Part IIE_RegExpEuks.pptx PDF: Control of Gene Expression (Eukaryotes)
Document Details
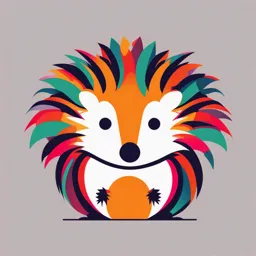
Uploaded by SaneHilbert
University of St. Thomas (TX)
Tags
Summary
This presentation discusses the control of gene expression in eukaryotes. It details various levels of regulation, from genome structure to post-transcriptional mechanisms, including epigenetic modifications and the role of non-coding RNAs. The presentation also touches upon transcriptional factors and the regulatory networks involved in cellular differentiation.
Full Transcript
2 PART II-E Control of gene expression (Eukaryotes) 3 Regulation of gene expression occurs at several levels: 1. Genome/chromatin structure 2. Epigenetic profile and imprinting 3. Transcriptional r...
2 PART II-E Control of gene expression (Eukaryotes) 3 Regulation of gene expression occurs at several levels: 1. Genome/chromatin structure 2. Epigenetic profile and imprinting 3. Transcriptional regulation 4. Post-transcriptional regulation 5. Regulation of Translation 6. Regulation via non-coding RNAs (embedded in other sections) 4 Genome and chromatin structure Euchromatin and heterochromatin Transcription is possible from euchromatic DNA. CTCF delimits euchromatic/heterochromatic boundaries. Chromosome territories Domains closer to the nuclear envelope are silenced. TADs Different cell types have distinct profiles or active/inactive TADs. ncRNAs ncRNAs can bind DNA and proteins that bind DNA or RNA. Found in scaffolding complexes that impact chromatin structure. Can recruit chromatin remodeling complexes. 5 6 7 Epigenetic profile and imprinting Epigenetic mechanisms: histone modifications, nucleosome positioning and DNA methylation. Chromatin structure is tightly associated with the epigenetic profile. Epigenetic code: Chemical modifications of the histones produce a variety of chromatin states, each with different levels of transcription. Chromatin binding proteins recognize epigenetic marks and help establish open/closed chromatin. 8 Epigenetic profile and imprinting DNA methylation (5-meC of CpG): Associated with transcriptional repression. Transcription factors cannot bind methylated DNA. Specific proteins bind methylated DNA for chromatin organization and epigenetic maintenance. Epigenetic memory: remains through mitosis (DNMT1 methylase). CpG islands of gene-rich areas are not methylated. During gametogenesis, the epigenome must be reset to a totipotent state. Imprinting: About 150 human genes are imprinted. Differential expression from maternal/paternal chromosome. Most common: methylation of maternal DMR. 9 10 11 12 13 14 Epigenetic profile and imprinting X-inactivation: Epigenetic process that occurs during early embryogenesis: Random X inactivation in blastula. Balances expression of X-linked genes. Epigenetic memory: Epigenetic marks persist during mitosis. Mechanism is not well understood, but evidence supports transgenerational epigenetic memory. 15 ~ 19 Kb Xi is heterochromatic, except about 15% of genes outside PAR XIC: X inactivation center (1 Mb) 19 Kb ncRNA (X inactivation specific transcript) binds more abundantly to the chromosome that will be inactivated 16 17 18 19 Transcriptional regulation Nucleosome positioning: Promoters are usually devoid of nucleosomes for PIC assembly. Chromatin remodeling complexes. Promoters: About half of mammalian genes have alternate promoters. Enhancers: Cell- and tissue-specific gene expression. DNA looping (cohesin, mediator proteins) is required. Structure of TADs can facilitate interactions. Transcription factors and the epigenetic landscape: Thousands of transcriptional factors (general, tissue-specific) allow development, definition of cellular identity and expression in cells. As cells differentiate, they become more epigenetically restricted. 20 21 DMD has 8 alternate promoters 22 Waddington’s epigenetic landscape Master transcription factors + Super-enhancers Increase in epigenetic restriction Subordinate transcription factors + Enhancers 23 Transcriptional regulation Transcription elongation: Sometimes transcription factors bind the promoter, but elongation does not proceed. TFIIH allows DNA pol to access the promoter. DNA pol must be phosphorylated by p-TEFb to proceed beyond abortive transcription. Termination of transcription: PAS (DNA Pol II). About half of mammalian genes use alternative cleavage and polyadenylation signals. ncRNAs: Anti-sense and bi-directional transcription of ncRNAs interferes with sense transcription, DNA methylation, modifying histone code, or inducing heterochromatic states. Also silence transposons. 24 25 a| Aberrant transcriptional extension of LUC7L produces an antisense transcript that overlaps with HBA1 gene, which methylates the HBA1 promoter and represses its expression. b| The nascent antisense transcript ANRIL recruits a repressive complex (PRC2), which induces H3K27me. This represses transcription of the tumor suppressor locus CDKN2B– CDKN2A57 and also leads to long-term promoter DNA methylation at this locus. c| HOX transcript antisense RNA (HOTAIR) silences the HOXD locus in trans through PRC2 recruitment. d| Antisense transcripts that originate from internal cryptic promoters and modify the chromatin of their associated sense promoters by depositing H3K4me2, modulating the binding of the histone deacetylase Set3 and gene 26 Post-transcriptional regulation Alternative splicing: Can be modulated by splicing enhancers/suppressors to produce strong/weak splice sites in a tissue-dependent manner. Impact: Sub-cellular compartment signal, transmembrane domain, temporal-special specific isoforms, specific post-translational modifications, poison exons. Epigenetic marks on DNA can impact splicing by modulating RNAP RNA turnover RNA editing 27 Regulation of translation Leader peptides: About 1/3 of human transcripts have additional upstream ORF. Structure of mRNA: Stem-loop structures can impact ribosome advancement. Bind RNA-binding regulatory proteins. ncRNAs: miRNAs + siRNAs + piRNAs can repress expression of target mRNAs. miRNA can bind 3’UTR to modulate rate of translation. miRNAs can compete for the target mRNA (regulatory network of modest effects). 28 uORF 29 This mechanism counterbalances both cellular iron deficiency and iron overload. Cellular iron homeostasis: iron responsive element (IRE)/ iron regulatory protein (IRP) system. IRP1 and IRP2 bind to IREs present in either the 5’ UTR or 3’ UTR of mRNAs and regulate their translation and stability, respectively. In iron-depleted cells, IRPs bind to an IRE localized in the 5’ UTR of mRNAs to repress translation, while IRP binding to IREs in the 3’ UTR stabilizes mRNAs. 30 Summary 31 ADDITIONAL SLIDES 32 Example TPO gene and HR gene, Strachan page 352, 353. Human protein atlas: This gene encodes a protein that is involved in hair growth. This protein functions as a transcriptional corepressor of multiple nuclear receptors, including thyroid hormone receptor, the retinoic acid receptor-related orphan receptors and the vitamin D receptors, and it interacts with histone deacetylases. The translation of this protein is modulated by a regulatory open reading frame (ORF) that exists upstream of the primary ORF. Mutations in this upstream ORF cause Marie Unna hereditary hypotrichosis (MUHH), an autosomal dominant form of genetic hair loss. Mutations in this gene also cause autosomal recessive congenital alopecia and atrichia with papular lesions, other diseases resulting in hair loss. Two transcript variants encoding different isoforms have been found for this gene. [provided by RefSeq, Oct 2014] 33 Regulation of Gene Expression in Eukaryotes 34 35 36 37 38 39 40 41 FIGURE 2. The epigenetic control of rRNA: pRNA and PAPAS RNAPI binding to rDNA promoter gives rise to the transcription of pRNA, whose sequences match that of rDNA promoter. In contrast, the antisense transcription of rDNA, mediated by RNAPII, gives rise to PAPAS, spanning from rDNA gene body to intergenic, promoter, and enhancer regions (Figure, central panel). pRNA can mediate the silencing of rDNA loci by (A) directly recruiting DNMT3b, leading to de novo methylation and heterochromatinization; (B) binding NoRC and HDAC, promoting nucleosome sliding and deacetylation, respectively. (C) PAPAS leads to rDNA silencing by recruiting SUV4 on rDNA promoter. (D) PAPAS can associate with the chromatin remodeling complex CHD4/NuRD and guide it on the rDNA locus to promote nucleosome shifting and transcriptional repression of rDNA in a heat stress-dependent manner. https://doi.org/10.3389/fcell.2023.1123975 42 43 Non-coding RNAs Transcription Translation Transposon silencing Chromatin structure