PA 603 Remediation Topics PDF
Document Details
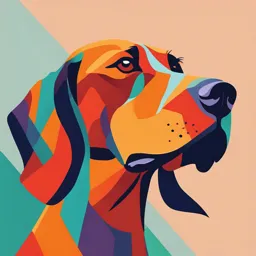
Uploaded by LustrousSerenity839
Saint Elizabeth University
Tags
Summary
This document is a past paper containing remediation topics in physiology, covering the action potential, different muscle types, and muscle cell excitation-contraction coupling. It includes multiple-choice questions and a presentation component.
Full Transcript
PA 603-Remediation (Two Components totaling 100 points each) Student must achieve an average of 80 or better on each of the following two assessments in order to pass the remediation. 1. Physiology Multiple Choice Exam -60 questions- 100 points (60 minutes timeframe) â—Ź Student may bring in 1 page (...
PA 603-Remediation (Two Components totaling 100 points each) Student must achieve an average of 80 or better on each of the following two assessments in order to pass the remediation. 1. Physiology Multiple Choice Exam -60 questions- 100 points (60 minutes timeframe) ● Student may bring in 1 page (8.5”x11”) front & back of notes 2. “ I Get It Presentation”- requiring between 8-10 points - 10 minute oral presentation ● Based on any system in which student had lowest exam grade ● Student’s choice of any physiologic topic related to that particular system ● See attached rubric (Same assessment utilized during semester so students are familiar with criteria) Physiology Systems: Cellular Physiology ● Describe the changes in ionic channels that underlie the action potential Resting State: o At rest, a neuron has a negative charge inside compared to the outside, with a potential difference across its membrane. o Voltage-gated sodium (Na+) channels are closed, and some potassium (K+) channels are open, maintaining this resting membrane potential. ● Depolarization Phase: o When a stimulus is received, the membrane potential becomes less negative (depolarized). o Voltage-gated sodium channels open, allowing an influx of sodium ions into the neuron. o The rapid influx of positive sodium ions causes a sharp rise in membrane potential, creating the depolarization phase. ● Threshold and Action Potential: o If the depolarization reaches a certain threshold, it triggers the opening of more voltage-gated sodium channels. o This positive feedback loop results in a rapid and large influx of sodium ions, causing a spike in membrane potential known as the action potential. ● Repolarization Phase: o After reaching its peak, the voltage-gated sodium channels begin to close. o Voltage-gated potassium channels open, allowing potassium ions to move out of the neuron, contributing to repolarization. o The efflux of positive ions (potassium) restores the negative charge inside the neuron, bringing the membrane potential back towards its resting state. ● Hyperpolarization Phase: o In some neurons, there is a brief hyperpolarization phase where the membrane potential becomes more negative than the resting state. o This occurs because potassium channels remain open for a short period after repolarization. ● Sodium-Potassium Pump: o The sodium-potassium pump actively transports sodium ions out of the neuron and potassium ions back in, restoring the ionic balance and preparing the neuron for another action potential. ● Differentiate the major classes of muscle in the body Skeletal Muscle: o Structure: Skeletal muscles are attached to bones by tendons and are responsible for the voluntary movement of the body. o Function: They enable body movements such as walking, talking, and grasping objects. o Control: Skeletal muscles are under conscious control, meaning they respond to signals from the somatic nervous system. They contract in response to signals from the brain. ● Smooth Muscle: o Structure: Smooth muscles are found in the walls of internal organs, blood vessels, and structures like the digestive tract. o Function: They are responsible for involuntary, rhythmic contractions, helping to propel substances through various internal body systems, such as food through the digestive tract. o Control: Smooth muscles are primarily under the control of the autonomic nervous system, and their contractions are often not consciously controlled. ● Cardiac Muscle: o Structure: Cardiac muscles make up the heart. o Function: They contract rhythmically to pump blood throughout the body, providing the circulatory function. o Control: Cardiac muscle contractions are involuntary and are regulated by the autonomic nervous system. However, the cardiac muscle has some unique properties that allow it to generate its own rhythmic contractions. ● Describe the molecular and electrical makeup of muscle cell excitation–contraction coupling Neuromuscular Junction (NMJ): o The process begins when a motor neuron releases acetylcholine (ACh) at the neuromuscular junction, a specialized synapse between the motor neuron and the muscle fiber. ● Action Potential Propagation: o The released ACh binds to receptors on the muscle cell membrane, known as the sarcolemma, leading to depolarization of the membrane. o This depolarization generates an action potential that spreads along the sarcolemma and into the muscle fiber through structures called T-tubules (transverse tubules). ● Calcium Release from Sarcoplasmic Reticulum (SR): o The action potential travels deep into the muscle fiber via T-tubules, stimulating the sarcoplasmic reticulum (SR), an internal membrane system in muscle cells. o The SR releases stored calcium ions (Ca2+) into the cytoplasm of the muscle cell. ● Troponin-Tropomyosin Complex and Actin-Myosin Interaction: o Calcium binds to troponin, a regulatory protein associated with the thin filaments of muscle cells. o This binding causes a conformational change in the troponin-tropomyosin complex, exposing binding sites on the actin filaments. ● Cross-Bridge Formation and Contraction: o Myosin heads (from the thick filaments) bind to the exposed sites on actin, forming cross-bridges. o ATP (adenosine triphosphate) is hydrolyzed, providing energy for the myosin heads to pull the actin filaments toward the center of the sarcomere. o This repeated formation and breaking of cross-bridges result in muscle contraction. ● Calcium Removal and Muscle Relaxation: o When the action potential ceases, calcium is actively pumped back into the SR by a calcium pump, reducing the cytoplasmic calcium concentration. o As calcium dissociates from troponin, the troponin-tropomyosin complex covers the binding sites on actin, preventing further cross-bridge formation and leading to muscle relaxation. ● Define elements of the sarcomere that underlie striated muscle contraction Actin Filaments: o Actin is a thin filament composed of globular actin (G-actin) monomers. o Actin filaments are anchored to the Z-discs and extend towards the center of the sarcomere. ● Myosin Filaments: o Myosin is a thick filament composed of myosin molecules. o Myosin filaments are located in the center of the sarcomere and overlap with actin filaments. ● Z-Disc (Z-Line): o The Z-disc is a protein structure that bisects the sarcomere. o It serves as the attachment point for the thin actin filaments and helps define the boundaries of the sarcomere. ● I-Band (Isotropic Band): o The I-band is a region of the sarcomere that contains only thin actin filaments. o It extends from the Z-disc towards the center of the sarcomere. ● A-Band (Anisotropic Band): o The A-band is the central region of the sarcomere where thick myosin filaments are present. o It includes both overlapping actin and myosin filaments. ● H-Zone: o The H-zone is a region within the A-band where only myosin filaments are present, and there is no overlap with actin. o It is located at the center of the A-band. ● M-Line: o The M-line is a protein structure at the center of the H-zone that helps stabilize and align the myosin filaments. ● Titin: o Titin is a giant protein that spans from the Z-disc to the M-line, providing structural support and elasticity to the sarcomere. ● Describe the main morphologic features of synapses ​ Presynaptic Terminal: ● The axon terminal of the presynaptic neuron contains synaptic vesicles filled with neurotransmitters. ● These vesicles are membrane-bound structures that store and release neurotransmitters into the synaptic cleft. ​ Synaptic Cleft: ● The synaptic cleft is a small fluid-filled gap that separates the presynaptic and postsynaptic membranes. ● It serves as a physical barrier that neurotransmitters must cross to transmit the signal from the presynaptic to the postsynaptic neuron or target cell. ​ Postsynaptic Membrane: ● The membrane on the receiving side of the synapse is called the postsynaptic membrane. ● It contains specialized receptors that bind to neurotransmitters released by the presynaptic neuron. ● The postsynaptic membrane may be part of another neuron, a muscle cell, or a gland cell, depending on the type of synapse. ​ Synaptic Vesicles: ● These small, membrane-bound vesicles within the presynaptic terminal store neurotransmitters. ● When an action potential reaches the presynaptic terminal, it triggers the release of neurotransmitters into the synaptic cleft. ​ Neurotransmitters: ● These chemical messengers are released from synaptic vesicles in response to an action potential. ● Neurotransmitters diffuse across the synaptic cleft and bind to receptors on the postsynaptic membrane, initiating a response in the postsynaptic cell. ​ Synaptic Endbulbs or Boutons: ● The terminal endings of the presynaptic axon often form specialized structures called synaptic endbulbs or boutons. ● These structures contain the synaptic vesicles and are in close proximity to the postsynaptic membrane. ​ Mitochondria: ● Synapses are energy-demanding structures, and mitochondria are often present in both the presynaptic and postsynaptic regions to provide the necessary energy for neurotransmitter release and other cellular processes. ​ Synaptic Density: ● The number of synapses in a given area, known as synaptic density, varies throughout the nervous system and is particularly high in regions involved in complex processing and learning. ​ ● Distinguish between chemical and electrical transmissions at synapses ● Chemical Synapses: o Presynaptic Terminal: This is the end of the axon of the presynaptic neuron. It contains synaptic vesicles filled with neurotransmitters. o Synaptic Cleft: The small gap between the presynaptic terminal and the postsynaptic membrane. Neurotransmitters are released into this cleft. o Postsynaptic Membrane: The membrane of the target cell (often another neuron or a muscle cell) that faces the synaptic cleft. It contains receptors for neurotransmitters. o Synaptic Vesicles: Small membrane-bound sacs within the presynaptic terminal that store neurotransmitters. o Neurotransmitters: Chemical messengers released from synaptic vesicles into the synaptic cleft. They bind to receptors on the postsynaptic membrane. ● Electrical Synapses: o Gap Junctions: Electrical synapses are characterized by gap junctions, which are clusters of channels that directly connect the cytoplasm of adjacent cells. o Connexons: These are the protein complexes that form gap junction channels. Each connexon is made up of six connexin proteins. o Cytoplasmic Continuity: Electrical synapses allow the direct flow of ions and other small molecules between the cytoplasm of connected cells, enabling rapid electrical communication. ● Describe the neuromuscular junction, and explain how action potentials in the motor neuron at the junction lead to contraction of the skeletal muscle Neuromuscular Junction: ​ Motor Neuron Axon Terminal: ● The process begins with a motor neuron, specifically its axon terminal, which approaches the muscle fiber. ​ Synaptic Cleft: ● The motor neuron's axon terminal is separated from the muscle fiber by a small gap called the synaptic cleft. ​ Motor End Plate: ● The muscle fiber's membrane at the synaptic cleft is known as the motor end plate. It contains receptors for acetylcholine (ACh), a neurotransmitter. ​ Action Potential Initiation: ● When an action potential reaches the axon terminal of the motor neuron, it triggers the opening of voltage-gated calcium channels. ​ Calcium Influx: ● The influx of calcium into the motor neuron's axon terminal promotes the fusion of synaptic vesicles containing acetylcholine with the neuron's cell membrane. ​ Release of Acetylcholine: ● Acetylcholine is released into the synaptic cleft from the synaptic vesicles. ​ Acetylcholine Binding: ● Acetylcholine binds to receptors on the motor end plate, causing a temporary change in the permeability of the muscle cell membrane to sodium ions. Action Potential Propagation in the Muscle Fiber: ● End Plate Potential (EPP): o The binding of acetylcholine generates an end plate potential (EPP) in the muscle fiber. This is a local depolarization of the motor end plate. ● Depolarization of Muscle Fiber: o If the EPP is of sufficient magnitude, it triggers an action potential that spreads across the muscle fiber's membrane and into its interior through T-tubules (transverse tubules). ● Calcium Release from Sarcoplasmic Reticulum (SR): o The action potential in the T-tubules signals the release of calcium ions from the sarcoplasmic reticulum (SR), an internal membrane system in the muscle cell. ● Contraction: o Calcium ions bind to troponin, initiating a series of events that expose binding sites on actin filaments. o Myosin heads bind to actin, forming cross-bridges, and muscle contraction occurs as the thin actin filaments slide over the thick myosin filaments. ● Termination of Signal: o Acetylcholine is quickly broken down by acetylcholinesterase in the synaptic cleft, terminating the signal. ● Relaxation: o Calcium is actively pumped back into the SR, leading to the relaxation of the muscle fiber. ● List the major types of neurotransmitters (ex. acetylcholine) ● Acetylcholine (ACh): o Function: Involved in muscle contraction, learning, and memory. o Location: Found in the neuromuscular junction and various areas of the brain. ● Dopamine: o Function: Regulates mood, reward, and pleasure; involved in motor control. o Location: Found in areas of the brain related to reward and motor function. ● Serotonin: o Function: Regulates mood, appetite, and sleep; contributes to the feeling of well-being. o Location: Primarily found in the gastrointestinal tract and the central nervous system. ● Gamma-Aminobutyric Acid (GABA): o Function: Inhibitory neurotransmitter, regulates anxiety and stress; contributes to motor control. o Location: Found throughout the central nervous system. ● Glutamate: o Function: Excitatory neurotransmitter, involved in learning and memory. o Location: Abundant in the central nervous system, especially in the brain. ● Norepinephrine (Noradrenaline): o Function: Modulates attention, alertness, and mood; involved in the "fight or flight" response. o Location: Found in the brain and sympathetic nervous system. ● Epinephrine (Adrenaline): o Function: Involved in the "fight or flight" response; increases heart rate and prepares the body for intense physical activity. o Location: Released by the adrenal glands in response to stress. ● Histamine: o Function: Involved in the immune response, regulation of stomach acid, and neurotransmission in the brain. o Location: Found in the central nervous system and mast cells. ● Oxytocin: o Function: Regulates social bonding, trust, and maternal behavior. o Location: Produced in the hypothalamus and released by the pituitary gland. ● Endorphins: o Function: Act as natural painkillers and produce feelings of euphoria. o Location: Released in response to stress and pain. ● Summarize the steps involved in the biosynthesis, release, action, and removal from the synaptic cleft of the major neurotransmitters Acetylcholine (ACh): ● Biosynthesis: Synthesized from choline and acetyl coenzyme A (acetyl-CoA) in the presynaptic neuron. ● Release: Action potentials in the motor neuron lead to the release of ACh into the synaptic cleft at the neuromuscular junction. ● Action: ACh binds to receptors on the motor end plate of the muscle fiber, leading to depolarization and muscle contraction. ● Removal: Acetylcholinesterase breaks down ACh into choline and acetate in the synaptic cleft. 2. Dopamine: ● Biosynthesis: Synthesized from the amino acid tyrosine. ● Release: Action potentials in dopaminergic neurons lead to the release of dopamine into the synaptic cleft. ● Action: Dopamine binds to receptors on the postsynaptic neuron, modulating mood, reward, and motor control. ● Removal: Reuptake pumps on the presynaptic neuron reabsorb dopamine, or it may be broken down by enzymes like monoamine oxidase (MAO). 3. Serotonin: ● Biosynthesis: Synthesized from the amino acid tryptophan. ● Release: Action potentials in serotonergic neurons lead to the release of serotonin into the synaptic cleft. ● Action: Serotonin binds to receptors on the postsynaptic neuron, influencing mood, appetite, and sleep. ● Removal: Reuptake pumps on the presynaptic neuron reabsorb serotonin. 4. Gamma-Aminobutyric Acid (GABA): ● Biosynthesis: Synthesized from glutamate. ● Release: Action potentials in GABAergic neurons lead to the release of GABA into the synaptic cleft. ● Action: GABA binds to receptors on the postsynaptic neuron, causing inhibitory effects, preventing excessive neural activity. ● Removal: Reuptake pumps on the presynaptic neuron reabsorb GABA, or it may be broken down by the enzyme GABA transaminase. 5. Glutamate: ● Biosynthesis: Synthesized from the amino acid glutamine. ● Release: Action potentials in glutamatergic neurons lead to the release of glutamate into the synaptic cleft. ● Action: Glutamate binds to receptors on the postsynaptic neuron, causing excitatory effects and facilitating neural transmission. ● Removal: Reuptake pumps on the presynaptic neuron reabsorb glutamate, or it may be converted to glutamine by glial cells. ● Describe the process of muscle contractility ● Neuromuscular Junction Activation: o The process begins with the arrival of an action potential at the neuromuscular junction, where a motor neuron connects with a muscle fiber. ● Release of Acetylcholine (ACh): o The action potential in the motor neuron triggers the release of acetylcholine into the synaptic cleft at the neuromuscular junction. ● Binding of ACh to Receptors: o Acetylcholine binds to receptors on the motor end plate of the muscle fiber, leading to a change in the membrane potential of the muscle cell. ● Generation of Action Potential in Muscle Fiber: o The binding of acetylcholine generates an end-plate potential (EPP) in the muscle fiber, which, if it reaches the threshold, triggers an action potential along the sarcolemma (muscle cell membrane). ● Propagation of Action Potential: o The action potential travels along the sarcolemma and penetrates into the muscle fiber through T-tubules (transverse tubules), ensuring the signal reaches the interior of the muscle cell. ● Release of Calcium from Sarcoplasmic Reticulum (SR): o The action potential in the T-tubules signals the release of calcium ions (Ca2+) from the sarcoplasmic reticulum (SR), an internal membrane system in the muscle cell. ● Binding of Calcium to Troponin: o Calcium ions bind to troponin, a regulatory protein associated with the actin filaments in the sarcomere. ● Exposure of Myosin Binding Sites on Actin: o The binding of calcium to troponin causes a conformational change, exposing myosin-binding sites on the actin filaments. ● Cross-Bridge Formation: o Myosin heads (from the thick filaments) bind to the exposed sites on actin, forming cross-bridges. ● Sliding of Actin and Myosin Filaments: o ATP (adenosine triphosphate) is hydrolyzed, providing energy for the myosin heads to pull the actin filaments toward the center of the sarcomere. ● Muscle Contraction: o The repeated formation and breaking of cross-bridges result in the sliding of actin over myosin filaments, causing the sarcomere to shorten and the muscle to contract. ● Termination of Contraction: o The action potential ceases, and calcium ions are actively pumped back into the SR, leading to the relaxation of the muscle fiber. ● Explain the physiologic changes that occur during and after aerobic and anaerobic exercise Aerobic Exercise: During Exercise: ​ Increased Oxygen Consumption: ● Aerobic exercise relies on oxygen for energy production. As exercise intensity increases, there is a rise in oxygen consumption to support oxidative phosphorylation in the mitochondria. ​ Aerobic Metabolism: ● The primary energy sources are carbohydrates and fats. These macronutrients undergo aerobic metabolism to produce ATP, water, and carbon dioxide. ​ Increased Heart Rate and Stroke Volume: ● To meet the oxygen demands of the muscles, the heart rate and stroke volume increase. This enhances cardiac output, ensuring that oxygen-rich blood is delivered to working muscles. ​ Respiratory Rate and Oxygen Delivery: ● Respiratory rate and depth increase to facilitate the exchange of oxygen and carbon dioxide in the lungs. This helps in oxygen delivery to tissues and removal of metabolic byproducts. ​ Sustained Energy Output: ● Aerobic exercise is characterized by sustained, moderate-intensity efforts. It is conducive to activities like running, swimming, and cycling. After Exercise: ​ Recovery Period: ● Following aerobic exercise, there is a brief recovery period during which heart rate, breathing rate, and metabolic processes gradually return to baseline. ​ EPOC (Excess Post-exercise Oxygen Consumption): ● EPOC represents the oxygen consumed post-exercise to restore the body to its pre-exercise state. It helps repay the oxygen debt incurred during intense aerobic activity. ​ Replenishment of Energy Stores: ● Carbohydrate stores (glycogen) are replenished, and metabolic processes shift back toward a balanced state. The body also utilizes fatty acids for ongoing energy needs. Anaerobic Exercise: During Exercise: ​ Limited Oxygen Availability: ● Anaerobic exercise involves high-intensity, short-duration efforts where oxygen demand exceeds supply. Energy is derived without the need for oxygen. ​ Anaerobic Glycolysis: ● Glycolysis, the breakdown of glucose, is the primary energy pathway. This occurs without oxygen and produces lactate as a byproduct. ​ ATP from Phosphocreatine: ● For very short bursts of activity, phosphocreatine is rapidly broken down to regenerate ATP, providing quick energy for activities like sprinting and weightlifting. ​ Lactic Acid Production: ● Lactic acid is produced when pyruvate is converted to lactate during anaerobic glycolysis. This can contribute to muscle fatigue. After Exercise: ​ Lactate Clearance: ● During the post-exercise period, lactate is gradually cleared from the muscles and converted back to pyruvate, which can be used for energy or converted to glucose. ​ Replenishment of ATP and Phosphocreatine: ● ATP and phosphocreatine stores are replenished during the recovery phase, utilizing oxygen-dependent processes. ​ Restoration of pH Balance: ● The body works to restore the pH balance disrupted by the accumulation of lactic acid during anaerobic activity. ​ Post-Exercise Oxygen Consumption (EPOC): ● While EPOC is less pronounced after anaerobic exercise compared to aerobic exercise, there is still an elevated metabolic rate during the recovery phase. ● Compare and contrast skeletal muscle to cardiac and smooth muscle in reference to physiologic anatomy, cellular and molecular organization, neuromuscular junction, production of action potentials and excitation-contraction coupling Physiologic Anatomy: Skeletal Muscle: ● Location: Attached to bones via tendons, forming the skeletal system. ● Appearance: Striated (striped) appearance under the microscope. ● Voluntary Control: Under conscious control for voluntary movements. Cardiac Muscle: ● Location: Forms the heart walls. ● Appearance: Striated appearance similar to skeletal muscle but with intercalated discs. ● Involuntary Control: Involuntary contraction controlled by the autonomic nervous system. Smooth Muscle: ● Location: Found in the walls of internal organs, blood vessels, and structures like the digestive tract. ● Appearance: Non-striated, with a spindle-shaped morphology. ● Involuntary Control: Under involuntary control, regulated by the autonomic nervous system. Cellular and Molecular Organization: Skeletal Muscle: ● Muscle Fiber Structure: Long, multinucleated fibers. ● Myofibrils: Highly organized units containing sarcomeres. ● Control of Contraction: Controlled by somatic motor neurons. Cardiac Muscle: ● Cardiomyocyte Structure: Branched cells with a single nucleus. ● Intercalated Discs: Specialized cell-to-cell junctions that allow coordinated contraction. ● Control of Contraction: Involuntary contraction regulated by the autonomic nervous system. Smooth Muscle: ● Cell Structure: Spindle-shaped cells with a single nucleus. ● No Sarcomeres: Lack the highly organized sarcomere structure. ● Control of Contraction: Involuntary contraction regulated by the autonomic nervous system and hormones. Neuromuscular Junction: Skeletal Muscle: ● Neuromuscular Junction: Present at the motor end plate of each muscle fiber. ● Neurotransmitter: Acetylcholine is the neurotransmitter. ● Control: Voluntary control with conscious input. Cardiac Muscle: ● Neuromuscular Junction: Connections are less defined than in skeletal muscle. ● Neurotransmitter: Acetylcholine is released but has a modulatory effect; norepinephrine is the primary neurotransmitter. ● Control: Involuntary control regulated by the autonomic nervous system. Smooth Muscle: ● Neuromuscular Junction: Less defined than in skeletal muscle. ● Neurotransmitter: Varied neurotransmitters, including acetylcholine and norepinephrine. ● Control: Involuntary control regulated by the autonomic nervous system and hormones. Production of Action Potentials: Skeletal Muscle: ● Action Potentials: Produced by somatic motor neurons. ● Initiation: Stimulated by voluntary neural input. Cardiac Muscle: ● Action Potentials: Generated spontaneously and can be modulated by the autonomic nervous system. ● Initiation: Autorhythmic; originates in the pacemaker cells of the heart. Smooth Muscle: ● Action Potentials: Generated spontaneously and modulated by the autonomic nervous system and hormones. ● Initiation: May be initiated by pacemaker cells or external stimuli. Excitation-Contraction Coupling: Skeletal Muscle: ● Mechanism: Calcium-induced calcium release. ● Regulation: Troponin-tropomyosin complex regulates the exposure of myosin-binding sites on actin. Cardiac Muscle: ● Mechanism: Calcium-induced calcium release. ● Regulation: Similar to skeletal muscle but with a longer duration of contraction and a role for extracellular calcium. Smooth Muscle: ● Mechanism: Calcium-calmodulin-dependent myosin light chain kinase. ● Regulation: Myosin light chain phosphorylation regulates the interaction of myosin with actin. ● Describe the process of contraction of smooth muscle and the influence of nervous and hormonal inputs Process of Contraction of Smooth Muscle: ​ Calcium Entry: ● The process begins with an increase in intracellular calcium concentration. This can occur through the entry of extracellular calcium through channels in the cell membrane or through the release of calcium from intracellular stores, such as the sarcoplasmic reticulum. ​ Calmodulin Activation: ● Calcium binds to calmodulin, a regulatory protein in smooth muscle cells. ​ Activation of Myosin Light Chain Kinase (MLCK): ● The calcium-calmodulin complex activates myosin light chain kinase (MLCK), an enzyme responsible for phosphorylating the myosin light chain. ​ Phosphorylation of Myosin: ● MLCK phosphorylates the myosin light chain, leading to a conformational change in the myosin molecule. ​ Formation of Cross-Bridges: ● The phosphorylated myosin binds to actin, forming cross-bridges between the thick and thin filaments. ​ Power Stroke and Muscle Contraction: ● The power stroke occurs as the cross-bridges pull the actin filaments, resulting in muscle contraction. This process is ATP-dependent. ​ Myosin Light Chain Dephosphorylation: ● Myosin light chain phosphatase (MLCP) facilitates the dephosphorylation of the myosin light chain, leading to muscle relaxation. Influence of Nervous Inputs: ​ Autonomic Nervous System: ● Smooth muscle is innervated by the autonomic nervous system, consisting of sympathetic and parasympathetic divisions. ● Sympathetic Stimulation: Norepinephrine released from sympathetic nerve terminals binds to adrenergic receptors, leading to an increase in intracellular calcium, MLCK activation, and muscle contraction. ● Parasympathetic Stimulation: Acetylcholine released from parasympathetic nerve terminals binds to muscarinic receptors, influencing calcium levels and MLCK activity, leading to muscle contraction or relaxation depending on the organ and receptor subtype. ​ Enteric Nervous System: ● The enteric nervous system, a division of the autonomic nervous system local to the gastrointestinal tract, also plays a significant role in regulating smooth muscle contraction. Influence of Hormonal Inputs: ​ Hormones: ● Various hormones can modulate smooth muscle contraction. ● Epinephrine and Norepinephrine: Released from the adrenal medulla, these hormones can stimulate or inhibit smooth muscle contraction depending on the receptor subtype and the tissue involved. ● Angiotensin II: A vasoconstrictor that influences smooth muscle contraction in blood vessels. ​ Local Factors: ● Nitric Oxide (NO): Released by endothelial cells, NO acts as a vasodilator and relaxes smooth muscle in blood vessels. ● Prostaglandins: Can have both relaxing and contracting effects on smooth muscle depending on the context and specific receptors involved. ● Describe pathophysiology of action potential conduction- myelination vs unmyelinated-impairing nervous system Myelinated Nerve Fibers: ​ Normal Conduction: ● In myelinated nerve fibers, the action potential "jumps" from one node of Ranvier to the next, a process known as saltatory conduction. ● The myelin sheath acts as an insulator, preventing the dissipation of electrical charge and allowing for rapid propagation of the action potential. ​ Impairment - Demyelination: ● Diseases or conditions that lead to demyelination, such as multiple sclerosis (MS), disrupt the myelin sheath. ● Without the insulating effect of myelin, the action potential conduction becomes slower, and there is a higher likelihood of signal loss and dispersion. ​ Consequences: ● Slowed conduction can lead to symptoms such as weakness, sensory deficits, and impaired coordination. ● Demyelination also makes nerve fibers more vulnerable to fatigue. Unmyelinated Nerve Fibers: ​ Normal Conduction: ● In unmyelinated nerve fibers, the action potential travels continuously along the entire length of the axon. ● Conduction is slower compared to myelinated fibers. ​ Impairment - Axonal Damage: ● Conditions that damage the axon itself, such as peripheral neuropathies, can impair action potential conduction in unmyelinated fibers. ● Axonal damage disrupts the integrity of the nerve fiber and compromises the ability to transmit signals effectively. ​ Consequences: ● Impaired conduction in unmyelinated fibers can result in symptoms such as numbness, tingling, and pain. ● Axonal damage may lead to a loss of sensory or motor function. General Implications for the Nervous System: ​ Signal Transmission: ● Impaired conduction in either myelinated or unmyelinated fibers can result in the failure of signal transmission between neurons. ​ Motor and Sensory Dysfunction: ● Disruptions in action potential conduction can lead to motor dysfunction, sensory deficits, and other neurological symptoms. ​ Neuropathic Pain: ● Impaired conduction and nerve damage may contribute to neuropathic pain, characterized by abnormal sensations and pain signals. ​ Loss of Function: ● Depending on the location and extent of impairment, the loss of function can be localized or affect broader regions of the nervous system. ● Describe pathophysiology of neuromuscular junction- Myasthenia Gravis, toxins/drugs affecting neuromuscular transmission, Clostridium tetani Myasthenia Gravis: Pathophysiology: ​ Autoimmune Disorder: ● Myasthenia Gravis (MG) is an autoimmune disorder where the immune system mistakenly attacks and destroys acetylcholine receptors (AChRs) at the neuromuscular junction. ​ Reduced AChR Density: ● Antibodies, mainly targeting the nicotinic AChRs, bind to and block these receptors. This results in a decrease in the effective density of AChRs on the postsynaptic membrane. ​ Impaired Signal Transmission: ● With fewer available receptors, the binding of acetylcholine (ACh) released by the motor neuron is less effective. This leads to impaired signal transmission and muscle weakness. ​ Fatigue and Fluctuating Symptoms: ● As the muscle is repeatedly stimulated, fatigue sets in, and symptoms worsen. MG often presents with fluctuating muscle weakness, particularly in muscles that control eye and facial movements, chewing, and swallowing. Toxins/Drugs Affecting Neuromuscular Transmission: Botulinum Toxin: ​ Source: ● Produced by Clostridium botulinum bacteria. ​ Mechanism: ● Inhibits the release of ACh at the neuromuscular junction by blocking the fusion of synaptic vesicles with the cell membrane, preventing the release of neurotransmitter. ​ Effect: ● Causes muscle paralysis by reducing the ability of motor neurons to stimulate muscle contraction. ● Used therapeutically in small, controlled doses for cosmetic and medical purposes, such as treating muscle spasms. Curare-Like Drugs: ​ Source: ● Various plants and tree bark. ​ Mechanism: ● Blocks nicotinic ACh receptors on the postsynaptic membrane, preventing ACh from binding and initiating muscle contraction. ​ Effect: ● Induces muscle paralysis and is used during surgical procedures to facilitate intubation and muscle relaxation. Clostridium Tetani (Tetanus Toxin): Pathophysiology: ​ Toxin Production: ● Clostridium tetani, a bacterium found in soil and dust, produces tetanus toxin. ​ Toxin Action: ● Tetanus toxin enters the body through wounds or cuts. Once inside, it blocks the release of inhibitory neurotransmitters (glycine and gamma-aminobutyric acid or GABA) in the spinal cord. ​ Unopposed Excitation: ● With the inhibition of inhibitory signals, there is unopposed excitation of motor neurons, leading to excessive muscle contractions and spasms. ​ Characteristics: ● Tetanus is characterized by sustained and painful muscle contractions, often starting in the jaw (lockjaw) and spreading to other parts of the body. ​ Prevention: ● Tetanus vaccination is a crucial preventive measure. Blood/Immunology ● Describe the genesis of red blood cells, white blood cell and platelets beginning with the pluripotent hematopoietic stem cell and ending with the mature cell in the lineage; B2.02b (What are the formed elements in blood? ex. RBC’s, WBC’s etc.) Pluripotent Hematopoietic Stem Cell (HSC): ● Origin: Located in the bone marrow. ● Characteristics: These cells have the potential to differentiate into various blood cell lineages. 2. Common Myeloid Progenitor (CMP) and Common Lymphoid Progenitor (CLP): ● Differentiation: ● Pluripotent HSCs give rise to common myeloid progenitors (CMP) and common lymphoid progenitors (CLP). ● CMPs give rise to cells of the myeloid lineage, including erythrocytes, platelets, and various types of white blood cells. ● CLPs give rise to cells of the lymphoid lineage, including lymphocytes. 3. Formation of Red Blood Cells (Erythropoiesis): 3.1. Proerythroblast: ● Differentiation from CMP leads to the formation of proerythroblasts. ● Proerythroblasts are committed to the erythroid lineage. 3.2. Erythroblast Stages: ● Proerythroblasts progress through several stages, including basophilic, polychromatophilic, and orthochromatic erythroblasts. 3.3. Reticulocyte: ● The final stage of erythropoiesis is the reticulocyte, which still contains residual organelles. ● Reticulocytes are released into the bloodstream. 3.4. Mature Red Blood Cell (Erythrocyte): ● Reticulocytes lose their remaining organelles and become mature red blood cells (erythrocytes). ● Erythrocytes are biconcave discs that lack a nucleus, allowing for efficient oxygen transport. 4. Formation of White Blood Cells (Myelopoiesis): 4.1. Myeloblast: ● Differentiation from CMP leads to the formation of myeloblasts. ● Myeloblasts are committed to the myeloid lineage. 4.2. Granulocyte Stages: ● Myeloblasts progress through stages, including promyelocytes, myelocytes, metamyelocytes, and band cells. ● Depending on the specific lineage, these cells differentiate into neutrophils, eosinophils, or basophils. 4.3. Monocyte Formation: ● Monocytes, another type of white blood cell, arise from monoblasts. 5. Formation of Platelets (Megakaryopoiesis): 5.1. Megakaryoblast: ● Differentiation from CMP leads to the formation of megakaryoblasts. ● Megakaryoblasts are committed to the megakaryocytic lineage. 5.2. Megakaryocyte: ● Megakaryoblasts undergo endomitosis (replication without division) to form large, multinucleated megakaryocytes. 5.3. Platelet Budding: ● Megakaryocytes extend projections (proplatelets) into sinusoidal blood vessels in the bone marrow. ● Platelets bud off from the proplatelets. 5.4. Circulating Platelets: ● Released platelets circulate in the bloodstream, playing a crucial role in blood clotting. Summary (Formed Elements in Blood): ● Red Blood Cells (Erythrocytes): ● Originate from proerythroblasts and mature into reticulocytes and erythrocytes. ● White Blood Cells (Leukocytes): ● Neutrophils, eosinophils, basophils, lymphocytes, and monocytes originate from myeloblasts, lymphoid progenitors, and monoblasts, respectively. ● Platelets (Thrombocytes): ● Derived from megakaryocytes through platelet budding. ● Explain the factors, which raise and lower red blood cell production and WBC production; B2.02b (Leukocytosis vs thrombocytopenia, and polycythemia- hematocrit results ) Factors Influencing Red Blood Cell (RBC) Production: 1. Erythropoietin (EPO): ● Stimulates RBC Production: EPO, released by the kidneys in response to low oxygen levels in the blood, stimulates the production of red blood cells in the bone marrow. 2. Oxygen Levels: ● Hypoxia Induces EPO Release: Low oxygen levels (hypoxia) in tissues and organs stimulate the release of EPO, promoting erythropoiesis. 3. Iron Availability: ● Essential for Hemoglobin Synthesis: Adequate iron levels are essential for hemoglobin synthesis. Iron deficiency can lead to decreased RBC production. 4. Vitamin B12 and Folate: ● Essential for DNA Synthesis: Vitamin B12 and folate are crucial for DNA synthesis during RBC maturation. Deficiencies can result in impaired RBC production. 5. Bone Marrow Health: ● Critical for Erythropoiesis: Healthy bone marrow is essential for the production of red blood cells. Factors Influencing White Blood Cell (WBC) Production: 1. Colony-Stimulating Factors (CSFs): ● Stimulate WBC Production: CSFs, such as granulocyte colony-stimulating factor (G-CSF) and granulocyte-macrophage colony-stimulating factor (GM-CSF), stimulate the production of white blood cells. 2. Infection or Inflammation: ● Leukocytosis: In response to infection or inflammation, the body can increase the production of white blood cells to combat pathogens. 3. Corticosteroids: ● Regulation of WBC Production: Corticosteroids can influence the production and release of white blood cells from the bone marrow. 4. Leukopenia Causes: ● Bone Marrow Disorders: Conditions affecting the bone marrow, such as leukemia or myelodysplastic syndromes, can lead to decreased WBC production. Conditions Related to Blood Cell Counts: 1. Leukocytosis: ● Definition: Elevated white blood cell count (WBC). ● Causes: Infection, inflammation, leukemia, and other conditions can lead to leukocytosis. 2. Thrombocytopenia: ● Definition: Low platelet count. ● Causes: Bone marrow disorders, immune system disorders, medications, or excessive destruction of platelets can result in thrombocytopenia. 3. Polycythemia: ● Definition: Elevated red blood cell count. ● Causes: Polycythemia vera (a myeloproliferative disorder), chronic hypoxia, or certain kidney diseases can lead to polycythemia. 4. Hematocrit: ● Definition: The proportion of blood that is cellular (primarily red blood cells). ● Normal Range: Typically expressed as a percentage, with normal values varying by age and sex. ● Polycythemia: Elevated hematocrit is associated with polycythemia. ● Anemia: Low hematocrit may indicate anemia. Clinical Implications: ● Leukocytosis: Often indicative of an immune response to infection or inflammation. ● Thrombocytopenia: Increases the risk of bleeding and impaired blood clotting. ● Polycythemia: May result in increased blood viscosity, potentially leading to circulatory issues. ● Discuss the steps involved in platelet plug formation, blood coagulation and clot retraction; B2.02b (What promotes hemostasis during platelet activation and prevents hemostasis at site of injury?) Hemostasis: Hemostasis is the process that prevents and stops bleeding. It involves three main steps: vascular spasm, platelet plug formation, and blood coagulation. 1. Vascular Spasm: ● Constriction of Blood Vessels: ● In response to injury, blood vessels constrict (vasospasm) to reduce blood flow and minimize blood loss. 2. Platelet Plug Formation (Primary Hemostasis): a. Adhesion: ● Platelets adhere to the exposed collagen fibers at the site of injury. ● Von Willebrand factor (vWF): Released by damaged endothelial cells, it helps in platelet adhesion. b. Release Reaction: ● Adhered platelets release granules containing substances like ADP and thromboxane A2. c. Aggregation: ● Platelets become activated and stick together (aggregate) through fibrinogen bridges. ● Forms a temporary "platelet plug" that covers the breach in the vessel wall. 3. Blood Coagulation (Secondary Hemostasis): ● Intrinsic and Extrinsic Pathways: ● Injured tissues release tissue factor, initiating the extrinsic pathway. ● The intrinsic pathway is activated by platelets and exposed collagen. ● Both pathways converge at Factor X, leading to the common pathway. a. Formation of Thrombin: ● Factor X combines with other factors to form thrombin. b. Conversion of Fibrinogen to Fibrin: ● Thrombin converts soluble fibrinogen into insoluble fibrin threads. c. Formation of the Clot: ● Fibrin threads form a mesh that traps blood cells, creating a stable clot. 4. Clot Retraction and Repair: a. Clot Retraction: ● Platelets contract, pulling torn areas of the blood vessel together. ● Reduces the size of the clot. b. Clot Repair: ● Platelets release growth factors that stimulate healing and repair of the blood vessel. Regulation of Hemostasis: Positive Feedback: ● Platelet activation releases substances that attract more platelets to the site, amplifying the response. Negative Feedback: ● Inhibitory factors prevent excessive clot formation to avoid vessel occlusion. Anticoagulants: 1. Antithrombin: ● Inactivates thrombin. 2. Protein C and S: ● Inactivate factors Va and VIIIa. 3. Heparin: ● Enhances antithrombin activity. Summary: ● Platelet Plug Formation: Adhesion, release reaction, and aggregation lead to the formation of a temporary platelet plug. ● Blood Coagulation: Intrinsic and extrinsic pathways converge to form thrombin, which converts fibrinogen to fibrin, creating a stable clot. ● Clot Retraction and Repair: Platelets contract, reducing the clot size, and release growth factors for vessel repair. ● Regulation: Positive and negative feedback mechanisms balance hemostasis. ● Anticoagulants: Inhibit various steps in the coagulation cascade to prevent excessive clot formation. Promotion and Prevention of Hemostasis: ● Promotion during Platelet Activation: ● Positive feedback amplifies platelet activation at the site of injury. ● Released substances attract more platelets. ● Prevention at the Site of Injury: ● Anticoagulants and negative feedback mechanisms prevent excessive clot formation and avoid vessel occlusion. ● Define the circulating and tissue cell types that contribute immune and inflammatory responses; B2.02b (ex. cells involved with seasonal allergies, mast cells, B-cell lines/antibodies) Circulating Immune Cells: ​ White Blood Cells (Leukocytes): ● Neutrophils: ● Highly phagocytic cells that are among the first responders to bacterial infections. ● Monocytes: ● Circulate in the blood and differentiate into macrophages when they enter tissues, playing a role in phagocytosis. ● Lymphocytes: ● T Cells: ● Play a central role in cell-mediated immunity, recognizing and destroying infected or abnormal cells. ● B Cells: ● Differentiate into plasma cells, producing antibodies that target specific pathogens. ● Natural Killer (NK) Cells: ● Recognize and destroy infected or cancerous cells without prior sensitization. ​ Eosinophils: ● Involved in the immune response to parasitic infections and allergic reactions. ● Release substances to combat parasites and modulate the immune response. ​ Basophils and Mast Cells: ● Release histamine and other mediators involved in allergic reactions and inflammatory responses. Tissue-Resident Immune Cells: ​ Macrophages: ● Found in tissues, macrophages are highly phagocytic cells that play a key role in innate immunity and antigen presentation to T cells. ​ Dendritic Cells: ● Present in tissues and lymphoid organs, dendritic cells capture and process antigens, presenting them to T cells to initiate adaptive immune responses. ​ Mast Cells: ● Found in connective tissues and mucous membranes, mast cells release histamine and other inflammatory mediators in response to allergens or pathogens. ​ Resident Memory T Cells: ● T cells that reside in tissues and provide rapid response upon re-exposure to previously encountered pathogens. Other Cell Types Involved in Inflammatory Responses: ​ Fibroblasts: ● Involved in tissue repair and the formation of scar tissue following inflammation. ​ Endothelial Cells: ● Line blood vessels and play a role in immune responses by facilitating the recruitment of immune cells to sites of infection or injury. Seasonal Allergies: ​ Mast Cells and Basophils: ● Release histamine and other mediators in response to allergens, triggering allergic reactions. ● Eosinophils may also be involved in the response to allergens. ​ T Cells and B Cells: ● T cells may play a role in modulating the immune response in allergic reactions. ● B cells can produce antibodies, contributing to the allergic response. B-Cell Lines and Antibodies: ​ B Cells: ● Differentiate into plasma cells, which produce antibodies. ● Antibodies (immunoglobulins) neutralize pathogens, activate complement, and facilitate phagocytosis. ​ Antibodies: ● Proteins produced by B cells that recognize and bind to specific antigens. ● Play a crucial role in adaptive immunity by marking pathogens for destruction. ● Explain the basis of inflammatory responses and wound healing; B2.02b Inflammatory Responses: **1. Initiation: ● Inflammation is triggered by factors such as infection, tissue damage, or the presence of harmful substances. **2. Vasodilation and Increased Permeability: ● Blood vessels dilate to increase blood flow to the affected area. ● Increased permeability allows immune cells to exit the bloodstream and reach the site of injury or infection. **3. Chemotaxis: ● Immune cells are attracted to the site by chemotactic signals released by damaged cells, pathogens, or other immune cells. **4. Phagocytosis: ● Phagocytes (neutrophils and macrophages) engulf and digest pathogens, debris, and damaged cells. **5. Release of Inflammatory Mediators: ● Mast cells release histamine, leading to vasodilation and increased permeability. ● Cytokines and chemokines coordinate the inflammatory response and attract immune cells. **6. Resolution: ● Anti-inflammatory signals help resolve the inflammatory response once the threat is eliminated. ● Damaged tissue undergoes repair and regeneration. Wound Healing: **1. Inflammation (Early Phase): ● Inflammatory cells clear debris and pathogens from the wound. ● Growth factors stimulate the migration of cells involved in tissue repair. **2. Proliferation (Intermediate Phase): ● Fibroblasts produce collagen, creating a provisional matrix. ● Angiogenesis (new blood vessel formation) provides nutrients and oxygen to support tissue growth. ● Epithelial cells migrate across the wound to close the gap. **3. Remodeling (Late Phase): ● Collagen is remodeled and reorganized to enhance tissue strength. ● Excess cells undergo apoptosis (programmed cell death). ● The scar tissue matures, and the wound heals. **4. Growth Factors: ● Platelet-derived growth factor (PDGF), transforming growth factor-beta (TGF-β), and other growth factors stimulate cell proliferation and tissue repair. **5. Angiogenesis: ● New blood vessels form to provide nutrients, oxygen, and immune cells to the healing tissue. **6. Epithelialization: ● Epithelial cells at the wound edges proliferate and migrate to cover the wound, forming a new surface layer. **7. Collagen Synthesis: ● Fibroblasts synthesize collagen, providing structural support to the healing tissue. **8. Contraction: ● Myofibroblasts contribute to wound contraction, reducing the wound size. **9. Scar Formation: ● Collagen fibers are gradually replaced by a more organized matrix, forming scar tissue. Key Players in Inflammatory Responses and Wound Healing: **1. Neutrophils and Macrophages: ● Phagocytic cells that clear debris and pathogens during inflammation. ● Macrophages also contribute to tissue repair. **2. Fibroblasts: ● Produce collagen and contribute to tissue remodeling and repair. **3. Platelets: ● Release growth factors that stimulate wound healing. **4. Growth Factors: ● Regulate cell proliferation, migration, and differentiation during wound healing. **5. Epithelial Cells: ● Contribute to wound closure through migration and proliferation. **6. Myofibroblasts: ● Assist in wound contraction. Balancing Inflammation and Resolution: ● Timely Resolution: ● Failure to resolve inflammation can lead to chronic inflammatory conditions. ● Anti-inflammatory signals help bring the inflammatory response to an end. Nervous ● Discuss the organization and function of the nervous system in the human body; B2.02b (Describe types of intracranial hemorrhages, brain tumors aggressive vs non-aggressive, structure of brain responsible for long term memory) 1. Central Nervous System (CNS): ● Consists of the brain and spinal cord. ● Responsible for processing and integrating information, as well as generating responses. 2. Peripheral Nervous System (PNS): ● Comprises nerves outside the CNS, including sensory and motor neurons. ● Connects the CNS to the rest of the body. 3. Neurons: ● Basic structural and functional units of the nervous system. ● Transmit electrical signals (action potentials) to communicate information. 4. Glial Cells: ● Support and protect neurons. ● Include astrocytes, oligodendrocytes, microglia, and Schwann cells. 5. Divisions of the Peripheral Nervous System: ● Somatic Nervous System (SNS): Controls voluntary muscle movements. ● Autonomic Nervous System (ANS): Regulates involuntary functions (e.g., heart rate, digestion). 6. Autonomic Nervous System Divisions: ● Sympathetic Nervous System: Activates the "fight or flight" response. ● Parasympathetic Nervous System: Promotes the "rest and digest" response. Types of Intracranial Hemorrhages: 1. Subarachnoid Hemorrhage (SAH): ● Bleeding into the space between the brain and the arachnoid membrane. ● Often caused by a ruptured aneurysm. 2. Intracerebral Hemorrhage (ICH): ● Bleeding within the brain tissue itself. ● Can result from trauma, hypertension, or underlying vascular abnormalities. 3. Epidural Hematoma: ● Accumulation of blood between the skull and the outermost layer of the brain (dura mater). ● Typically associated with head trauma. 4. Subdural Hematoma: ● Blood collects between the dura mater and the arachnoid membrane. ● May be caused by head injury or tearing of blood vessels. Brain Tumors: Aggressive vs. Non-aggressive: 1. Aggressive Brain Tumors: ● Glioblastoma Multiforme (GBM): ● Highly malignant and fast-growing. ● Infiltrates surrounding brain tissue. ● Challenging to treat and often associated with a poor prognosis. 2. Non-aggressive Brain Tumors: ● Meningioma: ● Typically slow-growing. ● Arises from the meninges (membranes covering the brain). ● Generally considered non-malignant. Structure of the Brain Responsible for Long-Term Memory: 1. Hippocampus: ● Location: Located in the medial temporal lobe. ● Function: Crucial for the formation of new memories, especially declarative and episodic memories. ● Role in Long-Term Memory: Converts short-term memories into long-term memories. 2. Amygdala: ● Location: Adjacent to the hippocampus in the temporal lobe. ● Function: Involved in the processing of emotions and emotional memories. ● Role in Long-Term Memory: Enhances the consolidation of emotionally significant memories. 3. Cerebral Cortex: ● Location: Outer layer of the brain. ● Function: Responsible for higher cognitive functions, including memory. ● Role in Long-Term Memory: Different regions of the cerebral cortex are involved in various aspects of long-term memory storage and retrieval. ● Describe how the nervous system communicates information in the body; B2.02b (Describe types of glia cells & function) 1. Neurons: ● Function: Transmit electrical impulses (action potentials) for communication. ● Structure: ● Cell Body (Soma): Contains the nucleus and organelles. ● Dendrites: Branch-like extensions that receive signals from other neurons. ● Axon: Long, slender extension that transmits signals away from the cell body. ● Axon Terminals: Release neurotransmitters to communicate with other cells. 2. Action Potential: ● Neurons generate electrical impulses known as action potentials. ● Action potentials travel along the axon to transmit signals. 3. Synapse: ● The junction between two neurons or between a neuron and a target cell. ● Neurotransmitters released from the axon terminals transmit signals across the synapse. 4. Neurotransmitters: ● Chemical messengers released by neurons to transmit signals to other neurons, muscle cells, or glands. 5. Receptor Cells: ● Receive neurotransmitters on the surface of target cells. ● Initiate a response in the target cell. 6. Electrical and Chemical Signal Transmission: ● Electrical signals (action potentials) within neurons. ● Chemical signals (neurotransmitters) between neurons and at synapses. Types of Glial Cells and Functions: 1. Astrocytes: ● Function: ● Provide physical and metabolic support to neurons. ● Regulate the concentration of ions and neurotransmitters in the extracellular space. ● Form the blood-brain barrier, restricting the passage of substances between the blood and brain. 2. Oligodendrocytes (in CNS) and Schwann Cells (in PNS): ● Function: ● Produce myelin, a fatty substance that wraps around axons. ● Myelin sheaths insulate axons, speeding up the conduction of action potentials. 3. Microglia: ● Function: ● Act as the immune cells of the CNS. ● Remove debris, damaged cells, and pathogens through phagocytosis. ● Contribute to the inflammatory response in the brain. 4. Ependymal Cells: ● Function: ● Line the ventricles of the brain and central canal of the spinal cord. ● Produce cerebrospinal fluid (CSF), which provides buoyancy and protection for the brain and spinal cord. 5. Satellite Cells (PNS): ● Function: ● Surround neurons in peripheral ganglia. ● Provide structural support and regulate the exchange of nutrients and waste. 6. Enteric Glial Cells: ● Function: ● Found in the enteric nervous system of the digestive tract. ● Support neurons and modulate digestive function. 7. Radial Glia (During Development): ● Function: ● Serve as guides for migrating neurons during embryonic development. Glial Cell Interactions with Neurons: ● Metabolic Support: ● Astrocytes provide neurons with nutrients and help regulate ion concentrations. ● Myelination: ● Oligodendrocytes in the CNS and Schwann cells in the PNS produce myelin to insulate axons. ● Immune Response: ● Microglia participate in immune responses within the CNS. ● Cerebrospinal Fluid Production: ● Ependymal cells contribute to the production of cerebrospinal fluid. ● List the component parts of the central nervous system and describe their function in the processing of afferent and efferent neural transmission; B2.02b (Describe lower motor neuron versus upper motor neuron disease presentation/symptoms) ​ Brain: ● Function: ● Coordinates sensory information. ● Regulates motor activities. ● Supports cognitive functions such as thinking, memory, and emotions. ​ Spinal Cord: ● Function: ● Facilitates communication between the brain and peripheral nerves. ● Mediates reflexes. ● Acts as a conduit for ascending (sensory) and descending (motor) signals. Afferent (Sensory) and Efferent (Motor) Neural Transmission: 1. Afferent (Sensory) Neural Transmission: ● Process: ● Sensory receptors detect stimuli (e.g., touch, temperature, pain). ● Sensory neurons transmit signals from receptors to the spinal cord or brain. ● Ascending pathways carry sensory information to higher brain centers. ● Function: ● Perceive and process sensory information. ● Contribute to conscious awareness and reflex responses. 2. Efferent (Motor) Neural Transmission: ● Process: ● Motor neurons transmit signals from the brain or spinal cord to muscles or glands. ● Descending pathways carry motor commands to lower motor neurons. ● Motor commands result in muscle contraction or glandular secretion. ● Function: ● Initiate and control voluntary movements. ● Regulate autonomic functions. Lower Motor Neuron vs. Upper Motor Neuron Disease: 1. Lower Motor Neuron (LMN) Disease: ● Definition: ● Affects motor neurons located in the spinal cord or peripheral nerves. ● Symptoms: ● Muscle weakness or atrophy. ● Fasciculations (twitching) in affected muscles. ● Hyporeflexia (reduced reflexes). ● Fibrillations (spontaneous muscle contractions). ● Muscle cramps. ● Examples: ● Amyotrophic lateral sclerosis (ALS). ● Poliomyelitis. ● Spinal muscular atrophy. 2. Upper Motor Neuron (UMN) Disease: ● Definition: ● Affects motor neurons located in the brain or descending pathways. ● Symptoms: ● Spasticity (increased muscle tone). ● Hyperreflexia (exaggerated reflexes). ● Clonus (rhythmic muscle contractions). ● Babinski sign (abnormal reflex of the big toe). ● Weakness or paralysis, usually on one side of the body. ● Examples: ● Stroke (cerebrovascular accident). ● Multiple sclerosis. ● Traumatic brain injury. Distinctive Features: 1. Lower Motor Neuron (LMN) Lesions: ● Location of Lesion: ● Located at or below the level of the spinal cord. ● Clinical Feat