Nyki's Ch. 09 PPT Fall 2021 (2).ppt
Document Details
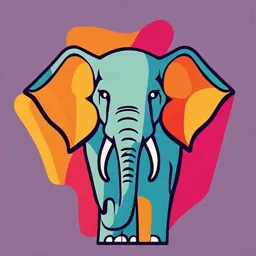
Uploaded by FineLookingPeach996
Full Transcript
Chapter 09 Lecture Outline See separate PowerPoint slides for all figures and tables pre-inserted into PowerPoint without notes and animations. 9-1 Copyright © The McGraw-Hill Companies, Inc. Permission required for reproduction or display. 9.1 Functions of the Muscular System • • • • • • • Move...
Chapter 09 Lecture Outline See separate PowerPoint slides for all figures and tables pre-inserted into PowerPoint without notes and animations. 9-1 Copyright © The McGraw-Hill Companies, Inc. Permission required for reproduction or display. 9.1 Functions of the Muscular System • • • • • • • Movement of the body Maintenance of posture Respiration Production of body heat Communication Constriction of organs and vessels Contraction of the heart 9-2 9.2 General Properties of Muscle • Contractility: ability of a muscle to shorten with force • Excitability: capacity of muscle to respond to a stimulus (from our nerves) • Extensibility: muscle can be stretched to its normal resting length and beyond to a limited degree • Elasticity: ability of muscle to recoil to original resting length after stretched 9-3 Types of Muscle Tissue • Skeletal – Responsible for locomotion, facial expressions, posture, respiratory movements, other types of body movement – Voluntary • Smooth – Walls of hollow organs, blood vessels, eye, glands, skin – Some functions: propel urine, mix food in digestive tract, dilating/constricting pupils, regulating blood flow – In some locations, autorhythmic – Controlled involuntarily by endocrine and autonomic nervous systems • Cardiac – Heart: major source of movement of blood – Autorhythmic – Controlled involuntarily by endocrine and autonomic nervous systems 9-4 Organization of the Nervous System Copyright 2009 John Wiley & Sons, Inc. Diff. Text 9.0; Slide #9-5 9.3 Skeletal Muscle Structure Copyright © The McGraw-Hill Companies, Inc. Permission required for reproduction or display. • Composed of muscle cells (fibers), connective tissue, blood vessels, nerves • Fibers are long, cylindrical, multinucleated • Tend to be smaller diameter in small muscles and larger in large muscles. 1 mm- 4 cm in length • Develop from myoblasts; numbers remain constant • Striated appearance due to light and dark banding LM 800x Skeletal muscle fiber Nucleus Striations © Ed Reschke Fig.9.2; Slide # 9-6 Connective Tissue Coverings of Muscle • Layers – Epimysium. C.T. that surrounds a whole muscle (many fascicles) – Perimysium. Denser C.T. surrounding a group of muscle fibers. Each group called a fasciculus – Endomysium. Loose C.T. with reticular fibers. • Muscular fascia: connective tissue sheet – External to epimysium – Holds muscles together and separates them into functional groups. 9-7 Copyright © The McGraw-Hill Companies, Inc. Permission required for reproduction or display. Muscular fascia (surrounds individual muscles and groups of muscles) Artery Vein Epimysium (surrounds muscles) Perimysium (surrounds fasciculi) Nerve Endomysium (surrounds muscle fibers) Muscle fiber Artery Nerve Vein Fasciculus Capillary Axon of motor neuron Synapse or neuromuscular junction Fig. 9.1; Slide # 9-8 Nerves and Blood Vessel Supply Copyright © The McGraw-Hill Companies, Inc. Permission required for reproduction or display. Muscular fascia (surrounds individual muscles and groups of muscles) Artery Vein Nerve Epimysium (surrounds muscles) Perimysium (surrounds fasciculi) Endomysium (surrounds muscle fibers) Muscle fiber Artery Nerve Vein Fasciculus • Motor neurons: – stimulate muscle fibers to contract. Nerve cells with cell bodies in brain or spinal cord; axons extend to skeletal muscle fibers through nerves – Axons branch so that each muscle fiber is innervated – Contact is neuromuscular junction Capillary • Capillary beds surround muscle fibers Axon of motor neuron Synapse or neuromuscular junction Fig. 9.1; Slide #9-9 Skeletal Muscle Fibers • Several nuclei just inside sarcolemma • Cell packed with myofibrils within cytoplasm (sarcoplasm) – Threadlike – Composed of protein threads called myofilaments: thin (actin) and thick (myosin) – Sarcomeres: highly ordered repeating units of myofilaments (actin & myosin) 9-10 Skeletal Muscle Tissue • Sarcolemma – The plasma membrane of a muscle cell • Transverse (T) tubules – Connect the plasma membrane to the interior of the cell – Muscle action potentials travel through the T tubules – Ensure that action potential excites all parts of the muscle fiber at the same time • Sarcoplasm, the cytoplasm of a muscle fiber – Sarcoplasm includes glycogen used for synthesis of ATP and a redcolored protein called myoglobin which binds oxygen molecules – Myoglobin releases oxygen when it is needed for ATP production Copyright 2009, John Wiley & Sons, Inc. Skeletal Muscle Tissue • Myofibrils – Thread-like structures which have a contractile function • Sarcoplasmic reticulum (SR) – Membranous sacs which encircles each myofibril – Stores calcium ions (Ca2+) – Release of Ca2+ triggers muscle contraction • Filaments (AKA Myofilaments) – Function in the contractile process – Two types of filaments (Thick and Thin) – There are two thin filaments for every thick filament • Sarcomeres – Compartments of arranged filaments – Basic functional unit of a myofibril Copyright 2009, John Wiley & Sons, Inc. Skeletal Muscle Tissue Diff Text 9.3 (a); Slide # 9-13 Skeletal Muscle Tissue Diff Text 9.3 (b); Slide # 9-14 • Z discs Skeletal Muscle Tissue – Separate one sarcomere from the next • A band – Darker middle part of the sarcomere – Thick and thin filaments overlap • I band – Lighter, contains thin filaments but no thick filaments – Z discs pass through the center of each I band • H zone – Center of each A band which contains thick but no thin filaments • M line – Supporting proteins that hold the thick filaments together in the H zone Copyright 2009, John Wiley & Sons, Inc. Sarcomeres: Z Disk to Z Disk • Sarcomere: basic functional unit of muscle fiber • Z disk: filamentous network of protein. Serves as attachment for actin myofilaments • Striated appearance – I bands: from Z disks to ends of thick filaments; Lighter, contains thin filaments but no thick filaments; Z discs pass through the center of each I band – A bands: length of thick filaments; Darker middle part of the sarcomere; Thick and thin filaments overlap – H zone: region in A band where actin and myosin do not overlap; contains thick but no thin filaments – M line: middle of H zone; delicate filaments holding myosin in place 9-16 Contraction and Relaxation of Skeletal Muscle Diff. Text. 9.3 (c); Slide # 9-17 Skeletal Muscle Tissue • Muscle Proteins – Myofibrils are built from three kinds of proteins • Contractile proteins – Generate force during contraction • Regulatory proteins – Switch the contraction process on and off • Structural proteins – Align the thick and thin filaments properly – Provide elasticity and extensibility – Link the myofibrils to the sarcolemma Copyright 2009, John Wiley & Sons, Inc. 9-18 Skeletal Muscle Tissue • Contractile Proteins – Myosin • Thick filaments • Functions as a motor protein which can achieve motion • Converts ATP to energy of motion (ATPase enzymes, breaks down/hydrolyzes adenosine triphosphate (ATP), releasing energy. Part of the energy is used to bend the hinge region of the myosin molecule during contraction) • Projections of each myosin molecule protrude outward (myosin head) – Actin • • • • Thin filaments Actin molecules provide a site where a myosin head can attach Tropomyosin and troponin are also part of the thin filament In relaxed muscle: – Myosin is blocked from binding to actin – Strands of tropomyosin cover the myosin-binding sites • Calcium ion binding to troponin moves tropomyosin away from myosin-binding sites (prior to contraction) • Allows muscle contraction to begin as myosin binds to actin 9-19 Copyright 2009, John Wiley & Sons, Inc. Actin (Thin) Myofilaments • Two strands of actin form a double helix extending the length of the myofilament; attached at either end at sarcomere. – Each monomer of actin has an active site – Actin site can bind myosin during muscle contraction. • Tropomyosin: an elongated protein winds along the groove of the double helix. • Troponin is composed of three subunits: one that binds to actin, a second that binds to tropomyosin, and a third that binds to calcium ions •The tropomyosin/troponin complex regulates the interaction between active sites on G actin and myosin. 9-20 Contraction and Relaxation of Skeletal Muscle Copyright 2009, John Wiley & Sons, Inc. Diff Text 9.3 (d); Slide # 9-21 Skeletal Muscle Tissue • Structural Proteins – Titin • Stabilizes the position of myosin • Accounts for much of the elasticity and extensibility of myofibrils – Dystrophin • Links thin filaments to the sarcolemma – Nebulin • Anchors Actin to Z-disc & regulates the length of Actin during development – Myomesin • Forms the M-line & binds Titan; connects adjacent myosin filaments together – Alpha-Actinin • Binds Z-disc to Actin & to Titan Copyright 2009, John Wiley & Sons, Inc. 9-22 9.4 Sliding Filament Model • Actin myofilaments sliding over myosin to shorten sarcomeres – Actin and myosin do not change length – Shortening sarcomeres responsible for skeletal muscle contraction • During relaxation, sarcomeres lengthen because of some external force, like contraction of antagonistic muscles – Muscles that produce the opposite effect 9-23 Contraction and Relaxation of Skeletal Muscle Copyright 2009, John Wiley & Sons, Inc. Diff Text Fig. 9.4; Slide # 9-24 Contraction and Relaxation of Skeletal Muscle Copyright 2009, John Wiley & Sons, Inc. Diff. Text 9.5; Slide # 9-25 9.5 Physiology of Skeletal Muscle Fibers Copyright © The McGraw-Hill Companies, Inc. Permission required for reproduction or display. Oscilloscope + + + + + + + + + 0 – – – – – – – – – mV–50 –85 – – – – – – – – – Time + + + + + + + + + Nerve or muscle cell Measuring the resting membrane potential • Nervous system controls muscle contractions through action potentials • Resting membrane potentials – Membrane voltage difference across membranes (polarized) • Inside cell more negative due to accumulation of large protein molecules. More K+ on inside than outside. K+ leaks out but not completely because negative proteins hold some back. • Outside cell more positive and more Na+ on outside than inside. • Na+ /K+ pump maintains this situation. Fig. 9.7; Slide # 9-26 – Must exist for action potential to occur Concentration Gradient Concentration Gradient: is the difference established when a physical barrier prevents particles from diffusing until equilibrium is reached. •The plasma membrane of a neuron orNMJ creates a barrier that prevents ions (specifically Na+ and K+) from freely crossing. The difference in the concentration of a particular ion coupled with the differences in the charge on opposites of the plasma membrane creates both an electrical and a chemical gradient. Membrane potential is the voltage difference/gradient established by the differing concentrations of ions on opposite sides of a cellular membrane. This is written as: –70 mV, meaning the inside of the cell is 70mV more negative compared to the outside of the cell. <-70mV for a neuron; -90mV for skeletal muscle> http://www.sumanasinc.com/webcontent/animations/content/electricalsignaling.html 9-27 Resting Membrane Potential Resting Membrane Potential: the potential difference of the charges across the cell membrane when the cell is not excited. This is established by the varying ion concentration as well as protein concentration on either side of the membrane. AT REST: [K+] is greater INSIDE the cell vs. outside the cell [Na+] is greater outside the cell vs. INSIDE the cell 9-28 Copyright © The McGraw-Hill Companies, Inc. Permission required for reproduction or display. Oscilloscope 0 + + + + + + + + + – – – – – – – – – mV–50 –85 Time – – – – – – – – – + + + + + + + + + Nerve or muscle cell (a) Measuring the resting membrane potential K+ 1 In a resting cell, there is a higher concentration of K+ (purple circles) inside the cell membrane and a higher concentration of Na+ (pink circles) outside the cell membrane. Because the membrane is not permeable to negatively charged proteins (green) they are isolated to inside of the cell membrane. K+ leak channel Pr – Na+ leak channel Pr – Pr – 2 3 There are more K+ leak channels than Na+ leak channels. In the resting cell, only the leak channels are opened; the gated channels (not shown) are closed. Because of the ion concentration differences across the membrane, K+ diffuses out of the cell down its concentration gradient and Na+ diffuses into the cell down its concentration gradient. The tendency for K+ to diffuse out of the cell is opposed by the tendency of the positively charged K+ to be attracted back into the cell by the negatively charged proteins. The sodium-potassium pump helps maintain the differential levels of Na+ and K+ by pumping three Na+ out of the cell in exchange for two K+ into the cell. The pump is driven by ATP hydrolysis. The resting membrane potential is established when the movement of K+ out of the cell is equal to the movement of K+ into the cell. (b) Generation of the resting membrane potential Na+ concentration gradient K+ concentration gradient Na+ Pr – Pr – Sodiumpotassium pump Pr – ATP ADP Fig. 9.7; Slide # 9-29 • Types Ion Channels – Ligand-gated. Ligands are molecules that bind to receptors. Receptor: protein or glycoprotein with a receptor site • Example: neurotransmitters • Gate is closed until neurotransmitter attaches to receptor molecule. When Ach attaches to receptor on muscle cell, Na+ gate opens. Na+ moves into cell due to concentration gradient – Voltage-gated • Open and close in response to small voltage changes across plasma membrane • Each is specific for certain ions 9-30 Action Potentials Copyright © The McGraw-Hill Companies, Inc. Permission required for reproduction or display. (mV) 0- Threshold Polarized –85 - Depolarization Time (ms) (a) Depolarization is a change of the charge difference across the plasma membrane, making the charge inside the cell less negative and the charge outside the plasma membrane less positive. Once threshold is reached, an action potential is produced. +20 0 Depolarization phase Repolarization phase (mV) • Phases – Depolarization: Inside of plasma membrane becomes less negative. If change reaches threshold, depolarization occurs – Repolarization: return of resting membrane potential. Note that during repolarization, the membrane potential drops lower than its original resting potential, then rebounds. This is because Na+ plus K+ together are higher, but then Na/K+ pump restores the resting potential • All-or-none principle: like camera flash system • Propagate: Spread from one location to another. Action potential does not move along the membrane: new action potential at each successive location. • Frequency: number of action potential produced per unit of time Threshold Polarized –85 Depolarization Time (ms) (b) During the depolarization phase of the action potential, the membrane potential changes from approximately –85 mV to approximately +20 mV. During the repolarization phase, the inside of the plasma membrane changes from approximately +20 mV back to –85 mV. Fig. 9.8 (a & b); Slide# 9-31 Action Potentials Copyright 2009 John Wiley & Sons, Inc. Diff Text 9.8 (b); Slide# 9-32 Gated Ion Channels and the Action Potential Copyright © The McGraw-Hill Companies, Inc. Permission required for reproduction or display. Na+ Na+ channel 1 Resting membrane potential. Na+ channels (pink) and some, but not all, K+ channels (purple) are closed. K+ diffuses down its concentration gradient through the open K+ channels, making the inside of the cell membrane negatively charged compared to the outside. K+ concentration gradient Extracellular fluid K+ channel Charge Difference Across the Plasma Membrane + + + + + + + – – – – – – – 1 Cytoplasm K+ Na+ – – – – – – – + + + + + + + 2 Depolarization. Na + channels are open. Na+ diffuses down its concentration gradient through the open Na+ channels, making the inside of the cell membrane positively charged compared to the outside. Na+ concentration gradient Na+ 2 Na+ diffuse into cell Na+ 3 Repolarization. Na + channels are closed, and Na + movement into the cells stops. More K + channels open. K+ movement out of the cell increases, making the inside of the cell membrane negatively charged compared to the outside, once again. K+ concentration gradient K+ diffuse out of cell + + + + + + + – K+ + K channels open K+ Na+ channels close – – – – – – 3 Fig. 9.9; Slide# 9-33 Action Potential Propagation Copyright © The McGraw-Hill Companies, Inc. Permission required for reproduction or display. 1 An action potential in a local area of the plasma membrane is indicated by the orange band. Note the reversal of charge across the membrane. + + – – + + + + + + + + – – + + – – – – – – – – 1 – – + + – – – – – – – – + + – – + + + + + + + + Stimulus 2 The action potential is a stimulus that causes another action potential to be produced in the adjacent plasma membrane. 3 The action potential propagates along the plasma membrane (orange arrow). + + + + – – – – Muscle fiber – – + + + + + + + + – – – – – – 2 – – – – + + + + + + – – – – – – – – + + + + + + + + + + + + – – – – – – – – + + + + + + – – – – – – – – – – + + + + + + + + – – – – – – + + + Fig. + 9.10; Slide# 9-34 3 Contraction and Relaxation of Skeletal Muscle • Excitation–Contraction Coupling – An increase in Ca2+ concentration in the muscle starts contraction – A decrease in Ca2+ stops it – Action potentials causes Ca2+ to be released from the SR into the muscle cell – Ca2+ moves tropomyosin away from the myosin-binding sites on actin allowing cross-bridges to form – The muscle cell membrane contains Ca2+ pumps to return Ca2+ back to the SR quickly • Decreasing calcium ion levels – As the Ca2+ level in the cell drops, myosin-binding sites are covered and the muscle relaxes Copyright 2009, John Wiley & Sons, Inc. Neuromuscular Junction Copyright © The McGraw-Hill Companies, Inc. Permission required for reproduction or display. Presynaptic terminal Axon branch Synaptic vesicles Neuromuscular junction Presynaptic terminal Sarcolemma Capillary Sarcoplasm Muscle fiber Sarcoplasmic reticulum (a) Myofibrils (b) Mitochondrion Axon branches Neuromuscular junctions Skeletal muscle fiber Postsynaptic membrane (sarcolemma) Synaptic cleft • Synapse: axon terminal resting in an invagination of the sarcolemma • Neuromuscular junction (NMJ): – Presynaptic terminal: axon terminal with synaptic vesicles – Synaptic cleft: space – Postsynaptic membrane or motor end-plate • sarcolemma (c) c: © Fred Hossler/Visuals Unlimited Fig. 9.11 (a) , (b), & (c); Slide# 9-36 Contraction and Relaxation of Skeletal Muscle • The Neuromuscular Junction – Motor neurons have a threadlike axon that extends from the brain or spinal cord to a group of muscle fibers – Action potentials arise at the interface of the motor neuron and muscle fiber • Synapse – Where communication occurs between a somatic motor neuron and a muscle fiber • Synaptic cleft – Gap that separates the two cells • Neurotransmitter – Chemical released from a presynaptic membrane that diffuses across the synaptic cleft and stimulates (or inhibits) the production of an action potential in the postsynaptic membrane. (Acetylcholine) • Synaptic vesicles – Sacs suspended within the synaptic end bulb containing molecules of the neurotransmitter acetylcholine (Ach) – Acetylcholinesterase: A degrading enzyme in synaptic cleft. Prevents accumulation of ACh • Motor end plate – The region of the muscle cell membrane opposite the synaptic end bulbs – Contains acetylcholine receptors Copyright 2009, John Wiley & Sons, Inc. Copyright © The McGraw-Hill Companies, Inc. Permission required for reproduction or display. 1 An action potential (orange arrow) arrives at the presynaptic terminal and causes voltage-gated Ca2+ channels in the presynaptic membrane to open. 2 Calcium ions enter the presynaptic terminal and initiate the release of the neurotransmitter acetylcholine (ACh) from synaptic vesicles. 3 ACh is released into the synaptic cleft by exocytosis. 4 ACh diffuses across the synaptic cleft and binds to ligand-gated Na+ channels on the postsynaptic membrane. 5 Ligand-gated Na+ channels open and Na+ enters the postsynaptic cell, causing the postsynaptic membrane to depolarize. If depolarization passes threshold, an action potential is generated along the postsynaptic membrane. Ac tio n po te nti al Voltage-gated Ca2+ channel Synaptic vesicles Presynaptic terminal Postsynaptic membrane 1 ACh Ca2+ Ca2+ Acetic acid 9 Synaptic cleft Acetic acid 6 ACh unbinds from the ligand-gated Na+ Choline 2 channels, which then close. 8 7 The enzyme acetylcholinesterase, which is attached to the postsynaptic membrane, removes acetylcholine from the synaptic cleft by breaking it down into acetic acid and choline. 8 9 3 Na+ Choline is symported with Na+ into the presynaptic terminal, where it can be recycled to make ACh. Acetic acid diffuses away from the synaptic cleft. ACh is reformed within the presynaptic terminal using acetic acid generated from metabolism and from choline recycled from the synaptic cleft. Ach is then taken up by synaptic vesicles. 7 ACh 4 Action potential Ligand-gated Na+ channel (open) Choline ACh receptor site 6 Action potential Acetylcholinesterase 5 Na+ Ligand-gated Na+ channel (closed) Fig. 9.12; Slide# 9-38 Contraction and Relaxation of Skeletal Muscle Copyright 2009, John Wiley & Sons, Inc. Diff Text 9.13 (a); Slide# 9-39 Copyright 2009, John Wiley & Sons, Inc. Diff. Text. Fig. 9.13 (b); Slide# 9-40 Muscle Relaxation • Ca2+ moves back into sarcoplasmic reticulum by active transport. Requires energy • Ca2+ moves away from troponintropomyosin complex • Complex re-establishes its position and blocks binding sites. 9-41 9.6 Physiology of Skeletal Muscle Copyright © The McGraw-Hill Companies, Inc. Permission required for reproduction or display. Tension • Muscle Twitch Lag Contraction Relaxation phase phase phase Stimulus applied Time – Muscle contraction in response to a stimulus that causes action potential in one or more muscle fibers – Phases • Lag or latent • Contraction • Relaxation Fig. 9.17; Slide# 9-42 Motor Units • Motor units: a single motor neuron and all muscle fibers innervated by it • Motor Unit numbers: – Large muscles have motor units with many muscle fibers. – Small muscles that make delicate movements contain motor units with few muscle fibers Copyright © The McGraw-Hill Companies, Inc. Permission required for reproduction or display. Axon of motor Axon branches neuron Myofibrils Axons of motor neurons Neuromuscular junctions Muscle fibers (a) Neuromuscular Capillary junction Muscle fibers (b) b: © Don Fawcett/Photo Researchers, Inc. Fig. 9.18 (a) & (b); Slide#9-43 Stimulus Strength and Motor Unit Response • All-or-none law for muscle fibers – Contraction of equal force in response to each action potential • Sub-threshold stimulus: no action potential; no contraction • Threshold stimulus: action potential; contraction • Stronger than threshold; action potential; contraction equal to that with threshold stimulus Copyright © The McGraw-Hill Companies, Inc. Permission required for reproduction or display. Axon of motor neuron Axon branches Myofibrils Axons of motor neurons Neuromuscular junctions Muscle fibers (a) Neuromuscular junction Capillary Muscle fibers (b) b: © Don Fawcett/Photo Researchers, Inc. Fig. 9.18 (a) & (b); Slide#9-44 Stimulus Strength and Motor Unit Response • Strength of contraction is graded: ranges from weak to strong depending on the SIZE of the motor unit AND the number of units recruited. • Multiple motor unit summation: strength of contraction depends upon recruitment of motor units. A muscle has many motor units – Submaximal stimuli – Maximal stimulus – Supramaximal stimuli Tension Copyright © The McGraw-Hill Companies, Inc. Permission required for reproduction or display. Increasing stimulus strengths Subthreshold stimulus (no motor units respond) Threshold stimulus (one motor unit responds) Maximal stimulus (all motor units respond) Submaximal stimuli (increasing numbers of motor units respond) Time Supramaximal stimuli (all motor units respond) Fig. 9.20; Slide# 9-45 Treppe Tension Tr ep pe Copyright © The McGraw-Hill Companies, Inc. Permission required for reproduction or display. Stimuli of constant strength Time (ms) • Graded response • Occurs in muscle rested for prolonged period • Each subsequent contraction is stronger than previous until all equal after few stimuli • Possible explanation: more and more Ca2+ remains in sarcoplasm and is not all taken up into the sarcoplasmic reticulum Fig. 9.19; Slide# 9-46 Stimulus Frequency and Whole Muscle Contraction • As the frequency of action potentials increase, the frequency of contraction increases – Incomplete tetanus: muscle fibers partially relax between contraction – Complete tetanus: no relaxation between contractions – Multiple-wave summation: muscle tension increases as contraction frequencies increase Copyright © The McGraw-Hill Companies, Inc. Permission required for reproduction or display. Incomplete tetanus Twitch Frequency 2 Frequency 3 Frequency 4 Tension Frequency 1 Complete tetanus Fig. 9.21; Slide# 9-47 Time (ms) Copyright © The McGraw-Hill Companies, Inc. Permission required for reproduction or display. At the normal resting length of a muscle, the sarcomeres are also at an optimal length. The muscle produces maximum tension in response to a maximal stimulus at this length. Tension (% of maximum) Optimal sarcomere length 100 2 1 50 3 0 60 80 1 At muscle/sarcomere length 1, the muscle is not stretched, and the tension produced when the muscle contracts is small because there is too much overlap between actin and myosin myofilaments. The myosin myofilaments run into the Z disks, and the actin myofilaments interfere with each other at the center of the sarcomere, reducing thenumber of Cross-bridges that can form. Muscle length 3 100 50 Time At muscle/sarcomere length 2, the muscle is optimally stretched, and the tension produced when the muscle contracts is maximal because there is optimal overlap of actin and myosin myofilaments, so the number of crossbridges that can form is maximal. Tension (% of maximum) Time 3 Muscle length 2 Tension (% of maximum) Tension (% of maximum) 50 180 2 Muscle length 1 100 100 120 140 160 % of resting sarcomere length 100 50 Time At muscle/sarcomere length 3, the muscle is stretched severely, and the tension produced when the muscle contracts is small because there is little overlap between actin and myosin myofilaments, and few cross-bridges can form. Fig. 9. 22; Slide# 9-48 Muscle Contractions • Isometric: no change in length but tension increases – Postural muscles of body • Isotonic: change in length but tension constant – Concentric: overcomes opposing resistance and muscle shortens – Eccentric: tension maintained but muscle lengthens • Muscle tone: constant tension by muscles for long periods of time 9-49 9.7 Muscle Fatigue • Decreased capacity to work and reduced efficiency of performance • Types – Psychological: depends on emotional state of individual – Muscular: results from ATP depletion – Synaptic: occurs in NMJ due to lack of acetylcholine 9-50 Physiological Contracture and Rigor Mortis • Physiological contracture: state of fatigue where due to lack of ATP neither contraction nor relaxation can occur • Rigor mortis: development of rigid muscles several hours after death. Ca2+ leaks into sarcoplasm and attaches to myosin heads and crossbridges form. Rigor ends as tissues start to deteriorate. 9-51 9.8 Energy Sources • ATP provides immediate energy for muscle contractions. Produced from three sources – Creatine phosphate • During resting conditions stores energy to synthesize ATP – Anaerobic respiration • Occurs in absence of oxygen and results in breakdown of glucose to yield ATP and lactic acid – Aerobic respiration • Requires oxygen and breaks down glucose to produce ATP, carbon dioxide and water • More efficient than anaerobic • Oxygen debt: oxygen taken in by the body, above that required for resting metabolism after exercise. ATP produced from anaerobic sources contributes 9-52 Copyright © The McGraw-Hill Companies, Inc. Permission required for reproduction or display. TABLE 9.3 Sources of ATP in Muscles Creatine Phosphate Anaerobic Respiration Creatine phosphate Glucose Glucose 1 ATP Metabolic Process Aerobic Respiration 2 ATP Glycolysis Creatine 2ATP Glycolysis 2 pyruvic acid 2 pyruvic acid Fatty acids Amino acids 2 lactic acid Electron-transport chain Citric acid cycle 34 ATP Energy Source Creatine phosphate Glucose Glucose, fatty acids, aminoacids Oxygen Required No No Yes ATP Yield 1 per creatine phosphate 2 per glucose molecule Up to 36 per glucose molecule Duration of Energy Supply Up to 10 seconds Upto 3 minutes Hours Type of Work Supported Moderate exercise and extreme exercise Extreme exercise Resting and all exercise 9-53 Muscle Metabolism Copyright 2009, John Wiley & Sons, Inc. Diff Text 9.22 (b); Slide# 9-54 Slow and Fast Fibers • Distribution of fast-twitch and slow-twitch – Most muscles have both but varies for each muscle • Effects of exercise: change in size of muscle fibers – Hypertrophy: increase in muscle size • Increase in myofibrils • Increase in nuclei due to fusion of satellite cells • Increase in strength due to better coordination of muscles, increase in production of metabolic enzymes, better circulation, less restriction by fat – Atrophy: decrease in muscle size • Reverse except in severe situations where cells die 9-55 Types of Skeletal Muscle Fibers • Muscle fibers contract at different speeds, and vary in how quickly they fatigue • Muscle fibers are classified into three main types – Slow oxidative fibers – Fast oxidative-glycolytic fibers – Fast glycolytic fibers Copyright 2009, John Wiley & Sons, Inc. 9.9 Slow and Fast Fibers • Slow-twitch oxidative – Contract more slowly, smaller in diameter, better blood supply, more mitochondria, more fatigue-resistant than fasttwitch, large amount of myoglobin. – Postural muscles, more in lower than upper limbs. Dark meat of chicken. • Fast-twitch – Respond rapidly to nervous stimulation, contain myosin that can break down ATP more rapidly than that in Type I, less blood supply, fewer and smaller mitochondria than slowtwitch – Lower limbs in sprinter, upper limbs of most people. White meat in chicken. – Comes in oxidative and glycolytic forms 9-57 Types of Skeletal Muscle Fibers • Slow Oxidative Fibers (SO fibers) – – – – – Smallest in diameter Least powerful type of muscle fibers Appear dark red (more myoglobin) Generate ATP mainly by aerobic cellular respiration Have a slow speed of contraction • Twitch contractions last from 100 to 200 msec – Very resistant to fatigue – Capable of prolonged, sustained contractions for many hours – Adapted for maintaining posture and for aerobic, endurance-type activities such as running a marathon Copyright 2009, John Wiley & Sons, Inc. Types of Skeletal Muscle Fibers • Fast Oxidative–Glycolytic Fibers (FOG fibers) – Intermediate in diameter between the other two types of fibers – Contain large amounts of myoglobin and many blood capillaries – Have a dark red appearance – Generate considerable ATP by aerobic cellular respiration – Moderately high resistance to fatigue – Generate some ATP by anaerobic glycolysis – Speed of contraction faster • Twitch contractions last less than 100 msec – Contribute to activities such as walking and sprinting Copyright 2009, John Wiley & Sons, Inc. Types of Skeletal Muscle Fibers • Fast Glycolytic Fibers (FG fibers) – – – – – – – – – – Largest in diameter Generate the most powerful contractions Have low myoglobin content Relatively few blood capillaries Few mitochondria Appear white in color Generate ATP mainly by glycolysis Fibers contract strongly and quickly Fatigue quickly Adapted for intense anaerobic movements of short duration • Weight lifting or throwing a ball Copyright 2009, John Wiley & Sons, Inc. Types of Skeletal Muscle Fibers Copyright 2009, John Wiley & Sons, Inc. 9.10 Heat Production • Exercise: metabolic rate and heat production increase. • Post-exercise: metabolic rate stays high due to oxygen debt. • Excess heat lost because of vasodilation and sweating • Shivering: uncoordinated contraction of muscle fibers resulting in shaking and heat production 9-62 9.11 Smooth Muscle • • • • • Not striated, fibers smaller than those in skeletal muscle Spindle-shaped; single, central nucleus More actin than myosin Caveolae: indentations in sarcolemma; may act like T tubules Dense bodies instead of Z disks as in skeletal muscle; have noncontractile intermediate filaments • Ca2+ required to initiate contractions; binds to calmodulin which regulates myosin kinase. Cross-bridging occurs • Relaxation: caused by enzyme myosin phosphatase 9-63 Smooth Muscle Anatomy LM 800x Nuclei of smooth muscle cells © Victor Eroschenko Copyright © The McGraw-Hill Companies, Inc. Permission required for reproduction or display. Fig. 9.23; Slide#9-64 9-64 Smooth Muscle Anatomy Copyright © The McGraw-Hill Companies, Inc. Permission required for reproduction or display. Actin myofilament Myosin myofilament Dense bodies in sarcoplasm LM 800x Contraction Dense area attached to sarcolemma Intermediate filaments Myofilaments Copyright © The McGraw-Hill Companies, Inc. Permission required for reproduction or display. Fig. 9.24 Slide# 9-65 Types of Smooth Muscle • Visceral or unitary: cells in sheets; function as a unit – Numerous gap junctions; waves of contraction – Often autorhythmic • Multiunit: cells or groups of cells act as independent units – Sheets (blood vessels); bundles (arrector pili and iris); single cells (capsule of spleen) 9-66 Electrical Properties of Smooth Muscle • Slow waves of depolarization and repolarization transferred from cell to cell • Depolarization caused by spontaneous diffusion of Na+ and Ca2+ into cell • Does not follow all-or-none law • May have pacemaker cells • Contraction regulated by nervous system and by hormones (mV) (mV) (mV) Copyright © The McGraw-Hill Companies, Inc. Permission required for reproduction or display. – 60 – 60 Time (ms) (a) Slow waves of depolarization – 60 Time (ms) (b) Action potentials in smooth muscle superimposed on a slow wave of depolarization Time (ms) (c) Action potential with prolonged depolarization (plateau) Fig. 9.26; Slide# 9-67 Functional Properties of Smooth Muscle • Some visceral muscle exhibits autorhythmic contractions • Tends to contract in response to sudden stretch but not to slow increase in length • Exhibits relatively constant tension: smooth muscle tone • Amplitude of contraction remains constant although muscle length varies 9-68 Regulation of Smooth Muscle • Innervated by autonomic nervous system • Neurotransmitters are acetylcholine and norepinephrine • Hormones important as epinephrine and oxytocin • Receptors present on plasma membrane; which neurotransmitters or hormones bind determines response 9-69 Copyright © The McGraw-Hill Companies, Inc. Permission required for reproduction or display. Hormone Ca2+ Hormone receptor 1 A hormone combines with a hormone receptor and activates a G protein mechanism, or depolarization of the plasma membrane occurs. GDP GTP G protein complex GTP replaces separates GDP on subunit. from receptor. Calmodulin (inactive) 2 2 An α subunit opens the Ca2+ channel in the plasma membrane, or depolarization opens Ca2+ channels. Calcium ions diffuse through the Ca2+ channels and combine with calmodulin. 3 Calmodulin with a Ca2+ bound to it binds with myosin kinase and activates it. 4 Activated myosin kinase attaches phosphate from ATP to myosin heads to activate the contractile process. 5 A cycle of cross-bridge formation, movement, detachment, and cross-bridge formation occurs. 1 Ca2+ channel (closed) Myosin kinase (inactive) Ca2+ channel (open) α GTP α subunit with GTP binds to Ca2+ channel and causes it to open. Ca2+ bound to calmodulin 3 Myosin kinase (active) Calmodulin (active) ATP 4 ADP Myosin P Actin Myosin P 5 Actin 6 Relaxation occurs when myosin phosphatase removes phosphate from myosin. Myosin phosphatase Myosin P 6 Fig. 9.25; Slide# 9-70 9.12 Cardiac Muscle • • • • • • Found only in heart Striated Each cell usually has one nucleus Has intercalated disks and gap junctions Autorhythmic cells Action potentials of longer duration and longer refractory period • Ca2+ regulates contraction 9-71 9.13 Effects of Aging on Skeletal Muscle • Reduced muscle mass • Increased time for muscle to contract in response to nervous stimuli • Reduced stamina • Increased recovery time • Loss of muscle fibers • Decreased density of capillaries in muscle 9-72 Ch. 9 Learning Objectives After reading this chapter, students should be able to: • Explain the four functional properties of muscle tissue. • Summarize the major characteristics of skeletal, smooth, and cardiac muscle. • Describe the connective tissue components of skeletal muscle. • Explain the blood supply and innervation of skeletal muscle. • Discuss the origin of muscle fibers and explain how muscle hypertrophy occurs. • Produce diagrams that illustrate the arrangement of myofilaments in a sarcomere. • Describe how the sliding filament model explains the contraction of muscle fibers. 9-73 Ch. 9 Learning Objectives • Describe the resting membrane potential, and how it is generated and maintained. • Explain the role of ion channels in the production of an action potential. • Discuss the production of an action potential, including depolarization and repolarization. • State the all-or-none principle as it pertains to action potentials. • Describe the structure of a neuromuscular junction, and explain how an action potential is transmitted across the junction. • Explain the events of excitation-contraction coupling. • Summarize the events of cross-bridge movement and relate them to muscle contraction. • State the conditions needed for muscle relaxation. • Describe a muscle twitch, and the events that occur in each phase of a twitch. 9-74 Ch. 9 Learning Objectives • Describe a motor unit, and how motor unit number affects muscle control. • Explain how whole muscles respond in a graded fashion, and how the force of contraction can be increased. • Relate recruitment to multiple motor unit summation. • Describe incomplete tetanus and complete tetanus. • Distinguish between isometric and isotonic contractions. • Relate how muscle tone is maintained. • Compare the mechanisms involved in the major types of muscle fatigue. • Describe the three sources of energy to produce ATP for muscles. • Distinguish between oxygen deficit and recovery oxygen consumption. 9-75 Ch. 9 Learning Objectives • Distinguish between fast-twitch and slow-twitch muscle fibers. • Describe how training can increase the size and efficiency of both types of muscle fibers. • Describe how muscles respond to changes from normal body temperature. • Describe the structural features of smooth muscle cells, and contrast them with skeletal muscle fibers. • Explain the steps of smooth muscle contraction, and how the contraction differs from skeletal muscle contraction. • Describe the electrical and functional properties of smooth muscle. • Explain how smooth muscle activities are regulated. • Discuss the structural and functional characteristics of cardiac muscle. • Describe the changes that occur in aging skeletal muscle. 9-76