Pes Revision PDF
Document Details
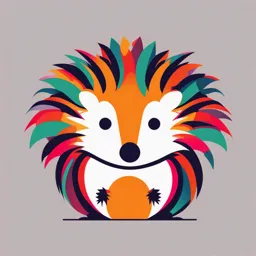
Uploaded by WellKnownBirch
SKEMA Business School
Tags
Summary
This document contains revision notes for a session on various aspects of soil and agriculture, including weathering, microorganisms, the carbon cycle, and soil threats. It also discusses modern agricultural techniques such as aquaponics, integrated pest management, and composting.
Full Transcript
SESSION 6 1. What is weathering and its 3 different forms? (Slide 14) Weathering is the gradual breakdown of rocks to form soil. It occurs in three forms: Physical Weathering: Breakdown through mechanical actions like temperature changes, abrasion, or frost. Chemical Weathering...
SESSION 6 1. What is weathering and its 3 different forms? (Slide 14) Weathering is the gradual breakdown of rocks to form soil. It occurs in three forms: Physical Weathering: Breakdown through mechanical actions like temperature changes, abrasion, or frost. Chemical Weathering: Breakdown via chemical reactions, such as minerals reacting with water, air, or other chemicals. Biological Weathering: Breakdown by living organisms, e.g., plant roots splitting rocks or burrowing animals allowing air and water to penetrate. 2. Explain the 3 mechanisms responsible for the boost in plant growth associated with microorganisms. (Slide 33) Microorganisms enhance plant growth through: 1. Manipulating hormonal signaling of plants to promote growth. 2. Repelling or outcompeting pathogenic microbes that could harm plants. 3. Increasing the bioavailability of soil nutrients for easier uptake by plants. 3. List 4 benefits of soil microbes. (Slide 38) Improve soil structure. Enhance nutrient availability. Protect plants from pathogens. Contribute to the decomposition process, forming humus. 4. Describe the role of soil in the carbon cycle. (Slides 40-41) Soils act as a major carbon sink, storing over three times more carbon than the atmosphere. Carbon is added to the soil through photosynthesis and organic matter decomposition. Carbon losses occur through respiration and oxidation, but healthy soil management can sequester more carbon and mitigate greenhouse gas emissions. 5. Describe 4 soil threats. (Slides 49-58) 1. Deforestation: Leads to soil erosion and loss of fertility. 2. Salinization: Accumulation of salts in the soil, reducing its productivity. 3. Compaction: Reduces soil aeration and water infiltration. 4. Erosion: Loss of topsoil due to wind or water. 6. What are the three major technological advances that define “modern agriculture”? (Slide 76) 1. Development of irrigation systems. 2. Use of synthetic fertilizers. 3. Application of synthetic pesticides. 7. List 4 natural capital degradations resulting from modern agricultural practices and give 2 examples for each. (Slide 96) 1. Soil Degradation: ○ Loss of organic matter. ○ Soil compaction. 2. Water Contamination: ○ Fertilizer runoff leading to eutrophication. ○ Pesticides polluting groundwater. 3. Biodiversity Loss: ○ Reduction of pollinator populations. ○ Habitat destruction from monocultures. 4. Climate Change Contribution: ○ Increased greenhouse gas emissions from fertilizers. ○ Carbon release from soil degradation. SESSION 7 Aquaponics (Slides 48-52) Aquaponics is a sustainable agricultural system combining aquaculture (raising fish) and hydroponics (soilless plant cultivation). Fish waste provides nutrients for plants, and plants filter the water for the fish. This symbiotic system is highly water-efficient, using only 1/6th of the water required for traditional farming to produce 6–8 times more food per acre. It avoids soil-borne diseases and eliminates the need for chemical fertilizers or pesticides. However, it demands substantial initial investment and expertise in both aquaculture and hydroponics management. Research highlights its potential to improve food security in arid regions and urban areas with non-arable land. Reference: Love et al. (2015) in Aquaponics—Integrated Fish and Plant Farming: A Sustainable Practice for Food Security. Integrated Pest Management (IPM) (Slides 53-58) IPM is an ecosystem-based strategy to manage pests effectively with minimal environmental impact. It integrates biological controls (natural predators, parasites), cultural practices (crop rotation, resistant varieties), and precise chemical use when necessary. Farmers begin with preventive measures and use pesticides only after pest populations exceed economic thresholds. IPM has been successful globally, with examples like reducing pesticide use by over 50% in Sweden and Denmark. Although more knowledge-intensive, IPM reduces long-term costs and environmental harm. Reference: Pretty and Bharucha (2015) in Integrated Pest Management for Sustainable Agriculture. Composting (Slides 39-41) Composting is the aerobic decomposition of organic waste to produce nutrient-rich soil amendments. It reduces landfill waste and methane emissions while enhancing soil structure, water retention, and fertility. Composting also minimizes reliance on synthetic fertilizers and improves the soil's carbon storage capacity. Cities like San Francisco have diverted over 80% of their waste from landfills through large-scale composting initiatives. Reference: Brown et al. (2008) in Compost Science & Utilization. Agroforestry (Slides 12-23) Agroforestry integrates trees and shrubs with crops or livestock. This approach enhances biodiversity, reduces erosion, and sequesters carbon through deeper root systems and long-term biomass accumulation. Practices include silvopasture, riparian buffers, and windbreaks. Agroforestry supports sustainable food production while preserving ecosystem services. For instance, hedgerows in California's tomato fields sequestered 18% of farm carbon on just 6% of the land. Reference: Jose (2009) in Agroforestry Systems: Ecological and Economic Benefits. Conservation Tillage (Slides 2-11) Conservation tillage reduces soil disturbance by leaving crop residues on at least 30% of the soil surface. Techniques include no-till, strip-till, and ridge-till. Benefits include improved carbon sequestration, reduced erosion, enhanced moisture retention, and lower labor requirements. While initial yields may drop, long-term studies show yield improvements of up to 70%. Challenges include pest management and upfront costs for equipment and cover crops. Reference: Lal et al. (2007) in Conservation Tillage: Enhancing Soil Carbon Sequestration. Mob Grazing (Slides 33-38) Mob grazing mimics natural grazing patterns by concentrating livestock in small areas for short durations, followed by long recovery periods. This promotes even manure distribution, deeper root growth, and increased plant diversity. It improves drought resilience and reduces soil compaction. However, careful planning is needed to avoid overgrazing and ensure balanced stocking densities. Reference: Pineiro et al. (2010) in Effects of Grazing on Soil Organic Matter in Grasslands. SESSION 8 1. What is a watershed? (Slide 8) A watershed, also known as a drainage basin, is the land area from which surface runoff drains into a specific water body, such as a stream, lake, or wetland. It collects precipitation that does not infiltrate the ground or return to the atmosphere via evaporation. 2. How has freshwater use evolved during the last century? What are the main driving forces behind this growth? (Slides 15-16) Evolution: Global freshwater use has increased sixfold over the last century and continues to grow by approximately 1% annually since the 1980s. Driving Forces: 1. Population Growth: Rising demand from an increasing global population. 2. Improved Living Standards: Higher water consumption for domestic and industrial needs. 3. Changing Consumption Patterns: Dietary shifts, particularly increased meat consumption. 4. Expansion of Irrigated Agriculture: Greater reliance on water-intensive farming techniques. 3. What are the 5 consequences of the depletion of groundwater? (Slides 78-86) 1. Lowering of the Water Table: Excessive pumping causes wells to dry up. 2. Decline in Surface Water: Reduced groundwater replenishment decreases lake and stream levels. 3. Increased Costs: Deeper wells and longer transport increase expenses. 4. Saltwater Intrusion: Coastal areas may experience groundwater contamination by saltwater. 5. Land Subsidence: Loss of soil stability leads to sinking ground and infrastructure damage. 4. What defines a common good? What is the tragedy of the common? (Slides 92-96) Common Good: A resource system (e.g., water) that: ○ Exhibits subtractability (one user's consumption reduces availability for others). ○ Faces difficulty in exclusion (hard to deny access to users). Tragedy of the Common: Occurs when individuals overexploit a shared resource for personal gain, leading to resource depletion due to lack of coordinated management. 5. List 3 principles and 3 attributes for community governance of common goods according to Elinor Ostrom. (Slides 110-115) Principles: 1. Shared Vision: Consensus on managing the resource and understanding the consequences of actions. 2. Self-Organization and Conflict Resolution: Ability to establish rules and resolve disputes locally. 3. Autonomy: Community autonomy in defining use rights with proper sanctions. Attributes: 1. Resource Renewal: Resources must not be overly damaged and capable of natural replenishment. 2. Monitoring Mechanisms: Indicators to track resource quantity and quality. 3. Predictability: Understanding future resource availability. 6. What are the 4 major issues in water management? (Slide 119) 1. Growing Demand Across Sectors: Balancing competing uses. 2. Extension of Rules and Usage Rights: Clarifying regulatory frameworks. 3. Overlapping Boundaries: Managing shared resources across jurisdictions. 4. Funding Shortages: Insufficient investment in infrastructure and regulation. 7. Describe the Nexus. (Slides 137-144) The Nexus approach addresses the interlinkages between water, energy, and food systems: Concept: Highlights the scarcity of resources and the need for coordinated management to avoid trade-offs that compromise sustainability. Key Interconnections: 1. Water-Energy: Energy production (e.g., hydroelectricity, cooling thermal plants) is water-intensive, and water management requires energy. 2. Water-Food: Agriculture is the largest consumer of water, impacting quality and availability. 3. Food-Energy: Energy is vital for food production, transport, and storage. Challenges: Integration across sectors and governance complexities hinder implementation. SESSION 9 1. What are Rare Earth Elements? (Slides 50-54) Rare Earth Elements (REE) are a group of 17 metals used in high-tech and clean-energy products. Their unique properties, such as magnetism, luminescence, and strength, make them critical for renewable energy technologies (e.g., wind turbines and solar panels), LED technologies, and electronics. Despite their name, they are not rare but are chemically similar, making separation into individual elements difficult. China dominates production, with 90% of global supply, much of it from the Bayan Obo Mine in Inner Mongolia. 2. Describe the global supply of lithium. (Slides 63-64) Geographical Concentration: 90% of lithium production comes from three countries: Australia, Chile, and China. Lithium is primarily extracted from salt brines and hard rock deposits. Processing Dominance: 65% of global lithium refining capacity is concentrated in China, where lithium is converted into lithium carbonate or lithium hydroxide for battery use. Limited geographical distribution creates a tight market, despite lithium’s abundance in the Earth’s crust. 3. What are three drivers behind metal demand during the 21st century? (Slide 69) 1. Infrastructure Development: Urbanization and industrialization require large quantities of metals for construction and transportation networks. 2. Electronic Revolution: Growth in consumer electronics and digital technologies increases demand for specialty metals. 3. Shift to Renewable Energy: Transition to clean energy technologies, such as solar panels and electric vehicles, relies heavily on metal-intensive materials. 4. Describe 3 environmental consequences of metal mining. (Slides 85-94) 1. Land Degradation: Mining alters landscapes, destroys ecosystems, and limits land use for other purposes. 2. Water Pollution: Acid mine drainage contaminates water sources with sulfuric acid and toxic metals like arsenic and mercury, harming aquatic ecosystems and human health. 3. Air Pollution: Mining releases dust, particulates, and harmful emissions, impacting air quality and contributing to respiratory illnesses. 5. Explain the relationship between energy transition and metals in 3 points. (Slides 126-128) 1. Mineral Intensity: Clean energy technologies require more materials than fossil fuel-based technologies. Achieving ambitious climate goals will significantly increase the demand for metals. 2. Resource Specificity: Different minerals have varying demand risks depending on their role in specific technologies (e.g., lithium for batteries, cobalt for EVs). 3. Mining Necessity: Despite increased recycling, mining remains essential to supply the critical minerals needed for the energy transition. 6. Explain the concept of the Life Cycle Approach. (Slide 132) The Life Cycle Approach (LCA) analyzes the environmental impacts of metals throughout their entire life cycle, including: Production: Extraction and refining of raw materials. Use: Application in products and technologies. End-of-Life: Recycling, reuse, or disposal. LCA provides a comprehensive view, helping stakeholders make informed decisions to minimize environmental footprints. SESSION 10 7. What are 4 factors determining the success in metal recycling? (Slides 143-144) 1. Intrinsic Value of Materials: High-value materials incentivize recycling. 2. Efficiency of Recycling Processes: Advanced physical and chemical techniques ensure effective recovery. 3. Waste Collection and Pre-Sorting: Organized systems improve material separation and recycling rates. 4. Robust Metallurgical Industry: Diverse and adaptable infrastructure supports the recycling of various metals. 1. List 4 different forms of energy with one example for each. (Slide 37) 1. Thermal Energy: Heat from geothermal sources or combustion. 2. Mechanical Energy: Kinetic energy in a wind turbine turning blades. 3. Chemical Energy: Stored energy in fossil fuels like coal. 4. Electrical Energy: Generated electricity from hydropower. 2. What is the origin of fossil fuels? (Slides 67-69) Fossil fuels are formed from ancient organic material: Initial Stage: Organic matter accumulates in low-oxygen environments like swamps and ocean floors. Formation of Black Shale: Organic-rich sediments turn into black shale with over 5% organic matter. Thermal Transformation: With heat and pressure over millions of years: ○ At ~90°C: Formation of liquid oil. ○ At ~150°C: Formation of natural gas. These fuels are concentrated energy sources due to their long formation process. 3. What are 3 types of non-conventional oil and two limitations to their production? (Slides 73-87) 1. Shale Oil: Extracted from oil shale rock by mining and heating. ○ Limitations: Low net energy yield, high environmental impact. 2. Tar Sands: Bitumen mixed with sand and clay, processed with water and energy. ○ Limitations: High water usage, significant greenhouse gas emissions. 3. Extra-Heavy Oil: Found in Venezuela; highly viscous and difficult to extract. ○ Limitations: Expensive to extract, short lifespan of wells. 4. What are 3 reasons why liquid fossil fuels are so dominant? (Slides 89-91) 1. High Energy Density: Easily stored and transported, providing substantial energy in small volumes. 2. Cost-Effectiveness: Relatively low cost compared to alternatives. 3. Versatility: Used in various applications, including transportation, heating, and petrochemical industries. 5. Describe fission nuclear energy and how a nuclear power plant functions to produce electricity. (Slides 124-126) Fission Process: Large atomic nuclei (e.g., uranium-235) are split into smaller nuclei, releasing energy. Nuclear Power Plant Functioning: 1. Fuel: Enriched uranium-235 pellets are placed in fuel rods. 2. Reaction: Controlled nuclear fission generates heat. 3. Steam Generation: Heat converts water into steam. 4. Electricity Production: Steam spins a turbine connected to a generator, producing electricity. 6. What is the main downside of renewable sources of energy like wind and solar? Propose 3 potential solutions to this problem. (Slides 190-195) Downside: Intermittency due to weather variability and time of day. Solutions: 1. Energy Storage: Batteries, pumped hydro, or thermal storage to balance supply and demand. 2. Demand Response Programs: Encouraging flexible energy use during peak production times. 3. Grid Modernization: Smart grids to integrate diverse energy sources and improve reliability. 7. Explain the Jevons paradox and rebound effect. (Slides 199-203) Jevons Paradox: Increased resource efficiency can lead to higher consumption of the resource due to reduced costs and increased usage. Rebound Effect: ○ Direct Rebound: Efficiency lowers costs, increasing consumption of the same service. ○ Indirect Rebound: Savings are redirected to other energy-intensive goods or services. This paradox highlights challenges in achieving energy savings through efficiency alone.