OLM MCB.10 DNA Replication Notes PDF
Document Details
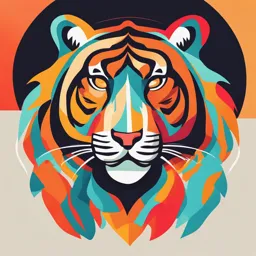
Uploaded by AccommodativeDesert1563
American University of the Caribbean, School of Medicine
Zsolt Fábián
Tags
Related
- Chapter 4 Part I DNA Biology & Cell Division PDF
- Molecular Biology of The Cell: Chapter 5 - DNA Replication, Repair, and Recombination PDF
- DNA and the Molecular Structure of Chromosomes PDF
- BIOL 360 - Module 2 - DNA Replication.pdf
- Introduction to Genetics, DNA replication and protein synthesis.pdf
- DNA Replication.pdf
Summary
DNA replication notes. The document details prokaryotic and eukaryotic DNA replication processes, including initiation, elongation, and termination.
Full Transcript
DNA replication Zsolt Fábián M.D., Ph.D., Dr. Habil. 1 DNA replication Lesson 1 – Prokaryotic DNA replication Zsolt Fábián M.D., Ph.D., Dr. Habil. 2 ...
DNA replication Zsolt Fábián M.D., Ph.D., Dr. Habil. 1 DNA replication Lesson 1 – Prokaryotic DNA replication Zsolt Fábián M.D., Ph.D., Dr. Habil. 2 Prokaryotic DNA replication General features of DNA replication Craig et al., Molecular Biology ‐ Principles of Genome Function, 2e, Oxford University Press, (2014) Before cells divide, DNA replication must occur DNA replication is the complete, faithful copying of the DNA comprising the cell’s chromosomes 3 Prokaryotic DNA replication DNA polymerase III Craig et al., Molecular Biology ‐ Principles of Genome Function, 2e, Oxford University Press, (2014) Cells have number of different polymerases – some for genome replication, some for repair and other functions like telomere replication The main polymerases involved in DNA replication are DNA polymerase III in bacteria and DNA polymerases δ and ε in eukaryotes These polymerases are highly conserved and are composed of multiple subunits – the different subunits have different functions, like proofreading DNA polymerases remain attached to DNA for long stretches before dissociation – this is the processivity of a polymerase DNA polymerases move along the DNA template strand, adding complementary bases and forming a new strand of DNA DNA polymerases catalyze the addition of the nucleotide to the 3′ OH of the last nucleotide in the newly growing strand Accuracy is essential – in addition to adding the correct nucleotides, many polymerases also proofread, checking for errors If errors are found, exonuclease activities remove the incorrect nucleotide 4 Prokaryotic DNA replication DNA synthesis by DNA polimerases Craig et al., Molecular Biology ‐ Principles of Genome Function, 2e, Oxford University Press, (2014) Direction of the synthesis of the new strand is 5’ to 3’ DNA polimerases are: template dependent primer dependent 5 Prokaryotic DNA replication Initiation Replication starts at special sites called origins (ori) of replication In bacteria and some eukaryotes, specific sequences define ori ori are required to direct replication Bacteria have one ori per chromosome, eukaryotes have many Ori are regions where the dsDNA is unwound and separated ready for replication proteins to attach In bacteria, sequences near the ori determine distribution of replicated chromosomes to the daughter cells. ter specifies replication termination Craig et al., Molecular Biology ‐ Principles of Genome Function, 2e, Oxford University Press, (2014) Replication starts at special sites called origins (ori) of replication In bacteria and some eukaryotes, specific sequences define ori ori are required to direct replication Bacteria have one ori per chromosome, eukaryotes have many Ori are regions where the dsDNA is unwound and separated ready for replication proteins to attach In bacteria, sequences near the ori determine distribution of replicated chromosomes to the daughter cells. ter specifies replication termination 6 Prokaryotic DNA replication Initiation Replication starts at special sites called origins (ori) of replication In bacteria and some eukaryotes, specific sequences define ori ori are required to direct replication Bacteria have one ori per chromosome, eukaryotes have many Ori are regions where the dsDNA is unwound and separated ready for replication proteins to attach In bacteria, sequences near the ori determine distribution of replicated chromosomes to the daughter cells. ter specifies replication termination Craig et al., Molecular Biology ‐ Principles of Genome Function, 2e, Oxford University Press, (2014) 7 Prokaryotic DNA replication Initiation of replication ‐ topoisomerases As DNA is unwound, torsional stress is introduced into the DNA – strand separation at the fork results in overwinding ahead of the fork Torsional stresses lead to changes in DNA supercoiling ‐ overwinding makes the DNA ahead of the fork more difficult to unwind Topoisomerases resolve the overwinding by transiently breaking DNA and allowing supercoils to relax. Type IA and IB topoisomerases break one of the two DNA strands and do not require ATP. Type II toposiomerases use ATP and break both strands. Craig et al., Molecular Biology ‐ Principles of Genome Function, 2e, Oxford University Press, (2014) 8 Prokaryotic DNA replication Initiation of replication – single‐stranded binding proteins Single stranded DNA can form secondary structures that would make copying by polymerase difficult Single‐stranded binding proteins (SSB) bind to the displaced single stranded DNA to keep the DNA open and interact with the DNA synthesis machinery. Craig et al., Molecular Biology ‐ Principles of Genome Function, 2e, Oxford University Press, (2014) Single stranded DNA can form secondary structures that would make copying by polymerase difficult Single‐stranded binding proteins (SSB) bind to the displaced single stranded DNA to keep the DNA open and interact with the DNA synthesis machinery. 9 Prokaryotic DNA replication Initiation of replication Craig et al., Molecular Biology ‐ Principles of Genome Function, 2e, Oxford University Press, (2014) DNA synthesis has three phases: initiation, elongation and termination Bi‐directonal DNA synthesis forms a replication bubble DNA synthesis on both strands occurs in a 5′ to 3′ direction by the multi‐enzyme complex termed replisome The origin of replication is recognized by an initiator protein that recruits helicases that opens up the double helix DNA helicases unwind the helix to expose single‐stranded DNA, which is coated with ssDNA binding proteins in bacteria or replication protein A in eukaryotes Initiation is controlled to occur only once per cell cycle DNA synthesis needs a primer – it can only add nucleotides to an existing 3′ end The primer is a short RNA strand synthesized by primase Topoisomerases unwind DNA DNA replication can start once the origin has been opened and helicases loaded Primers, short piece of RNA or RNA+DNA are must be synthesized by primases Primase binds to ssDNA coated with ssDNA binding proteins 10 Primase does not require an existing 3′ OH for synthesis, unlike DNA polymerase Bacterial primase synthesizes an RNA of 10‐30 bases 10 Prokaryotic DNA replication Elongation of replication Craig et al., Molecular Biology ‐ Principles of Genome Function, 2e, Oxford University Press, (2014) After the RNA primer is synthesized, the sliding clamp is recruited. DNA polymerase is associated with DNA via the clamp loader and the sliding clamp. The replication machinery moves along the DNA, copying the strands. Each base in the parental DNA is read by DNA polymerase, which adds complementary bases to the growing strand in a 5′ to 3′ direction. 11 Prokaryotic DNA replication Elongation of replication – the re‐replication block DnaA binds and multimerizes at oriC when bound to ATP After binding, DnaA unwinds DNA for replication initiation After initiation, another protein, Hda, stimulates hydrolysis of the DnaA‐ATP to ADP DnaA‐ADP dissociates and cannot reinitiate origin firing, preventing over‐stimulation of replication initiation This Regulatory Inactivation of DnaA (RIDA) blocks replication initiation RIDA is the major regulatory step preventing initiation Craig et al., Molecular Biology ‐ Principles of Genome Function, 2e, Oxford University Press, (2014) DnaA binds and multimerizes at oriC when bound to ATP After binding, DnaA unwinds DNA for replication initiation After initiation, another protein, Hda, stimulates hydrolysis of the DnaA‐ATP to ADP DnaA‐ADP dissociates and cannot reinitiate origin firing, preventing over‐ stimulation of replication initiation This Regulatory Inactivation of DnaA (RIDA) blocks replication initiation RIDA is the major regulatory step preventing initiation 12 Prokaryotic DNA replication Elongation of replication – the re‐replication block Craig et al., Molecular Biology ‐ Principles of Genome Function, 2e, Oxford University Press, (2014) Initiation at oriC can also be prevented by methylation DNA adenine methylase (Dam methylase) methylates the A residue in the sequence GATC 11 GATC sites in oriC overlap the DnaA binding sites Just after replication, only one strand of DNA is methylated (hemi‐methylation) SeqA binds to these hemi‐methylated GATC sites and blocks binding of methylase. This inhibits methylation and blocks access by DnaA This is only a temporary block – the origin is usually fully methylated ten minutes after initiation Another mechanism discourages re‐firing of origins DnaA can bind to the datA DNA region, which lies closes to oriC This regions sequesters about 25% of free DnaA in the cell Just after replication, there are two of these regions, so much more DnaA is sequestered, making it unavailable to rebind to oriC After segregation of chromosomes, there is again just one copy of datA so there is again enough DnaA available to bind to oriC and promote initiation DnaA‐ATP must be provided for the next round of initiation DnaA‐ATP is newly synthesized, and DnaA‐ADP is recycled to DnaA‐ATP DnaA binds to DARS regions of DNA (DnaA reactivating sequences) DARS serves as cofactors to stimulate exchange of ATP for ADP 13 Prokaryotic DNA replication Elongation of replication – lagging strand Leading and lagging strand synthesis is coupled. DNA on the lagging strand is looped. The DNA loop of the lagging strand needs to be released from polymerase frequently (every couple of seconds). In bacteria, the replication proteins are associated in the replication complex, or replisome The polymerases are linked by two subunits of tau (τ), which associates with the clamp loader and helicase Craig et al., Molecular Biology ‐ Principles of Genome Function, 2e, Oxford University Press, (2014) Leading and lagging strand synthesis is coupled. DNA on the lagging strand is looped. The DNA loop of the lagging strand needs to be released from polymerase frequently (every couple of seconds). In bacteria, the replication proteins are associated in the replication complex, or replisome The bacterial replisome has two copies of DNA polymerase III, sliding clamps and clamp loader The polymerases are linked by two subunits of tau (τ), which associates with the clamp loader and helicase 14 Prokaryotic DNA replication Elongation of replication – Okazaki fragments DNA is synthesized on both strands Because DNA polymerase synthesizes DNA in a 5′ to 3′ direction, DNA is made in short discontinuous fragments on the lagging strand – Okazaki fragments Okazaki fragments are joined after synthesis – Okazaki fragment maturation In E. coli, DNA polymerase III disassociates from the DNA when it reaches the next RNA primer. The sliding clamp remains attached DNA polymerase I removes the RNA primer and then fills in the gap with DNA, but cannot attach the end of the synthesized DNA to the next DNA fragment, leaving a “nick” in the DNA DNA ligase seals the DNA “nick” Craig et al., Molecular Biology ‐ Principles of Genome Function, 2e, Oxford University Press, (2014) DNA is synthesized on both strands Because DNA polymerase synthesizes DNA in a 5′ to 3′ direction, DNA is made in short discontinuous fragments on the lagging strand – Okazaki fragments Okazaki fragments are joined after synthesis – Okazaki fragment maturation In E. coli, DNA polymerase III disassociates from the DNA when it reaches the next RNA primer. The sliding clamp remains attached DNA polymerase I removes the RNA primer and then fills in the gap with DNA, but cannot attach the end of the synthesized DNA to the next DNA fragment, leaving a “nick” in the DNA DNA ligase seals the DNA “nick” 15 Prokaryotic DNA replication Elongation of replication – proofreading Replicative polymerases only make one uncorrected error per ~100,000 nucleotides Nucleotide identity is monitored during and after addition to the growing strand During addition, the polymerase recognizes the correct nucleotide due to the precise fit in the active site when base‐paired with the template strand Proofreading identifies incorrectly incorporated nucleaotides. The 3’‐5’ exonuclease of DNA polymerase III activity removes incorrectly incorporated nucleotides. Polymerase and endonuclease activities are spatially isolated. Removal of mismatched nucleotides is ATP‐dependent. Craig et al., Molecular Biology ‐ Principles of Genome Function, 2e, Oxford University Press, (2014) Replicative polymerases only make one uncorrected error per ~100,000 nucleotides Nucleotide identity is monitored during and after addition to the growing strand During addition, the polymerase recognizes the correct nucleotide due to the precise fit in the active site when base‐paired with the template strand Proofreading identifies incorrectly incorporated nucleaotides. The 3’‐5’ exonuclease of DNA polymerase III activity removes incorrectly incorporated nucleotides. Polymerase and endonuclease activities are spatially isolated. Removal of mismatched nucleotides is ATP‐dependent. 16 Prokaryotic DNA replication Elongation of replication – proofreading 17 Prokaryotic DNA replication Prokaryotic DNA‐dependent DNA polymerases DNA Pol III DNA Pol I 5'‐3' Polymerase Yes Yes (DNA Synthesis) 3'‐5' Exonuclease Yes Yes (Proofreading) 5'‐3' Exonuclease No Yes (Primer Removal) Polymerization Rate High Low Processivity High Low Craig et al., Molecular Biology ‐ Principles of Genome Function, 2e, Oxford University Press, (2014) 18 Prokaryotic DNA replication Termination of DNA synthesis Craig et al., Molecular Biology ‐ Principles of Genome Function, 2e, Oxford University Press, (2014) Termination occurs when two different forks meet or when polymerase meets the previously replicated strand. Replication complexes are disassembled RNA primers are removed and replaced with DNA DNA ligase connects adjacent strands 19 Prokaryotic DNA replication Termination of DNA synthesis Craig et al., Molecular Biology ‐ Principles of Genome Function, 2e, Oxford University Press, (2014) Topoisomerase II untangles interconnected daughter DNA 20 Prokaryotic DNA replication DNA Gyrase Type II topoisomerase Neutralizes the positive supercoils introduced by Helicase strand‐unwinding during DNA replication relaxes DNA by negative supercoiling ATP‐dependent Acts like a swivel out ahead of active replication fork 21 Prokaryotic DNA replication Type II topoisomerase inhibition Quinolones & Fluoroquinolones Quinolones inhibit the ligase activity of gyrase and topoisomerase IV The majority of quinolones in clinical use are fluoroquinolones, which have a fluorine atom attached to the central ring system, typically at the 6‐position or C‐7 position. Most of them are named with the ‐oxacin suffix. e.g. Ciprofloxacin Topoisomerases are essential for cell survival. Eukaryotic topoisomerase inhibitors are slightly different in structure, so have different names. 22 DNA replication Lesson 1 – Eukaryotic DNA replication Zsolt Fábián M.D., Ph.D., Dr. Habil. 23 Eukaryotic DNA replication Eukaryotic DNA polymerases Craig et al., Molecular Biology ‐ Principles of Genome Function, 2e, Oxford University Press, (2014) The main polymerases involved in eukaryotic DNA replication is DNA polymerases δ and ε As well as the main replicative polymerases, other polymerases exist that have special functions Polymerases can be grouped according to the evolutionary lineage of the rest of the protein Polymerases are in groups A, B, C, D X and Y Reverse transcriptases are DNA polymerases that copy RNA into DNA Reverse transcriptases use single‐stranded RNA as a template, but are structurally and catalytically similar to DNA polymerases Reverse transcriptases are encoded by viruses and by DNA elements in eukaryotes called retrotransposons Telomerase, which is needed to synthesize chromosome ends, is a specialized reverse transcriptase 24 Eukaryotic DNA replication Initiation of replication – single‐stranded binding proteins Eukaryotic single‐stranded binding proteins are termed replication protein A (RPA). Craig et al., Molecular Biology ‐ Principles of Genome Function, 2e, Oxford University Press, (2014) Single‐stranded binding proteins are termed replication protein A (RPA). 25 Eukaryotic DNA replication Initiation of replication – the sliding clamp The eukaryotic sliding clamp is termed PCNA. Craig et al., Molecular Biology ‐ Principles of Genome Function, 2e, Oxford University Press, (2014) The high processivity of replicative DNA polymerase is due to the sliding clamp that keeps it tethered to DNA. Both the clamp and clamp loader are highly conserved in bacteria, archaea and eukaryotes. The sliding clamp is a ring shape with a 35 Å hole that encloses the DNA – it is highly stable and stays associated with DNA once loaded. The eukaryotic sliding clamp is termed PCNA. PCNA has three proteins of two similar domains come together. The clamp loader is a 5 subunit ring structure termed Replication Factor C; RFC in eukaryotes. 26 Eukaryotic DNA replication Initiation Craig et al., Molecular Biology ‐ Principles of Genome Function, 2e, Oxford University Press, (2014) Eukaryotes have many (more DNA to replicate) roughly 100,000 origins per nuclear genome In some eukaryotes, specific sequences define ori In eukaryotes, the Origin Recognition Complex (ORC) is the initiator – in S. cerevisiae, ORC has 6 subunits, Orc1‐6 ATP regulates initiator binding – ATP binding by Orc1 is required for ORC to bind DNA 27 Eukaryotic DNA replication Initiation Craig et al., Molecular Biology ‐ Principles of Genome Function, 2e, Oxford University Press, (2014) S. cerevisiae origins have a similar structure to those in E. coli The yeast origin is ~100bp long, and has A1 and B1 sites that the ORC binds to AT‐rich sites, B2 and B3, are adjacent to the A1 and B1 sites As in E. coli, the origin DNA wraps round ORC ORC recruits Cdc6 and Cdt1, which are helicase loading proteins. These recruit the MCM helicase proteins These proteins together are called the pre‐replicative complex Chromatin structure is important for origins in multicellular organisms Replication is initiated in specific chromosomal regions, but the DNA sequences are not conserved In Drosophila, ORC is recruited to regions where histone tails are hyperacetylated – thus, histone acetyltransferases may be involved in regulating replication origins 28 Eukaryotic DNA replication Initiation Craig et al., Molecular Biology ‐ Principles of Genome Function, 2e, Oxford University Press, (2014) Eukaryotes have multiple replication origins Each must fire only once per cell cycle Origins are selected in G1. Origins are activated in S, and cannot be reused until re‐ prepared in another G1 phase Specific initiator proteins bind to an origin to form a complex – the origin recognition complex (ORC) ORC is made up of six subunits, Orc1‐6 ORC is at the center of a larger complex – the pre‐Replicative Complex (pre‐RC) that forms at the origin in G1 pre‐RC can only be assembled in G1, and includes Cdt1, Cdc6 and MCM helicase 29 Eukaryotic DNA replication Initiation Craig et al., Molecular Biology ‐ Principles of Genome Function, 2e, Oxford University Press, (2014) After origin firing, many members of the complex are inactivated so they cannot re‐ trigger initiation until the next cycle In S phase, origins are activated The pre‐RC is phosphorylated by S phase Cdks, making it active This allows the origins to unwind, and activates the Mcm helicase complex Replication must be completed before chromosome segregation occurs If replication forks stall, the DNA damage response is triggered, and mitosis is arrested until errors are fixed 30 Eukaryotic DNA replication Initiation ‐ primase Craig et al., Molecular Biology ‐ Principles of Genome Function, 2e, Oxford University Press, (2014) Primers must be synthesized on the leading strand (infrequently) and lagging strand (frequently) Primase does not require an existing 3′ OH for synthesis, unlike DNA polymerase Bacterial primase synthesizes an RNA of 10‐30 bases Eukaryotic primase has 4 subunits, 2 of which function as primase and make an RNA of 10‐30 bases. The polymerase α subunit then adds a short piece of DNA After the polymerase α subunit of the primase complex makes a short stretch of DNA, replicative polymerases take over – this is polymerase switching Polymerase switching occurs every time a new strand is started Replicative polymerase is recruited by the sliding clamp 31 Eukaryotic DNA replication Initiation ‐ primase Craig et al., Molecular Biology ‐ Principles of Genome Function, 2e, Oxford University Press, (2014) Eukaryotic replication proteins are not physically associated in a replisome, but clamp loader, sliding clamp and primase are all part of the replication machinery at the fork DNA polymerase δ likely replicates the leading strand and DNA polymerase ε the lagging strand DNA Polymerase α is a multisubunit enzyme complex with Primase and DNA Polymerase activities It synthesizes a short RNA primer (~10nt) then switches to DNA synthesis (20‐ 30nt) No proofreading! DNA Polymerase δ is the primary, highly‐processive eukaryotic polymerase Synthesizes both the leading and lagging strand Uses a “sliding clamp” know as PCNA (Proliferating cell nuclear antigen) DNA Polymerase ε is an alternative processive polymerase May be involved leading & lagging strand synthesis Primarily is a DNA repair enzyme 32 Eukaryotic DNA replication Elongation ‐ Okazaki fragment maturation in eukaryotes Craig et al., Molecular Biology ‐ Principles of Genome Function, 2e, Oxford University Press, (2014) Removal of the RNA primer and filling in with new DNA is different in eukaryotes Primers are NOT removed by the 5'‐3' exonuclease activity of a DNA polymerase, no eukaryotic equivalent of DNA polymerase I DNA polymerase continues to make DNA when it meets the RNA primer This displaces the existing RNA primer and DNA from the template strand, making a flap Flap endonuclease (Fen1 or RNase H) cleaves the flap DNA If Fen1 does not cleave the DNA, a long flap can form – in this case Dna2 cleaves the long flap, which is then processed by Fen1 A homolog of Fen1 is required in archaea, suggesting this mechanism is conserved in archaea and eukaryotes, but not bacteria DNA ligase seals the DNA “nick” Okazaki fragments are smaller (100‐200bp vs. 1000‐2000bp) 33 Eukaryotic DNA replication Termination – the end‐replication problem Craig et al., Molecular Biology ‐ Principles of Genome Function, 2e, Oxford University Press, (2014) The mechanism for lagging strand synthesis cannot replicate the very end of a linear chromosome – called the end‐replication problem As a consequence, substantial portions of chromosome ends could be lost over several rounds of replication One cause of this is RNA primer removal, which can leave a gap that cannot be filled in Another cause of failure to replicate the chromosome end is dissolution of the replication fork when the leading strand is finished, before lagging strand synthesis is complete This can lead to the loss of the terminal Okazaki fragment 34 Eukaryotic DNA replication Termination – the end‐replication problem Craig et al., Molecular Biology ‐ Principles of Genome Function, 2e, Oxford University Press, (2014) The end‐replication problem is completely avoided in organisms that have circular chromosomes Some viruses have interesting ways to avoid the problem: ‐In T4 viruses, several copies of the linear chromosome are stuck together before replication, reducing the number of ends ‐In vaccinia viruses, the two DNA strands are joined at the end, making a hairpin, so replication can just continue round the loop ‐In bacteriophage ϕ29, replication is primed from a terminal protein Eukaryotes use telomeres to circumvent the end‐ replication issue 35 Organization of the eukaryotic genome Repetitive sequences Doggett, Overview of Human Repetitive DNA Sequences. Current Protocols in Human Genetics (2001) A.1B.1‐A.1B.5 Telomere repeat tandemly repeating unit TTAGGG extending for 5000 to 12,000bp guanine‐guanine base pairs 36 Eukaryotic DNA replication Termination – the end‐replication problem Craig et al., Molecular Biology ‐ Principles of Genome Function, 2e, Oxford University Press, (2014) Eukaryotic chromosome ends have simple sequence repeats – telomeres Telomeres range in length from ~100bp to > 20,000bp, depending on species The ends of the telomeres get shorter after each round of replication Telomeres can be elongated by adding new specific telomere sequences at the ends In Drosophila, transposable elements transpose onto chromosome ends to maintain length 37 Eukaryotic DNA replication Termination – telomere replication Telomerase is a special DNA polymerase made of a protein and an RNA molecule The RNA provides the template for synthesis of telomere repeats, and has a role in enzyme activity The protein is an enzyme called telomerase reverse transcriptase (TERT), and is well conserved in eukaryotes Telomerase binds to single‐stranded telomere DNA The 3′ end of the DNA base‐pairs with the telomerase RNA The enzyme uses the RNA as a template to synthesize DNA Synthesis is repeated many times from a single RNA template Craig et al., Molecular Biology ‐ Principles of Genome Function, 2e, Oxford University Press, (2014) Telomerase is a special DNA polymerase made of a protein and an RNA molecule The RNA provides the template for synthesis of telomere repeats, and has a role in enzyme activity The protein is an enzyme called telomerase reverse transcriptase (TERT), and is well conserved in eukaryotes Telomerase binds to single‐stranded telomere DNA The 3′ end of the DNA base‐pairs with the telomerase RNA The enzyme uses the RNA as a template to synthesize DNA Synthesis is repeated many times from a single RNA template 38 Eukaryotic DNA replication Telomere replication ‐ Dyskeratosis congenita Craig et al., Molecular Biology ‐ Principles of Genome Function, 2e, Oxford University Press, (2014) Dyskeratosis congenita (DC) nail dystrophy, skin hyperpigmentation, and mucosal leukoplakia, bone marrow failure, premature aging, ataxia, hypoplasia of the cerebellum, and learning disabilities. Cytogenetic show shortening of the teleomeres. One form of DC is X‐linked and is associated with a gene, dyskeratosis congenita 1 (DKC1). The protein product is dyskerin. This is a component of the small nucleolar ribonucleoprotein (snoRNA) particles. Dyskerin plays an important role in the processing of telomere complexes. The autosomal dominant form of DC is associated with mutations in the gene telomerase RNA component (TERC). The product of TERC is an RNA that is a component of the telomere that actually functions as a 39 template. Thus the principal pathology of DC appears to be related to abnormalities of the processing of telomeres. 39 Eukaryotic DNA replication Role of chromatin in replacation Craig et al., Molecular Biology ‐ Principles of Genome Function, 2e, Oxford University Press, (2014) Chromatin consists of DNA plus specific proteins called histone proteins, which are bound to the DNA These proteins must be recruited to the correct places in the daughter DNA molecules after replication DNA modifications like methylation must also be maintained The correct recruitment of histones, and maintenance of chemical modifications such as methylation underpin epigenetic inheritance Eukaryotic DNA is packaged in nucleosomes – these are disrupted by the replication fork, then re‐bind after the fork has passed Half of the parental H3 and H4 go to one daughter, half to the other – parental histone segregation 40 Eukaryotic DNA replication Role of chromatin in replacation Old H3 and H4 are transferred to daughter DNAs by a complex containing Asf1 Asf1 binds a half nucleosome with a tetramer of 2 H3 and 2 H4 molecules and guides them to their new position New nucleosomes are added by Caf1 The newly synthesized H3 and H4 copy the modification states of their neighbors H2A and H2B are then added to the nucleosome Synthesis of these main histone subunits is tightly coupled to DNA synthesis – they are replication‐dependent histones or S phase histones Craig et al., Molecular Biology ‐ Principles of Genome Function, 2e, Oxford University Press, (2014) Old H3 and H4 are transferred to daughter DNAs by a complex containing Asf1 Asf1 binds a half nucleosome with a tetramer of 2 H3 and 2 H4 molecules and guides them to their new position New nucleosomes are added by Caf1 The newly synthesized H3 and H4 copy the modification states of their neighbors H2A and H2B are then added to the nucleosome Synthesis of these main histone subunits is tightly coupled to DNA synthesis – they are replication‐dependent histones or S phase histones 41 Eukaryotic DNA replication Role of chromatin in replacation Newly synthesized H3 and H4 are acetylated at specific lysines These are bound by Asf1 and Caf1 Caf1 interacts with the sliding clamp and deposits the new H3 and H4 onto DNA behind the replication fork Newly synthesized H2A and H2B are added to complete the nucleosome H3 and H4 are then deacetylated and can take on the modification state of the surrounding old nucleosomes Craig et al., Molecular Biology ‐ Principles of Genome Function, 2e, Oxford University Press, (2014) Newly synthesized H3 and H4 are acetylated at specific lysines These are bound by Asf1 and Caf1 Caf1 interacts with the sliding clamp and deposits the new H3 and H4 onto DNA behind the replication fork Newly synthesized H2A and H2B are added to complete the nucleosome H3 and H4 are then deacetylated and can take on the modification state of the surrounding old nucleosomes 42 Eukaryotic DNA replication Decatenation Craig et al., Molecular Biology ‐ Principles of Genome Function, 2e, Oxford University Press, (2014) In eukaryotes there are multiple origins of replication Termination can occur anywhere on the chromosome where two replication forks meet Forks and replication machinery disassemble and the remaining DNA filled in, though it is not yet known precisely how DNA molecules are entwined, as in bacteria, and are resolved by topoisomerases In some regions of eukaryotic chromosomes, replication is blocked in one direction – in yeast a termination site called the replication fork barrier is found in the rDNA The protein FOB1 binds to this site and blocks replication fork progression 43 Eukaryotic DNA replication Pro‐ vs. Eukaryotic replicative enzymes Function Prokaryotic Eukaryotic Initiation Initiator proteins ORC Unwinding Helicase MCM Helicase Remove supercoils Topoisomerase & Gyrase Topoisomerases Priming Primase DNA polymerase α Primary Synth. DNA polymerase III DNA polymerase δ (or ε) Removing Primers DNA polymerase I RNase H or FEN‐1 Gap Filling DNA polymerase I DNA polymerase δ (or ε) Sealing The Nick DNA Ligase DNA Ligase 44 Eukaryotic DNA replication Review figure 1. From the given structure, identify: ‐5' A. Direction of fork movement B. Leading strand template C. Lagging strand template 3'‐ 5'‐ lllllllllllllllllllllllllllllllll 2. Draw the leading and lagging strands, indicating direction of synthesis ‐3' 3. Point out where each of the proteins on the previous slide would act at the fork 45