profound muscle paralysis, careful dosing and monitoring are essential to prevent complications such as prolonged paralysis or inadequate muscle relaxation. Understanding the mechanism and uses of NMBAs is crucial for healthcare providers involved in anesthesia, surgery, and critical care, as these medications play a pivotal role in ensuring safe and effective patient care during various medical procedures. 3 Common NM blockers The danger associated with neuromuscular blocking agents (NMBAs), such as succinylcholine (Succ), cisatracurium (Cis), and rocuronium (Roc), lies in their potential to cause respiratory muscle paralysis. Here are the key considerations: 1. Respiratory Muscle Paralysis: ○ NMBAs block nicotinic acetylcholine receptors at the neuromuscular junction, leading to muscle relaxation and paralysis. ○ This effect includes the muscles involved in breathing, such as the diaphragm and intercostal muscles. ○ If not properly managed or monitored, respiratory muscle paralysis can lead to inadequate ventilation and respiratory arrest. ○ Therefore, these agents are typically administered alongside sedatives (e.g., benzodiazepines like midazolam) and analgesics (e.g., opioids like fentanyl) to ensure patient comfort and to facilitate controlled ventilation. 2. Timing and Reversibility: ○ Succinylcholine, although fast-acting and short-lasting, carries the risk of prolonged muscle paralysis in individuals with genetic variations affecting its metabolism (e.g., pseudocholinesterase deficiency). ○ Non-depolarizing NMBAs like cisatracurium and rocuronium have longer durations of action, which can prolong muscle paralysis beyond the intended period if not monitored closely. ○ Reversal agents such as acetylcholinesterase inhibitors (e.g., neostigmine) can be administered post-procedure to help reverse the effects of non-depolarizing agents, but they do not reverse succinylcholine's effects effectively. 3. Monitoring and Safety Protocols: ○ Continuous monitoring of respiratory function, including pulse oximetry and end-tidal carbon dioxide monitoring, is crucial during and after NMBA administration. ○ Anesthesia providers and critical care teams must be trained to recognize signs of inadequate ventilation promptly and to intervene with appropriate airway management techniques. ○ Strict adherence to dosing protocols, patient assessment, and preparation are essential to mitigate the risks associated with NMBA use, particularly in emergency or critical care settings. In summary, while neuromuscular blocking agents are indispensable for facilitating procedures like intubation, surgery, and ECT, their use requires vigilant monitoring and adherence to safety protocols to prevent complications, particularly respiratory muscle paralysis, which poses a significant risk to patient safety. 3.5 Adrenergic Agonists Adrenergic agonists are drugs that stimulate adrenergic receptors by mimicking the effects of endogenous catecholamines like norepinephrine and epinephrine. They are used to manage various cardiovascular, respiratory, and allergic conditions. Sympathomimetic Effects Sympathetic Nervous System - Innervating smooth muscles, glands - How can we stimulate glands and muscles in a sympathetic manner? - Direct Activation: Adrenergic agonists bind directly to adrenergic receptors (alpha and beta) to stimulate them. - Receptor Types: - Alpha Receptors: Alpha-1 and Alpha-2. - Beta Receptors: Beta-1, Beta-2, and Beta-3. Catecholamines: Natural or synthetic compounds (e.g., epinephrine, norepinephrine, dopamine, dobutamine). Non-Catecholamines: Synthetic drugs with longer durations of action (e.g., albuterol, phenylephrine). Arenal Medulla Receptor Specificity and Effects: Alpha-1 Agonists: Vasoconstriction, increased blood pressure, mydriasis. Alpha-2 Agonists: Inhibit norepinephrine release, decrease blood pressure. Beta-1 Agonists: Increase heart rate and contractility. Beta-2 Agonists: Bronchodilation, vasodilation, relaxation of uterine smooth muscle. Beta-3 Agonists: Lipolysis in adipose tissue, relaxation of bladder detrusor muscle. First Few slides are Intro to All Adrenergic Receptors Subcategories: A and B, both have agonist and antagonist NOTE: We also have indirect that don’t bind to a receptor, instead they impact the synsthe sis of NE and EPI. Mimic actions of Noradrelnaline and Epinephrine (Adrenaline) Sympathomimetics and those that block are called sympatholytics Receptor Effect Examples of Type s Agonists Alpha- Vasoconstriction, increased blood pressure, Phenylephrine, 1 mydriasis norepinephrine Alpha-2 Decreased norepinephrine release, lower Clonidine, methyldopa BP Beta-1 Increased heart rate and Dobutamine, isoproterenol contractility Beta-2 Bronchodilation, vasodilation Albuterol, salmeterol, terbutaline Beta-3 Lipolysis, bladder detrusor muscle relaxation Mirabegron 5 Main Steps in Adrenergic Neurotransmission: Alpha 1 and Alpha 2 Receptors Alpha-1 and Alpha-2 Adrenergic Receptors Adrenergic receptors are a class of G protein-coupled receptors that are targets of the catecholamines like norepinephrine and epinephrine. These receptors are divided into alpha and beta receptors, with further sub-classifications. Here, we will focus on alpha-1 and alpha-2 adrenergic receptors. Alpha-1 Adrenergic Receptors Location: Found primarily on the smooth muscles of blood vessels, the eye, bladder, and prostate. Function: Vasoconstriction: When activated, alpha-1 receptors cause the smooth muscles of blood vessels to contract, leading to vasoconstriction and increased blood pressure. Pupil Dilation: In the eye, alpha-1 activation leads to contraction of the radial muscle of the iris, resulting in pupil dilation (mydriasis). Bladder and Prostate: Alpha-1 receptors are also present in the bladder and prostate, where their activation can cause contraction of the bladder sphincter and the prostate, affecting urinary retention. Therapeutic Use of Alpha-1 Agonists: Nasal Decongestants: Drugs like phenylephrine act on alpha-1 receptors to constrict blood vessels in the nasal mucosa, reducing congestion. Hypotension: Used to increase blood pressure in cases of acute hypotension. Adverse Effects: Hypertension: Excessive vasoconstriction can lead to high blood pressure. Urinary Retention: Increased tone in the bladder sphincter can cause difficulty in urination. Alpha-2 Adrenergic Receptors Location: Predominantly found on presynaptic nerve terminals but also present in the central nervous system (CNS) and various other tissues. Function: Inhibition of Norepinephrine Release: Activation of presynaptic alpha-2 receptors inhibits the release of norepinephrine, decreasing sympathetic outflow. CNS Effects: In the CNS, alpha-2 receptors help reduce sympathetic activity, leading to sedative and hypotensive effects. Therapeutic Use of Alpha-2 Agonists: Antihypertensive Agents: Clonidine and methyldopa are examples of alpha-2 agonists that reduce blood pressure by decreasing sympathetic outflow. Sedation: Used in some anesthetic protocols for their sedative effects. Withdrawal Symptoms: Can help manage symptoms of o