Pallor Case Study - C.A. - PDF
Document Details
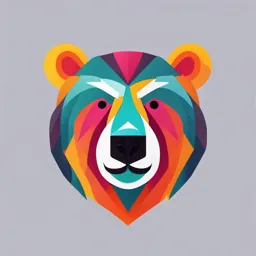
Uploaded by RecommendedCedar8572
Tags
Summary
This document is a case study of a patient with complaints of pallor and fatigue. The patient has heavy menstrual bleeding and presents with symptoms consistent with anemia. Initial diagnostic tests and a proposed treatment plan are included in the case study.
Full Transcript
Patient ID: C.A., 18 years old, single, from Camalig, Albay Handout 1 Chief Complaint: Maputla at madaling mapagod History of Present Illness C.A. is an 18-year-old woman who presents with a 3-month history of progressive pallor and easy fatigability. She reports feeling unusually tired...
Patient ID: C.A., 18 years old, single, from Camalig, Albay Handout 1 Chief Complaint: Maputla at madaling mapagod History of Present Illness C.A. is an 18-year-old woman who presents with a 3-month history of progressive pallor and easy fatigability. She reports feeling unusually tired after minimal physical exertion, such as climbing stairs or carrying groceries. Over the past month, she has also noticed occasional dizziness, shortness of breath on exertion, and difficulty concentrating in school. She denies chest pain, palpitations, or syncope. She mentions heavy menstrual periods, with bleeding lasting 7-8 days and requiring more than 8 pads per day. There is no history of intermenstrual bleeding or post-coital bleeding. She denies significant weight loss, fever, night sweats, or unusual bruising. Her diet consists mostly of rice, vegetables, and fish, with occasional meat intake. She acknowledges skipping meals occasionally due to a busy school schedule. There is no history of similar complaints in the past or known chronic illnesses. Past Medical History No previous hospitalizations or surgeries. No known allergies. No prior diagnosis of anemia or blood disorders. Immunization status up-to-date. Family History No family history of anemia, bleeding disorders, or chronic illnesses. Parents and siblings are healthy. Social History C.A. is a college student and lives with her parents. No history of smoking, alcohol consumption, or illicit drug use. No recent travel or exposure to infectious diseases. Handout 2 Physical Examination General Appearance: Alert and oriented but appears pale and tired. Vital Signs: Temperature: 36.7°C Blood Pressure: 100/60 mmHg Heart Rate: 92 bpm (regular) Respiratory Rate: 18 breaths/min Skin: Pale skin and mucous membranes. No jaundice, cyanosis, or petechiae. No rashes, petechiae, or ecchymosis noted. HEENT: Pale conjunctiva. No icterus. Oral mucosa dry, no ulcers. No lymphadenopathy. Thyroid not enlarged. No neck vein distention No carotid bruits auscultated. Cardiovascular: Tachycardia, regular rhythm. No murmurs, rubs, or gallops. No chest deformities observed. Respiratory: Symmetrical chest expansion observed. Clear breath sounds bilaterally. Abdomen: Soft, non-tender, no organomegaly. Normoactive bowel sounds heard. No venous hum, or abdominal bruits detected. Extremities: No edema. Nails show koilonychia Neurological: Intact cranial nerves, normal motor strength and sensation. Motor strength is 5/5 in all extremities. Normal sensation and reflexes. No focal neurological deficits. Review of Systems General: Fatigue, pallor, occasional dizziness. Cardiovascular: Mild palpitations on exertion, no chest pain. Respiratory: Shortness of breath on exertion. Gastrointestinal: No abdominal pain, nausea, vomiting, or changes in bowel habits. Genitourinary: Heavy menstrual bleeding, no dysuria or hematuria. Neurological: Difficulty concentrating, no headaches, or limb weakness. Handout 3 Initial Diagnostic Tests Ordered 1. Complete Blood Count (CBC): o Hemoglobin: Low o Hematocrit: Low o Mean Corpuscular Volume (MCV): Low o Red Cell Distribution Width (RDW): Elevated o Platelets and WBC: Normal 2. Peripheral Blood Smear: o Hypochromic, microcytic red blood cells. 3. Serum Iron Studies: o Serum Iron: Low o Total Iron Binding Capacity (TIBC): High o Ferritin: Low 4. Reticulocyte Count: o Low, 5. Stool Occult Blood Test: o Negative 6. Urinalysis: o Normal. 7. Pelvic Ultrasound Assessment C.A. presents with signs and symptoms of iron deficiency anemia likely secondary to chronic blood loss from menorrhagia and suboptimal dietary intake. Plan 1. Iron Supplementation: o Oral ferrous sulfate 325 mg once daily with vitamin C to enhance absorption. 2. Dietary Counseling: o Increase intake of iron-rich foods (e.g., red meat, dark leafy greens, beans). 3. Menorrhagia Management: o Referral to gynecologist for evaluation and management of heavy menstrual bleeding. 4. Follow-Up: o Repeat CBC and iron studies in 4 weeks to assess response to treatment. 5. Consider Additional Testing: o Thyroid function tests if indicated. o Coagulation profile if bleeding symptoms worsen. Guide Questions: A. General Understanding of Pallor 1. What is pallor, and how is it clinically assessed? 2. What are the hematological conditions commonly associated with pallor? 3. How does pallor correlate with reduced hemoglobin levels and anemia? 4. What is the embryological origin of blood cells, erythropoiesis and how does it relate to conditions causing pallor? 5. What is the histological structure of the bone marrow, and how does it reflect blood production capacity? 6. What are the physiological functions of hemoglobin, types of hemoglobin, hemoglobin synthesis, and how does its deficiency lead to pallor? B. Pathophysiology of Anemia 1. What is the global epidemiology of anemia? 2. What are the different types of anemia? Prevalence and Etiology? 3. What are the mechanisms by which anemia causes pallor and easy fatigability? 4. How does chronic blood loss lead to iron deficiency anemia, considering red cell metabolism and biochemistry? 5. What is the impact of iron deficiency on the synthesis and function of hemoglobin? 6. How does the composition of blood (plasma vs. cellular components) change in anemia? 7. What is the role of erythropoietin in red cell production, and how is it affected in anemia? 8. What histological changes occur in the bone marrow during anemia? C. Clinical Presentation and Evaluation 1. Why is it important to take a detailed menstrual history in young women with pallor? 2. How do other symptoms like dizziness, shortness of breath, and koilonychia provide clues to the diagnosis? 3. What are the key findings in the physical examination of this patient that support the diagnosis of anemia? 4. How does the physiology of oxygen transport explain the systemic effects of anemia? 5. What are the components of blood cells, and how do their abnormalities manifest clinically? D. Diagnostic Approach 1. What laboratory tests are essential in evaluating anemia, and why? 2. How do the findings on CBC (e.g., low MCV, low hemoglobin, elevated RDW) confirm iron deficiency anemia? 3. What is the biochemical basis of microcytic hypochromic anemia? 4. How do tests like serum ferritin and TIBC provide insights into iron metabolism? 5. What additional tests can be performed to identify the underlying cause of anemia (e.g., iron studies, reticulocyte count, stool occult blood test)? 6. How does the peripheral blood smear reflect red cell morphology and production defects? E. Differential Diagnosis 1. What are the differential diagnoses for microcytic anemia? 2. How can thalassemia be distinguished from iron deficiency anemia using hematologic and biochemical tests? 3. What is the embryological basis of hemoglobinopathies like thalassemia? 4. What other non-hematologic conditions could contribute to pallor in this patient? 5. How does the physiology of red blood cell destruction differ in hemolytic anemia vs. iron deficiency anemia? F. Management of Iron Deficiency Anemia 1. What are the key principles in the treatment of iron deficiency anemia? 2. How does vitamin C enhance the absorption of iron supplements? 3. What is the biochemical mechanism of iron absorption in the gut? 4. What dietary changes should be recommended to this patient, focusing on iron-rich foods? 5. How does the body regulate iron storage and release during anemia? G. Special Considerations in Adolescents 1. What are the long-term effects of untreated anemia on growth and cognitive function in young individuals? 2. How does the physiology of adolescents make them more susceptible to anemia? 3. How can menstrual management (e.g., hormonal therapy) address the issue of menorrhagia in this patient? 4. How does red cell metabolism adapt (or fail to adapt) to increased oxygen demands in growing individuals? H. Prevention and Public Health 1. What strategies can be implemented to prevent iron deficiency anemia in resource-limited settings? 2. How does patient education play a role in preventing recurrent anemia? 3. What are the public health policies that could reduce the prevalence of anemia among adolescent females? 4. How do genetics and embryological factors influence the global burden of anemia? 5. What is the role of population-wide screening programs in detecting and managing anemia early? Tutor’s Guide A. General Understanding of Pallor 1. What is pallor, and how is it clinically assessed? Pallor refers to an abnormal pale appearance of the skin and mucous membranes due to reduced blood flow, hemoglobin concentration, or both. It is often associated with anemia but can also occur in conditions causing poor perfusion or reduced oxygenation. Clinical Assessment of Pallor a. Inspection of Key Areas: o Conjunctiva: Pull down the lower eyelid to assess the paleness of the inner conjunctiva. o Nail Beds: Check the color of the nail beds for reduced pinkness. o Palmar Creases: Observe the palmar creases for abnormal paleness. o Lips and Oral Mucosa: Examine the lips and inner mouth lining for reduced redness. o Face and General Skin Tone: Compare the overall complexion to the patient’s baseline or population norms. b. Correlation with Hemoglobin Levels: o Pallor is more likely to be clinically detectable when hemoglobin levels fall below 7–8 g/dL. o The degree of pallor may correlate with the severity of anemia but is not always reliable due to variations in skin pigmentation and lighting. c. Physical Conditions Affecting Pallor: o In darker-skinned individuals, pallor may be better appreciated in less pigmented areas such as the conjunctiva, lips, and nail beds. o Assess pallor under natural lighting, as artificial lighting can distort color perception. d. Associated Findings: o Pallor is often assessed along with other signs of anemia such as fatigue, dizziness, shortness of breath, and tachycardia. 2. What are the hematological conditions commonly associated with pallor? Pallor is a clinical manifestation of reduced blood flow, low hemoglobin levels, or both. In hematology, it is frequently associated with conditions that lead to anemia, impaired blood production, or increased destruction of red blood cells. Below are some key hematological conditions commonly linked to pallor: a) Anemia (Decreased Hemoglobin Levels) Iron Deficiency Anemia: o The most common cause of pallor globally, often due to chronic blood loss (e.g., heavy menstruation, gastrointestinal bleeding) or insufficient dietary iron. Vitamin B12 Deficiency (Pernicious Anemia): o Leads to megaloblastic anemia, characterized by pallor, fatigue, and neurological symptoms. Folate Deficiency Anemia: o Causes megaloblastic anemia, often due to poor diet, alcohol abuse, or malabsorption. Anemia of Chronic Disease (ACD): o Associated with chronic infections, inflammatory diseases, or malignancy, causing impaired iron utilization. b) Hemolytic Anemias (Increased Red Cell Destruction) Hereditary Hemolytic Disorders: o Conditions such as sickle cell disease, hereditary spherocytosis, and thalassemia can cause pallor due to chronic hemolysis and anemia. Acquired Hemolytic Anemia: o Autoimmune hemolytic anemia or hemolysis caused by infections, toxins, or drugs. c) Bone Marrow Disorders (Decreased Production of Blood Cells) Aplastic Anemia: o A condition in which the bone marrow fails to produce sufficient blood cells, leading to pallor, fatigue, and bleeding tendencies. Myelodysplastic Syndromes (MDS): o Group of disorders resulting in ineffective blood cell production, often seen in older adults. d) Hemoglobinopathies Thalassemias: o Genetic disorders affecting hemoglobin production, leading to pallor, microcytosis, and sometimes jaundice. Sickle Cell Disease: o Chronic anemia with pallor due to the rapid breakdown of abnormally shaped red blood cells. e) Blood Loss (Acute or Chronic) Acute Hemorrhage: o Sudden blood loss, such as from trauma or surgery, can cause pallor due to hypovolemia and decreased oxygen-carrying capacity. Chronic Blood Loss: o Conditions like gastrointestinal bleeding or heavy menstruation lead to iron deficiency anemia and pallor over time. f) Hypoproliferative Anemias Renal Failure-Associated Anemia: o Caused by decreased erythropoietin production from the kidneys, leading to reduced red blood cell production. Endocrinopathies (e.g., hypothyroidism): o Low metabolic demand and decreased erythropoiesis contribute to mild anemia and pallor. g) Leukemias and Lymphomas Acute Leukemia: o Infiltration of the bone marrow by malignant cells suppresses normal blood production, leading to anemia and pallor. Chronic Leukemia and Lymphoma: o Chronic infiltration and disruption of hematopoiesis can also cause pallor. h) Rare Causes Paroxysmal Nocturnal Hemoglobinuria (PNH): o A rare disorder causing intravascular hemolysis, leading to anemia and pallor. Congenital Dyserythropoietic Anemia (CDA): o Rare genetic disorders with ineffective red blood cell production and pallor. 3. How does pallor correlate with reduced hemoglobin levels and anemia? Pallor is one of the hallmark clinical signs of anemia and is closely related to reduced hemoglobin levels. The degree of pallor often reflects the severity of anemia and provides an indirect assessment of oxygen-carrying capacity. Below is a detailed explanation of the correlation: a) Hemoglobin and Skin/Mucosal Color Hemoglobin’s Role in Skin Color: Hemoglobin in red blood cells gives blood its red color. Adequate blood flow and oxygenation contribute to the pinkish hue of the skin and mucous membranes. o In anemia, low hemoglobin levels reduce the redness of circulating blood, causing pallor. o Reduced red blood cells or hemoglobin also diminish the pinkish tone in vascular areas such as the conjunctiva, lips, and nail beds. b) Redistribution of Blood Flow in Anemia c) Compensatory Vasoconstriction: In anemia, the body redirects blood flow from less critical areas (e.g., skin) to vital organs (e.g., brain and heart) to maintain oxygen delivery. o This reduced blood flow to the skin and mucous membranes leads to pallor even if the anemia is mild. o The effect is more pronounced during severe anemia or hypovolemic states. d) Critical Hemoglobin Thresholds for Detecting Pallor Pallor is usually clinically detectable when hemoglobin levels drop significantly: o Mild Anemia: May not always cause visible pallor. o Moderate to Severe Anemia: Pallor becomes apparent when hemoglobin falls below 7–8 g/dL, depending on skin pigmentation and baseline blood flow. e) Oxygen Delivery and Tissue Hypoxia Anemia and Oxygen Transport: Hemoglobin binds and transports oxygen to tissues. Reduced hemoglobin impairs oxygen delivery, leading to tissue hypoxia and systemic signs such as fatigue and dyspnea, often accompanying pallor. o Tissue hypoxia may worsen pallor due to decreased metabolic activity in skin tissues. f) Differential Manifestations Based on Skin Pigmentation Light Skin: Pallor is more easily noticeable, especially in the face, palms, nail beds, and conjunctiva. Dark Skin: Pallor may be subtler and is best detected in less pigmented areas such as the conjunctiva, oral mucosa, and nail beds. g) Types of Anemia and Their Effects on Pallor Iron Deficiency Anemia: o Microcytic hypochromic anemia reduces hemoglobin concentration in red blood cells, making pallor prominent. Hemolytic Anemia: o Increased red cell destruction reduces hemoglobin levels, causing pallor. o Accompanying jaundice (due to bilirubin from red cell breakdown) may mask pallor. Megaloblastic Anemia: o Ineffective erythropoiesis leads to reduced functional hemoglobin and noticeable pallor. h) Limitations of Pallor as an Indicator of Hemoglobin Pallor is a subjective finding and may vary between individuals. o Poor lighting or naturally pale skin can lead to overestimation. o Conditions like vasoconstriction due to cold or shock may mimic anemia-induced pallor. 4. What is the embryological origin of blood cells, erythropoiesis and how does it relate to conditions causing pallor? The embryological origin of blood cells, known as hematopoiesis, occurs at different stages of development, with the primary sources being: a) Yolk Sac (Mesoblastic Stage): o During the early stages of embryonic development (around 2-8 weeks), the yolk sac is the first site of blood cell formation. This process is called primitive hematopoiesis. At this stage, blood cells are mostly primitive erythrocytes (red blood cells) and some other cell types, such as macrophages. These early blood cells are short-lived and are eventually replaced by cells from other hematopoietic sites. b) Liver and Spleen (Hepatic and Splenic Stages): o Between the 6th and 24th week of gestation, hematopoiesis shifts to the liver and spleen. This stage is called definitive hematopoiesis, where more mature and long-lasting blood cells are produced. This includes all major blood cell types: red blood cells, white blood cells, and platelets. c) Bone Marrow (Medullary Stage): o After the 24th week of gestation, the bone marrow becomes the primary site for hematopoiesis. By birth, the bone marrow is the main organ responsible for producing blood cells throughout life. In adults, hematopoiesis is restricted to the bone marrow of the ribs, sternum, pelvis, and vertebrae. Erythropoiesis Erythropoiesis, the process of red blood cell (RBC) production, is a tightly regulated physiological process that occurs in the bone marrow. It is essential for maintaining adequate oxygen delivery to tissues by producing RBCs with sufficient hemoglobin (Hb). Stages of Erythropoiesis Erythropoiesis begins with hematopoietic stem cells (HSCs) and progresses through distinct stages: a) Hematopoietic Stem Cells (HSCs) Location: Bone marrow niches. Function: Multipotent HSCs are progenitors capable of differentiating into all blood cell lineages. HSCs differentiate into common myeloid progenitors (CMPs) under the influence of cytokines and growth factors such as stem cell factor (SCF) and interleukin-3 (IL-3). b) Committed Progenitors CMPs further differentiate into erythroid progenitors, specifically: o Burst-forming unit-erythroid (BFU-E): Early erythroid progenitor sensitive to erythropoietin (EPO) and other cytokines. o Colony-forming unit-erythroid (CFU-E): A more mature erythroid progenitor with higher dependence on EPO for proliferation and survival. c) Erythroblast Stages The CFU-E cells give rise to immature erythroblasts. These stages are defined by morphological and functional changes: (a) Proerythroblasts First morphologically recognizable erythroid precursor. Large nucleus with dispersed chromatin. Begins to express hemoglobin synthesis enzymes and erythroid-specific surface markers. (b) Basophilic Erythroblasts Smaller than proerythroblasts. Cytoplasm becomes basophilic due to high RNA content for hemoglobin synthesis. (c) Polychromatic Erythroblasts Hemoglobin accumulation begins, giving the cytoplasm a mixed color (polychromatic appearance). The nucleus condenses further. (d) Orthochromatic Erythroblasts Final nucleated stage. Cytoplasm becomes eosinophilic due to high hemoglobin content. The nucleus becomes pyknotic (condensed) and is eventually extruded. d) Reticulocytes After nuclear extrusion, the cell becomes a reticulocyte. Reticulocytes are released into the bloodstream and complete their maturation (ribosomal RNA degradation) within 1–2 days. Key Features: Lack of a nucleus but retain residual organelles for continued hemoglobin synthesis. e) Mature Erythrocytes Reticulocytes mature into biconcave, flexible erythrocytes (RBCs) that lack organelles. The mature erythrocyte is optimized for gas exchange, carrying oxygen via hemoglobin and returning carbon dioxide to the lungs. Regulation of Erythropoiesis 1) Erythropoietin (EPO) Source: Produced mainly by the kidneys (90%) and, to a lesser extent, by the liver (10%). Stimulus: Hypoxia induces EPO synthesis via the hypoxia-inducible factor (HIF) pathway. Role: o Promotes survival and proliferation of CFU-E progenitors. o Enhances hemoglobin synthesis and accelerates the maturation of erythroid precursors. o Suppresses apoptosis in erythroid progenitors. 2) Iron Availability Essential Role: Iron is a critical component of hemoglobin. It is transported to the bone marrow by transferrin and stored intracellularly in ferritin. Deficiency: Iron deficiency impairs hemoglobin synthesis, leading to microcytic hypochromic anemia. 3) Hemoglobin Synthesis Key Molecules: o Globin chains (produced in the cytoplasm). o Heme group (synthesized in mitochondria from glycine and succinyl-CoA via the enzyme ALA synthase). Regulation: Coordinated production of globin and heme is vital for erythrocyte function. 4) Cytokines and Growth Factors Stem Cell Factor (SCF): Promotes HSC survival and differentiation. Interleukin-3 (IL-3): Stimulates early erythroid progenitors. Transforming Growth Factor-β (TGF-β): Inhibits erythropoiesis when levels are elevated, often in chronic inflammation. Molecular Mechanisms in Erythropoiesis a) Gene Regulation GATA1: Master regulator of erythroid differentiation, activates erythroid-specific genes. KLF1 (EKLF): Regulates β-globin gene expression and hemoglobin synthesis. FOG1 (Friend of GATA1): Co-factor for GATA1. b) Epigenetic Modifications Chromatin remodeling: Enhances the accessibility of erythroid-specific genes. DNA methylation and histone acetylation: Regulate transitions between progenitor stages. c) HIF Pathway Hypoxia-Inducible Factor (HIF): In response to low oxygen, HIF stabilizes and induces EPO transcription, promoting erythropoiesis. Relation to Conditions Causing Pallor: Pallor is the paleness of the skin and mucous membranes, often associated with an insufficient number of red blood cells (RBCs) or hemoglobin, which leads to reduced oxygen delivery to tissues. Hematopoiesis and pallor are related in the following ways: a) Anemia: o Anemia, a condition where there is a reduction in the number of red blood cells or hemoglobin, is a primary cause of pallor. Anemia can result from various causes: § Nutritional deficiencies (iron, vitamin B12, folate) § Bone marrow failure (due to diseases like aplastic anemia) § Blood loss (e.g., gastrointestinal bleeding, menstrual loss) § Hemolysis (destruction of red blood cells) § Chronic diseases (e.g., kidney disease, chronic inflammation) A failure of hematopoiesis in the bone marrow or disturbances in the development of blood cells in earlier stages (yolk sac, liver, spleen) can lead to anemia, which manifests as pallor due to reduced red blood cell count and oxygen-carrying capacity. b) Bone Marrow Disorders: o Conditions affecting bone marrow (e.g., leukemia, myelodysplastic syndromes) can impair the production of blood cells, including red blood cells, leading to anemia and pallor. c) Hemolytic Anemia: o Hemolysis, or the premature destruction of red blood cells, can cause anemia even if the bone marrow is producing red blood cells normally. Hemolytic anemia can be caused by genetic conditions, autoimmune diseases, infections, or certain medications. This results in reduced RBC count and subsequent pallor. d) Blood Loss: o Acute or chronic blood loss (e.g., from gastrointestinal bleeding, ulcers, trauma, or heavy menstruation) reduces the number of circulating red blood cells, leading to anemia and pallor. 5. What is the histological structure of the bone marrow, and how does it reflect blood production capacity? The bone marrow is a soft, spongy tissue located primarily in the medullary cavities of long bones (like the femur and humerus) and in the vertebrae, ribs, sternum, and pelvis in adults. It plays a crucial role in hematopoiesis, or the production of blood cells (red blood cells, white blood cells, and platelets). The histological structure of the bone marrow is specialized to support blood production. Here’s an overview of its structure and how it reflects the capacity for blood production: Two Types of Bone Marrow: Red Bone Marrow (Active Hematopoietic Marrow): This type is primarily involved in blood cell production. It contains a high number of hematopoietic stem cells, progenitor cells, and differentiated blood cells at various stages of development. Yellow Bone Marrow (Fatty Marrow): Found mostly in the shafts of long bones in adults, this marrow consists primarily of adipocytes (fat cells). It is less involved in hematopoiesis but can revert to red marrow in certain conditions, such as blood loss or anemia. Histological Structure: The bone marrow can be visualized under a microscope and has a highly organized structure, including the following key components: 1) Hematopoietic Cells: These are the cells responsible for the production of blood cells. They are arranged in cords or clusters and include: o Stem cells: Undifferentiated cells that can give rise to all blood cell types. o Progenitor cells: Intermediate cells that differentiate into specific blood cell types. o Mature blood cells: Cells at various stages of differentiation (e.g., erythrocytes, leukocytes, platelets). o Erythroid Cells: Clustered around macrophages in erythroblastic islands. Synthesize hemoglobin and mature into erythrocytes. o Myeloid Cells: Found in scattered groups; precursors of granulocytes and monocytes. o Megakaryocytes: Large cells located near sinusoids. Release platelets into the circulation by fragmentation of their cytoplasm. o Lymphoid Cells: Precursors of lymphocytes, distributed near sinusoids. 2) Stromal Cells: The bone marrow also contains non-hematopoietic cells that provide structural support. These cells form a stroma that includes: o Fibroblasts: Cells that produce extracellular matrix components, such as collagen and reticular fibers o Adipocytes: Fat cells, especially in yellow marrow, which may increase with age. Fill space in yellow marrow; provide energy and regulate hematopoiesis through cytokine secretion. o Endothelial cells: Line the blood vessels within the marrow and are involved in the trafficking of blood cells to and from the marrow. o Osteoblasts and Osteoclasts: Cells related to bone formation and resorption, respectively, which help maintain the bone marrow environment. 3) Sinusoids: These are specialized blood vessels within the marrow that allow for the exchange of cells and nutrients. The sinusoids have thin walls lined by endothelial cells, allowing newly formed blood cells (such as mature red blood cells, white blood cells, and platelets) to enter the bloodstream. This vascular network is essential for the release of mature blood cells into circulation. 4) Extracellular Matrix (ECM): The ECM provides a scaffold that supports hematopoiesis. It is composed of various proteins, including collagen, fibronectin, and laminin, which interact with hematopoietic stem cells and progenitor cells, influencing their growth and differentiation. Organization: Bone marrow tissue is typically organized into hematopoietic cords (also called marrow cords), which are surrounded by the vascular sinusoids. Within the cords, hematopoietic cells are arranged in a structured, progressive sequence based on their stage of differentiation. This organization reflects the continuous production and maturation of blood cells. Erythropoiesis (red blood cell production) is seen as clusters of developing erythroblasts, intermediate forms, and mature red blood cells. Leukopoiesis (white blood cell production) occurs in distinct regions of the marrow, with progenitors differentiating into granulocytes, monocytes, and lymphocytes. Megakaryopoiesis (platelet production) involves the differentiation of megakaryocytes, which extend cytoplasmic processes into the sinusoids to release platelets. Bone Marrow Microenvironment: The niche of hematopoietic stem cells within the bone marrow is highly specialized. The microenvironment regulates stem cell proliferation, differentiation, and survival. This is crucial for maintaining blood production capacity and responding to physiological demands, such as increased blood loss or infection. The marrow niche is influenced by growth factors, cytokines, and hormones (e.g., erythropoietin, granulocyte-colony stimulating factor [G-CSF], and thrombopoietin), which promote differentiation and maturation of blood cells. Reflection of Blood Production Capacity: The structure of the bone marrow is closely linked to its blood production capacity: High cell density and active hematopoiesis, seen in the red marrow, reflect a high rate of blood cell production. In this environment, hematopoietic stem cells are constantly renewing and differentiating into various blood cell types. Bone marrow failure or dysfunction, such as in aplastic anemia, results in a reduction in hematopoietic cells and can lead to hypoplastic marrow (with fewer blood cells) or fat infiltration (yellow marrow replacing red marrow). This reduces the capacity of the marrow to produce sufficient blood cells. Increased hematopoiesis can be seen in conditions such as myeloproliferative disorders, where the marrow produces excessive numbers of blood cells. 6. What are the physiological functions of hemoglobin, types of hemoglobin, hemoglobin synthesis, and how does its deficiency lead to pallor? Physiological Functions of Hemoglobin: Hemoglobin (Hb) is a protein found in red blood cells that plays a critical role in oxygen transport and carbon dioxide removal in the body. Its primary functions are: a) Oxygen Transport: o Hemoglobin binds to oxygen in the lungs, where oxygen concentration is high, and carries it through the bloodstream to the tissues and organs. Each hemoglobin molecule can carry up to four molecules of oxygen (one per heme group). o The oxygen-binding capacity of hemoglobin is influenced by the partial pressure of oxygen and the pH of the surrounding environment (Bohr effect), allowing it to pick up oxygen in the lungs and release it in tissues where oxygen is needed most. b) Carbon Dioxide Transport: o Hemoglobin also helps in the transport of carbon dioxide (CO₂), a byproduct of cellular metabolism. After oxygen is delivered to tissues, hemoglobin binds to some of the CO₂ and carries it back to the lungs, where it is expelled. About 20% of CO₂ is transported by hemoglobin in this way, while the rest is dissolved in plasma. c) Buffering Capacity: o Hemoglobin helps regulate the blood's pH by acting as a buffer. As hemoglobin binds to CO₂, it helps prevent large changes in the pH of the blood, contributing to maintaining acid-base balance. Types of Hemoglobin in Human Physiology Hemoglobin undergoes a developmental progression, with different types expressed during various stages of life to meet specific physiological needs. These hemoglobin types are distinguished by the globin chains that form their structure. Below is a detailed classification and discussion integrated into the context of hemoglobin synthesis: 1. Embryonic Hemoglobin (Produced during the first 8–12 weeks of gestation) Embryonic hemoglobin types are transient and primarily found in early fetal life. a) Hemoglobin Gower 1 (ζ₂ε₂) Globin Chains: Zeta (ζ) and epsilon (ε). Function: Provides oxygen to rapidly growing embryonic tissues. Expression: Found in yolk sac erythrocytes. b) Hemoglobin Gower 2 (α₂ε₂) Globin Chains: Alpha (α) and epsilon (ε). Transition: Gradually replaced by fetal hemoglobin as gestation progresses. c) Hemoglobin Portland (ζ₂γ₂) Globin Chains: Zeta (ζ) and gamma (γ). Rarity: Minor hemoglobin type, with little physiological significance in humans. 2. Fetal Hemoglobin (HbF, α₂γ₂) Globin Chains: Two alpha (α) and two gamma (γ) chains. Developmental Role: o Predominant hemoglobin from 12 weeks gestation to 6 months post-birth. o High oxygen affinity allows efficient oxygen transfer from maternal blood across the placenta. Persistence: o Low levels (3.5%) are seen in beta-thalassemia trait. c) Fetal Hemoglobin (HbF, α₂γ₂) Proportion: 14.5% As the body produces red blood cells under iron-deficient conditions, the red blood cells that are produced early in the process are smaller (microcytic), while some of the later cells may still be of normal size. This variation in cell size leads to an increased RDW. Clinical Significance: An elevated RDW is a significant indicator of iron deficiency anemia because it reflects the heterogeneity in the size of RBCs due to insufficient iron for consistent RBC production. RDW is often elevated early in iron deficiency, even before the MCV drops. Summary of Key CBC Findings in Iron Deficiency Anemia: 1. Low MCV (Microcytosis): o Indicates smaller-than-normal RBCs, characteristic of iron deficiency. 2. Low Hemoglobin: o Reflects the reduced capacity of the blood to carry oxygen due to insufficient hemoglobin production in the absence of adequate iron. 3. Elevated RDW: o Indicates variation in RBC size, caused by the presence of both smaller RBCs (early in iron deficiency) and normal-sized RBCs. Together, these CBC findings (low MCV, low hemoglobin, and elevated RDW) strongly support the diagnosis of iron deficiency anemia and help distinguish it from other types of anemia that may present with similar symptoms, such as anemia of chronic disease or thalassemia. What is the biochemical basis of microcytic hypochromic anemia? Microcytic hypochromic anemia refers to a type of anemia where the red blood cells (RBCs) are both smaller than normal (microcytic) and paler than normal (hypochromic). The biochemical basis for this condition involves impaired hemoglobin synthesis, particularly due to deficiencies in the components required for the production of hemoglobin. The most common cause of microcytic hypochromic anemia is iron deficiency, but it can also be caused by other conditions like thalassemia and sideroblastic anemia. Here is an overview of the biochemical mechanisms underlying this form of anemia: 1. Iron Deficiency and Impaired Hemoglobin Synthesis Hemoglobin (Hb) is the main protein in red blood cells responsible for oxygen transport. Hemoglobin is composed of heme (an iron-containing molecule) and globin (a protein chain). The iron component of heme is crucial for binding oxygen. Iron deficiency limits the production of heme, leading to reduced hemoglobin synthesis. Biochemical Impact of Iron Deficiency: o Reduced Heme Production: Iron is necessary for the synthesis of heme in the mitochondria of developing red blood cells. In the absence of adequate iron, the production of heme is impaired. This results in insufficient hemoglobin production, which causes the red blood cells to have less hemoglobin (hypochromic). o Microcytosis: As a compensatory mechanism, the bone marrow increases the production of red blood cells, but due to the lack of adequate hemoglobin synthesis, the RBCs remain smaller (microcytic). This is because the red blood cell precursors are unable to reach their normal size due to the limitation of hemoglobin content, which normally regulates cell size during maturation. o Cell Maturation Delays: Without enough iron for hemoglobin production, the maturation of RBCs is delayed, leading to the release of smaller, less mature RBCs into circulation. 2. Decreased Hemoglobin Synthesis in Thalassemia Thalassemia is a group of genetic disorders characterized by defects in the synthesis of the globin chains of hemoglobin (alpha or beta globin). o In alpha thalassemia, there is a deficiency or absence of alpha globin chains, leading to an imbalance in the production of hemoglobin. In beta thalassemia, beta globin chains are deficient or absent. o Biochemical Impact: § Ineffective Erythropoiesis: In both types of thalassemia, the lack of sufficient globin chains leads to the production of defective hemoglobin, which causes RBCs to be smaller (microcytic) and have less hemoglobin (hypochromic). § Increased Destruction of RBCs: The abnormal RBCs are less stable and prone to premature destruction, further contributing to anemia. 3. Sideroblastic Anemia Sideroblastic anemia is a type of anemia caused by defects in the mitochondrial enzymes involved in heme synthesis, resulting in impaired incorporation of iron into heme. o Biochemical Impact: § Iron Overload: In sideroblastic anemia, iron accumulates in the mitochondria of RBC precursors because it is not effectively incorporated into heme. This leads to the formation of sideroblasts (iron-loaded RBC precursors) in the bone marrow. § Microcytosis and Hypochromia: Similar to iron deficiency, the failure to produce adequate heme results in less hemoglobin in the RBCs, leading to microcytic hypochromic anemia. However, the underlying problem is not iron deficiency but a defect in heme synthesis despite the presence of iron. 4. Biochemical Mechanism of Microcytic Hypochromic Anemia In all these conditions, the central biochemical process is defective hemoglobin synthesis, either due to insufficient iron or defective globin chain production. Here are the key steps in the affected biochemical pathways: Iron Deficiency: o Iron is required for heme synthesis. Heme is synthesized from protoporphyrin and iron. When iron is insufficient, heme production is reduced, leading to a decrease in hemoglobin levels. o The bone marrow responds by producing more RBCs to compensate for the anemia, but without sufficient hemoglobin, the RBCs remain small and pale (microcytic and hypochromic). Globin Chain Defects (Thalassemia): o The defective globin chain synthesis leads to an imbalance in the production of alpha and beta globin chains. This results in the formation of unstable hemoglobin molecules or insufficient hemoglobin, causing microcytosis and hypochromia. Defective Heme Synthesis (Sideroblastic Anemia): o In sideroblastic anemia, the defect lies in the enzymes responsible for heme synthesis, preventing iron from being incorporated into the heme molecule. This results in the accumulation of iron and decreased hemoglobin production, leading to microcytic hypochromic anemia. Summary of Biochemical Basis of Microcytic Hypochromic Anemia: 1. Iron Deficiency: o Decreased iron for heme production → insufficient hemoglobin → small, pale RBCs (microcytic, hypochromic). 2. Thalassemia: o Defective globin chain production → imbalance in hemoglobin synthesis → small, hypochromic RBCs. 3. Sideroblastic Anemia: o Defective heme synthesis enzymes → impaired iron incorporation into heme → iron accumulation in mitochondria → small, hypochromic RBCs. How do tests like serum ferritin and TIBC provide insights into iron metabolism? Tests like serum ferritin and total iron-binding capacity (TIBC) provide valuable insights into iron metabolism, especially in the context of diagnosing iron deficiency anemia (IDA) and understanding the body’s iron stores and availability for red blood cell production. Here’s how these tests work and what they reveal about iron metabolism: 1. Serum Ferritin Ferritin is a protein that stores iron within cells, particularly in the liver, spleen, and bone marrow. The serum ferritin test measures the amount of ferritin in the blood, which reflects the body's iron stores. Normal Ferritin Levels: o Men: 30–300 ng/mL o Women: 20–150 ng/mL o These levels can vary slightly depending on the lab and individual factors. Role of Serum Ferritin in Iron Metabolism: Indicator of Iron Stores: Ferritin serves as the main form of iron storage in the body. When iron intake is low or iron is being used up (e.g., during blood loss), ferritin levels decrease, indicating depleted iron stores. Low Ferritin Levels: Low serum ferritin is typically one of the earliest indicators of iron deficiency, even before other iron-related tests (like serum iron) show abnormalities. A low ferritin level confirms iron deficiency anemia. Clinical Significance: In iron deficiency anemia, serum ferritin is low, reflecting depleted iron stores in the body. Conversely, in anemia of chronic disease (ACD), ferritin levels can be normal or elevated, even though iron utilization is impaired. This is because ferritin acts as an acute-phase reactant, and its levels can rise due to inflammation or infection, masking true iron deficiency. 2. Total Iron-Binding Capacity (TIBC) TIBC is a measure of the total amount of iron that can be bound by transferrin, the primary iron transport protein in the blood. It reflects the amount of available transferrin that can bind iron. Transferrin is produced by the liver, and its levels increase when iron stores are low, attempting to enhance iron transport. Normal TIBC: 250–450 µg/dL (varies by lab and method). High TIBC: High levels are typically seen in iron deficiency anemia, as the body increases the production of transferrin to maximize iron binding and transport when iron stores are low. Low TIBC: Low levels are often found in anemia of chronic disease or liver disease, where iron availability is restricted but transferrin production is decreased. Role of TIBC in Iron Metabolism: Reflects Iron Deficiency: When the body’s iron stores are low, transferrin levels rise to increase iron transport and absorption, leading to an increase in TIBC. This is a compensatory mechanism to ensure that available iron is carried to the bone marrow for red blood cell production. Increased TIBC in Iron Deficiency: In iron deficiency anemia, TIBC is elevated because there is a decreased iron supply, so the liver produces more transferrin to enhance iron binding and transport to the bone marrow. Clinical Significance: In iron deficiency anemia, both low ferritin and high TIBC are often found, suggesting that the body’s iron stores are depleted and the available transport capacity (transferrin) has increased to compensate for the lack of iron. 3. Transferrin Saturation Transferrin saturation is calculated from the serum iron and TIBC values. It reflects the percentage of transferrin that is bound to iron. This test gives a good indication of how much iron is being transported in the bloodstream compared to the total binding capacity. Transferrin Saturation = (Serum Iron / TIBC) × 100% Normal Transferrin Saturation: 20–50% Low Transferrin Saturation: < 20% (seen in iron deficiency anemia) High Transferrin Saturation: May be seen in iron overload conditions (e.g., hemochromatosis). 4. How Ferritin and TIBC Reflect Iron Metabolism The combination of serum ferritin and TIBC can provide a comprehensive picture of iron metabolism, particularly in distinguishing between different types of anemia: In Iron Deficiency Anemia (IDA): Low Ferritin: Indicates depleted iron stores, as ferritin is the main storage form of iron. High TIBC: Reflects the body's attempt to increase iron transport in response to low iron availability. These two findings together are strongly indicative of iron deficiency anemia. In Anemia of Chronic Disease (ACD): Normal or Elevated Ferritin: This occurs due to ferritin being an acute-phase reactant. In inflammation or chronic disease, ferritin levels may increase even when iron is being poorly utilized by the body. Low TIBC: TIBC is typically low because transferrin production decreases in response to chronic inflammation or infection, limiting the transport of iron. In Iron Overload Conditions (e.g., Hemochromatosis): High Ferritin: Indicates excess iron stores. Low TIBC: Since transferrin production decreases in response to excess iron, there is less available transferrin to bind and transport iron. 5. Summary of Key Insights into Iron Metabolism from Ferritin and TIBC Tests Serum Ferritin: o Low ferritin = depleted iron stores → iron deficiency anemia. o Normal or elevated ferritin in the context of anemia of chronic disease (due to inflammation). TIBC: o Elevated TIBC = iron deficiency → the body increases transferrin to transport more iron. o Decreased TIBC = chronic disease or iron overload → reduced transferrin production. Together, these tests offer a powerful method for diagnosing iron deficiency anemia and distinguishing it from other types of anemia, such as anemia of chronic disease, by assessing iron stores (ferritin) and iron transport capacity (TIBC). What additional tests can be performed to identify the underlying cause of anemia (e.g., iron studies, reticulocyte count, stool occult blood test)? To identify the underlying cause of anemia, additional tests can be performed, which can help differentiate between various types of anemia (e.g., iron deficiency anemia, anemia of chronic disease, hemolytic anemia, hemorrhagic anemia, etc.). The selection of these tests depends on the patient's clinical history, symptoms, and initial findings from routine blood tests like the CBC and iron studies. Here are some important tests that can help identify the cause of anemia: 1. Iron Studies (Iron Panel) Iron studies help assess whether iron deficiency is the cause of anemia and if there are issues with iron metabolism. Serum Iron: Measures the amount of circulating iron bound to transferrin. Low serum iron is seen in iron deficiency anemia. Total Iron-Binding Capacity (TIBC): Measures the total available transferrin for iron binding. It is often high in iron deficiency anemia and low in anemia of chronic disease. Transferrin Saturation: A calculated value (serum iron / TIBC × 100%) that reflects the percentage of transferrin bound to iron. Low transferrin saturation is seen in iron deficiency. Ferritin: Reflects iron stores in the body. Low ferritin indicates iron deficiency, while high ferritin suggests inflammation or iron overload. 2. Reticulocyte Count Reticulocytes are immature red blood cells released by the bone marrow into circulation. The reticulocyte count helps assess the bone marrow’s response to anemia and can help distinguish between different causes. Elevated Reticulocyte Count: An elevated reticulocyte count (reticulocytosis) suggests active red blood cell production, which may indicate acute blood loss, hemolysis, or recent blood loss (e.g., in gastrointestinal bleeding or trauma). Low Reticulocyte Count: A low count suggests ineffective erythropoiesis or bone marrow suppression, which can occur in conditions like anemia of chronic disease, iron deficiency anemia, or aplastic anemia. 3. Stool Occult Blood Test A stool occult blood test is used to detect hidden blood in the stool, which may indicate gastrointestinal bleeding as the underlying cause of anemia. This is particularly useful when: Iron deficiency anemia is suspected, but the source of blood loss is unclear. Chronic blood loss from ulcers, polyps, or malignancies (e.g., colorectal cancer) is suspected. The presence of occult blood in stool suggests that chronic gastrointestinal bleeding could be the cause of iron deficiency anemia, especially in older adults or those with a history of gastrointestinal issues. 4. Peripheral Blood Smear A peripheral blood smear provides detailed information about the morphology of red blood cells, which can help identify the cause of anemia. Microcytic, Hypochromic RBCs: Seen in iron deficiency anemia, thalassemia, and some forms of sideroblastic anemia. Macrocytic RBCs: Seen in vitamin B12 or folate deficiency, alcoholism, or myelodysplastic syndromes. Fragmented RBCs (schistocytes): Indicative of hemolytic anemia, often seen in conditions like microangiopathic hemolytic anemia or mechanical destruction (e.g., prosthetic heart valves). Sickle-shaped RBCs: Indicative of sickle cell anemia. 5. Bone Marrow Aspiration/Biopsy A bone marrow biopsy is a more invasive test and is typically performed when the cause of anemia is unclear after initial testing. It is used to assess the bone marrow’s ability to produce red blood cells and to look for abnormalities in the bone marrow cells. Aplastic Anemia: May show a hypocellular marrow (reduced cell production). Myelodysplastic Syndromes: May show abnormal cell development. Infiltration of Bone Marrow: Conditions like leukemia or lymphoma may be identified if the marrow is infiltrated by abnormal cells. 6. Hemolysis Markers If hemolytic anemia is suspected (where RBCs are prematurely destroyed), additional tests can be performed to assess the rate of red blood cell destruction. Serum Bilirubin (particularly indirect bilirubin): Elevated in hemolysis due to increased breakdown of heme. Lactate Dehydrogenase (LDH): Elevated in hemolysis, as LDH is released from destroyed RBCs. Haptoglobin: Decreased in hemolysis, as it binds to free hemoglobin released from lysed RBCs. Coombs Test (Direct Antiglobulin Test): Used to diagnose autoimmune hemolytic anemia (if antibodies are causing the destruction of RBCs). Hemoglobinuria: The presence of hemoglobin in the urine suggests hemolysis. 7. Vitamin B12 and Folate Levels Deficiency of vitamin B12 or folate can lead to megaloblastic anemia, which presents with macrocytic RBCs and is a common cause of fatigue and pallor. Testing these levels helps differentiate between microcytic and macrocytic anemias. Low Vitamin B12: Can result from pernicious anemia, malabsorption, or dietary deficiency. Low Folate: Can result from dietary deficiency, malabsorption, or increased demand (e.g., in pregnancy). 8. Tests for Chronic Disease or Inflammation If anemia of chronic disease (ACD) is suspected, additional tests can be done to investigate the underlying chronic condition. C-Reactive Protein (CRP) or Erythrocyte Sedimentation Rate (ESR): Elevated in inflammation or infection and may help suggest a chronic inflammatory disease or infection as the cause of anemia. Kidney Function Tests: Elevated levels of creatinine and urea nitrogen may indicate chronic kidney disease, which can lead to anemia due to decreased erythropoietin production. 9. Coagulation Studies If there is a suspicion of bleeding as a cause of anemia, coagulation tests such as prothrombin time (PT) and activated partial thromboplastin time (aPTT) can be done to evaluate bleeding tendencies. Prolonged PT and aPTT: May suggest bleeding disorders that could lead to anemia through blood loss. 10. Hemoglobin Electrophoresis Used to diagnose hemoglobinopathies (e.g., sickle cell disease, thalassemia). Sickle Cell Disease: Abnormal hemoglobin (HbS) can be identified through electrophoresis. Thalassemia: Abnormal hemoglobin patterns can be seen (e.g., increased HbF or HbA2). Summary of Tests for Identifying the Underlying Cause of Anemia: 1. Iron Studies (serum ferritin, TIBC, serum iron, transferrin saturation) for iron deficiency or iron overload. 2. Reticulocyte Count for assessing bone marrow response. 3. Stool Occult Blood Test for gastrointestinal bleeding. 4. Peripheral Blood Smear for RBC morphology. 5. Bone Marrow Aspiration/Biopsy for bone marrow disorders. 6. Hemolysis Markers (bilirubin, LDH, haptoglobin, Coombs test) for hemolytic anemia. 7. Vitamin B12 and Folate Levels for megaloblastic anemia. 8. Tests for Chronic Disease (CRP, ESR, kidney function tests) to detect underlying conditions. 9. Coagulation Studies for bleeding disorders. 10. Hemoglobin Electrophoresis for hemoglobinopathies. By combining these tests, healthcare providers can pinpoint the specific cause of anemia, enabling targeted treatment. How does the peripheral blood smear reflect red cell morphology and production defects? A peripheral blood smear is a crucial diagnostic tool that provides a detailed view of the morphology of red blood cells (RBCs) and can reveal abnormalities in red cell production, maturation, and destruction. It is particularly useful in identifying defects in RBCs that might lead to anemia and in differentiating between various types of anemia. Here’s how the findings on a peripheral blood smear reflect red cell morphology and production defects: Red Cell Morphology The peripheral blood smear allows the examiner to assess the shape, size, color, and presence of inclusions in red blood cells. These features provide clues to the underlying cause of anemia. a. Microcytic Hypochromic RBCs Appearance: RBCs are smaller than normal (microcytic) and have a pale center (hypochromic). Cause: This morphology is typically seen in iron deficiency anemia, thalassemia, and sideroblastic anemia. Production Defects: o In iron deficiency anemia, the bone marrow produces RBCs with insufficient hemoglobin, resulting in smaller (microcytic) and pale (hypochromic) cells due to lack of iron. o In thalassemia, defective hemoglobin synthesis leads to microcytic and hypochromic RBCs. b. Macrocytic RBCs Appearance: RBCs are larger than normal (macrocytic), and their color may still appear normal. Cause: Seen in megaloblastic anemia (due to vitamin B12 or folate deficiency), alcoholism, and liver disease. Production Defects: o In megaloblastic anemia, defective DNA synthesis (due to B12 or folate deficiency) leads to impaired maturation of RBC precursors in the bone marrow, resulting in large, immature RBCs being released into circulation. o Liver disease or alcoholism can lead to increased membrane lipid content in RBCs, causing macrocytosis. c. Normocytic RBCs Appearance: RBCs are normal-sized (normocytic), but their appearance can still provide clues. Cause: Seen in anemia of chronic disease, acute blood loss, and bone marrow failure. Production Defects: o In anemia of chronic disease, RBCs are typically normocytic but may be pale or slightly hypochromic due to impaired iron utilization and decreased erythropoiesis. o Acute blood loss can lead to normocytic anemia as the body responds by increasing production of new RBCs, which may not yet be fully matured. d. Poikilocytosis (Abnormal Shaped RBCs) Appearance: RBCs exhibit abnormal shapes, such as tear drop cells, spherocytes, ovalocytes, or fragmented cells. Cause: This is seen in a variety of conditions, including hemolytic anemias, sickle cell anemia, and thalassemia. Production Defects: o In sickle cell anemia, RBCs adopt a sickle shape due to a mutation in hemoglobin (HbS), leading to increased cell rigidity and premature destruction. o In thalassemia, RBCs can have a variety of abnormal shapes (e.g., target cells, ovalocytes) due to defective hemoglobin synthesis. o Fragmented cells (schistocytes) can be seen in microangiopathic hemolytic anemia (e.g., disseminated intravascular coagulation, thrombotic thrombocytopenic purpura) and mechanical destruction of RBCs. e. Target Cells (Codocytes) Appearance: RBCs have a central area of hemoglobin surrounded by a pale ring, resembling a target. Cause: This is often seen in thalassemia, liver disease, and iron deficiency anemia. Production Defects: o In thalassemia, abnormal hemoglobin production leads to a change in the RBC membrane that results in target cell formation. o Liver disease and iron deficiency anemia can also lead to target cells, due to changes in the lipid composition of the RBC membrane. Inclusions in Red Blood Cells The peripheral blood smear can also reveal inclusions within RBCs that reflect abnormalities in RBC production, iron metabolism, or infections. a. Howell-Jolly Bodies Appearance: Small, round, purple inclusions within RBCs. Cause: Splenectomy or hyposplenism, severe anemia, or megaloblastic anemia. Production Defects: Howell-Jolly bodies are remnants of the nucleus of immature RBCs that are normally removed by the spleen. Their presence suggests an impaired spleen function or a defective maturation process in the bone marrow. b. Basophilic Stippling Appearance: Small, blue dots scattered throughout the RBCs. Cause: Lead poisoning, thalassemia, and sideroblastic anemia. Production Defects: In lead poisoning, basophilic stippling occurs due to impaired heme synthesis. In thalassemia and sideroblastic anemia, it can result from abnormal hemoglobin synthesis or iron metabolism issues. c. Siderocytes (Pappenheimer Bodies) Appearance: Small, iron-containing granules in RBCs, typically visible with special staining. Cause: Sideroblastic anemia, thalassemia, or hemoglobinopathies. Production Defects: Siderocytes form when there is impaired incorporation of iron into heme, leading to iron accumulation in the RBCs. 3. Anisocytosis (Variation in RBC Size) Appearance: RBCs exhibit marked variation in size. Cause: Iron deficiency anemia, vitamin B12 or folate deficiency, hemolytic anemia, and bone marrow disorders. Production Defects: Anisocytosis occurs when the bone marrow releases RBCs of different sizes, often due to ineffective erythropoiesis or dysplastic changes in the bone marrow (as seen in megaloblastic anemia). 4. Nucleated Red Blood Cells (NRBCs) Appearance: RBCs with a nucleus. Cause: Severe anemia (e.g., acute blood loss, hemolysis) or bone marrow disorders. Production Defects: The presence of nucleated RBCs suggests premature release from the bone marrow into circulation, indicating bone marrow stress and an attempt to compensate for a severe shortage of RBCs. 5. Schistocytes (Fragmented RBCs) Appearance: Fragmented RBCs with jagged edges. Cause: Microangiopathic hemolytic anemia (e.g., thrombotic thrombocytopenic purpura, disseminated intravascular coagulation), mechanical heart valves, or severe trauma. Production Defects: Schistocytes are the result of mechanical damage or fragmentation due to abnormal shearing forces in the circulation, often seen in the microvasculature. 6. Sickle Cells Appearance: Crescent-shaped RBCs. Cause: Sickle cell anemia, a genetic disorder caused by the mutation in hemoglobin (HbS). Production Defects: The mutation in hemoglobin causes RBCs to become rigid and sickle-shaped, leading to blockage of small blood vessels, hemolysis, and chronic pain. Summary: How a Peripheral Blood Smear Reflects Red Cell Morphology and Production Defects Microcytic and hypochromic RBCs suggest iron deficiency anemia, thalassemia, or sideroblastic anemia. Macrocytic RBCs suggest megaloblastic anemia, liver disease, or alcoholism. Poikilocytosis and target cells suggest disorders like thalassemia, sickle cell disease, and hemolytic anemia. Inclusions like Howell-Jolly bodies and basophilic stippling indicate splenic dysfunction, lead poisoning, or sideroblastic anemia. Nucleated RBCs and schistocytes point to bone marrow stress and hemolytic anemia. By examining these findings, clinicians can pinpoint specific types of anemia and gain insights into production defects in the bone marrow or destruction of RBCs in the peripheral circulation, guiding further investigation and treatment. What are the differential diagnoses for iron deficiency anemia? When evaluating a patient for iron deficiency anemia (IDA), it’s crucial to consider several other conditions that can present with similar clinical features, such as fatigue, pallor, and decreased hemoglobin levels. The differential diagnosis includes other types of anemia as well as non-anemic conditions that can mimic iron deficiency symptoms. 1. Anemia of Chronic Disease (ACD) Cause: Associated with chronic infections, inflammation, or malignancy (e.g., rheumatoid arthritis, chronic kidney disease, cancer). Mechanism: In ACD, iron is sequestered in macrophages, and there is impaired erythropoiesis despite adequate iron stores. This results in low serum iron, low transferrin saturation, and normal to elevated ferritin (since ferritin is an acute-phase reactant). Distinguishing Features: o Ferritin is often normal or elevated in ACD, unlike in iron deficiency anemia (where ferritin is low). o The anemia in ACD is typically normocytic or microcytic and is usually mild or moderate. 2. Thalassemia (Alpha or Beta) Cause: A group of inherited disorders causing defective hemoglobin production, resulting in microcytic anemia. Mechanism: Thalassemia results in reduced or absent production of one of the globin chains (alpha or beta), leading to ineffective erythropoiesis and hemolysis. Distinguishing Features: o Peripheral blood smear typically shows target cells, basophilic stippling, and anisocytosis. o Hemoglobin electrophoresis can confirm the diagnosis by identifying abnormal hemoglobin patterns (e.g., increased HbA2 in beta-thalassemia). o Ferritin levels are typically normal or elevated in thalassemia, in contrast to iron deficiency anemia, where ferritin is low. 3. Sideroblastic Anemia Cause: A group of disorders where the bone marrow is unable to incorporate iron into hemoglobin, leading to iron accumulation in mitochondria and the formation of ringed sideroblasts. Mechanism: This can be either hereditary or acquired (e.g., due to lead poisoning, alcohol abuse, or drug- induced). Distinguishing Features: o Peripheral blood smear shows basophilic stippling, and bone marrow biopsy reveals ringed sideroblasts (iron-laden mitochondria surrounding the nucleus of RBC precursors). o Serum ferritin is often normal or elevated, while serum iron may be normal or increased, which contrasts with iron deficiency anemia (where ferritin is low). 4. Lead Poisoning Cause: Lead toxicity disrupts heme synthesis, leading to microcytic anemia. Mechanism: Lead inhibits ferrochelatase and ALA dehydratase, enzymes involved in heme synthesis, leading to the accumulation of protoporphyrin and iron in the blood. Distinguishing Features: o Basophilic stippling on the peripheral blood smear. o Elevated blood lead levels are diagnostic. o Ferritin levels can be normal or high, unlike in iron deficiency anemia, where ferritin is low. 5. Anemia Due to Blood Loss (Acute or Chronic) Cause: Chronic blood loss (e.g., gastrointestinal bleeding, menstrual bleeding) can lead to iron deficiency over time. Acute blood loss can cause a drop in hemoglobin, but it may not lead to iron deficiency immediately. Mechanism: In chronic blood loss, there is a gradual depletion of iron stores, leading to iron deficiency anemia. Distinguishing Features: o History of gastrointestinal bleeding (e.g., ulcers, malignancy, hemorrhoids) or heavy menstrual bleeding. o Stool occult blood test may detect gastrointestinal bleeding. o The peripheral blood smear will show signs of chronic blood loss such as anisocytosis, polychromasia, and a possible reticulocytosis (if the body is responding to blood loss). 6. Vitamin B12 or Folate Deficiency Cause: Deficiencies in vitamin B12 or folate can lead to megaloblastic anemia, which presents with fatigue and pallor similar to iron deficiency anemia. Mechanism: Impaired DNA synthesis leads to abnormal maturation of red blood cells (RBCs) in the bone marrow, resulting in larger-than-normal RBCs (macrocytic anemia). Distinguishing Features: o Peripheral blood smear shows macrocytic, oval-shaped RBCs, and hypersegmented neutrophils. o Serum B12 and folate levels are low in deficiency. o Iron studies may be normal or show a normal ferritin level. 7. Chronic Kidney Disease (Anemia of Renal Disease) Cause: Chronic kidney disease (CKD) can cause anemia due to decreased production of erythropoietin (EPO), which is needed for RBC production. Mechanism: The kidneys produce less erythropoietin, leading to reduced RBC production in the bone marrow. Distinguishing Features: o Normocytic or microcytic anemia with a low reticulocyte count. o Serum creatinine and blood urea nitrogen (BUN) levels are elevated in CKD. o Ferritin is usually normal or elevated, unlike in iron deficiency anemia where it is low. 8. Myelodysplastic Syndromes (MDS) Cause: A group of disorders caused by ineffective hematopoiesis, leading to defective red blood cell production. Mechanism: There is abnormal development of hematopoietic stem cells, leading to dysplastic RBCs in the bone marrow and peripheral blood. Distinguishing Features: o Peripheral blood smear shows dysplastic RBCs, including oval macrocytes, and anisocytosis. o Bone marrow biopsy may reveal hypercellularity with maturation arrest. 9. Hemolytic Anemia Cause: Conditions where RBCs are prematurely destroyed, leading to anemia. Common causes include autoimmune hemolytic anemia, sickle cell disease, hereditary spherocytosis, and G6PD deficiency. Mechanism: RBC destruction occurs prematurely, leading to the release of hemoglobin and bilirubin, and often reticulocytosis in response to the anemia. Distinguishing Features: o Peripheral blood smear may show spherocytes, schistocytes, or sickle cells, depending on the underlying cause. o Elevated reticulocyte count suggests bone marrow response to hemolysis. o Elevated indirect bilirubin and lactate dehydrogenase (LDH) levels. o Haptoglobin is usually low in hemolytic anemia. 10. Polycythemia Vera (PV) Cause: Polycythemia vera is a myeloproliferative disorder leading to increased red blood cell mass, which can present with a low red blood cell count due to hemodilution. Mechanism: Increased RBC production in the bone marrow leads to an increase in red blood cell mass and increased blood viscosity. Distinguishing Features: o Elevated hemoglobin or hematocrit (opposite of iron deficiency anemia). o JAK2 mutation is commonly present in PV. Summary of Differential Diagnoses for Iron Deficiency Anemia: 1. Anemia of Chronic Disease: Typically has normal/elevated ferritin and low serum iron/TIBC. 2. Thalassemia: Has microcytic, hypochromic RBCs with normal/elevated ferritin. 3. Sideroblastic Anemia: Has basophilic stippling and ringed sideroblasts on bone marrow biopsy. 4. Lead Poisoning: Shows basophilic stippling and elevated blood lead levels. 5. Blood Loss Anemia: Associated with chronic blood loss, may show a positive stool occult blood test. 6. B12/Folate Deficiency: Has macrocytic RBCs and hypersegmented neutrophils. 7. Anemia of Renal Disease: Normocytic anemia with elevated creatinine and BUN. 8. Myelodysplastic Syndromes: Shows dysplastic RBCs and hypercellular bone marrow. 9. Hemolytic Anemia: Has reticulocytosis, spherocytes, and elevated indirect bilirubin. 10. Polycythemia Vera: Presents with elevated hematocrit/hemoglobin and JAK2 mutation. How can thalassemia be distinguished from iron deficiency anemia using hematologic and biochemical tests? Thalassemia and iron deficiency anemia (IDA) can both present with microcytic hypochromic anemia, but they have distinct underlying causes and can be distinguished through hematologic and biochemical tests. Here’s a breakdown of how these two conditions can be differentiated: 1. Red Blood Cell (RBC) Morphology on Peripheral Blood Smear Thalassemia: o Target cells: RBCs with a central area of hemoglobin surrounded by a pale ring, characteristic of thalassemia. o Basophilic stippling: Presence of small blue dots inside RBCs, indicating abnormal hemoglobin production. o Anisocytosis and poikilocytosis: Variation in RBC size and shape, often with more pronounced irregular shapes than in iron deficiency anemia. o Nucleated RBCs: May be seen in severe cases. Iron Deficiency Anemia: o Microcytic hypochromic RBCs: RBCs are smaller (microcytic) and have a pale center (hypochromic), reflecting the reduced hemoglobin content due to lack of iron. o Poikilocytosis: Some variation in RBC shape, but typically not as extreme as in thalassemia. o Target cells: May occasionally appear but are not as common or prominent as in thalassemia. 2. Mean Corpuscular Volume (MCV) Thalassemia: o Low MCV (often