NURS 207 (N01) Skeletal Muscle PDF
Document Details
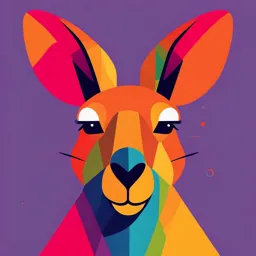
Uploaded by StrongerLove5832
Beal University
2024
Dr. P. Lee
Tags
Summary
This document is lecture notes on skeletal muscle, covering the sliding filament mechanism, the contraction-relaxation cycle, and muscle metabolism. It includes objectives and sample questions.
Full Transcript
NURS 207 (N01) Skeletal muscle Sliding filament mechanism, contraction-relaxation cycle, and muscle metabolism October 21, 2024 Dr. P. Lee Objectives: 1) Know the detail of sliding filament mechanism for muscle contraction and...
NURS 207 (N01) Skeletal muscle Sliding filament mechanism, contraction-relaxation cycle, and muscle metabolism October 21, 2024 Dr. P. Lee Objectives: 1) Know the detail of sliding filament mechanism for muscle contraction and the contraction- relaxation cycle 2) Know the detail of excitation-contraction coupling 3) Know the muscle metabolism with respect to the production of ATP Sliding filament mechanism for muscle contraction and contraction-relaxation cycle Contraction of skeletal muscle fiber Crossbridge formation The interlocking of myosin heads and G-actins between the parallel thick and thin filaments → Meaning that myosin heads of the thick filaments can bind to the myosin binding sites located in the G-actins of the thin filaments Titin Z disk Z disk M line Myosin crossbridges Crossbridge formation is the driving force behind the muscle contraction Skeletal muscle fiber Actin & Myosin Myosin Actin Crossbridge formation Contraction of skeletal muscle fiber Crossbridge formation Crossbridges have two states: ✓ Low-force i.e. When muscle is relaxing, but maintaining its resting muscle tone ✓ High-force i.e. When muscle is contracting Titin Z disk Z disk M line Myosin crossbridges Some degree of crossbridge formation is observed in muscle during relaxing state (low-force state) Contraction of skeletal muscle fiber Crossbridge formation In skeletal muscle, the troponin C (TnC) has two pairs of Ca2+-binding sites: ✓ Two high-affinity sites are always occupied by Ca2+ under physiological conditions ✓ Two low-affinity sites bind and release Ca2+ as cytoplasmic [Ca2+] rises and falls, respectively Contraction of skeletal muscle fiber Crossbridge formation The high-affinity sites are termed structural sites with sustained activation by constant Ca2+ binding → Under relaxed conditions (with low cytoplasm Ca2+ concentration), Ca2+ binds preferentially to the TnC high-affinity sites ✓ Without binding of Ca2+ to the low-affinity sites located at TnC: ▪ Most of the TnI will remain bind to the actin ▪ Or most of the myosin binding site located at the actin will be covered by the TnI attached to the tropomyosin Contraction of skeletal muscle fiber Crossbridge formation Binding of Ca2+ to low-affinity sites induces a conformational change in the troponin complex yields two effects : 1) Weakens TnI attachment to its binding sites on actin’s regulatory unit, permitting the tropomyosin molecule (Tm) to move 2) Exposes myosin-binding sites on actin, allowing the interaction between myosin and actin to engage in cross-bridge formation Crossbridge formation of skeletal muscle fiber (summary) Crossbridge formation Ca2+ exerts its effect on crossbridge formation by binding to regulatory proteins (TnC) rather than directly interacting with contractile proteins In the absence of Ca2+ binding to the low-affinity sites on the TnC, the regulatory proteins act in concert to inhibit actin-myosin interaction When Ca2+ binds to the low-affinity sites on the TnC: ✓ It induces conformational change in the regulatory complex ✓ Exposes the myosin binding sites located on actin ✓ Allows interaction between actin and myosin Contraction-relaxation cycle of skeletal muscle fiber Sliding filament theory of contraction Muscle contraction occurs when the thick and thin filaments slide past each other, with thin filaments move toward the M line (mid-line of the sarcomere) As sarcomere shortens during contraction, Z lines move closer towards each other and shortening both H zone and I band while A band remains constant I band A band Muscle Relaxed Myosin Actin Sarcomere Half of Half of Z line H zone shortens with I band I band contraction Original location Muscle Contracted H zone and I band both Final shorten, while A band remains constant I H I location Contraction of skeletal muscle fiber Sliding filament theory of contraction Muscle contraction and relaxation cycle involves the interaction between the actin and myosin of the thin and thick filaments, respectively It also involves the bounding and releasing of nucleotides [such as ATP (adenosine triphosphate), ADP (adenosine diphosphate)], and Pi (inorganic phosphate) to and from the myosin head Contraction of skeletal muscle fiber Sliding filament theory of contraction When an ATP molecule bounds to the myosin head, myosin head will detach itself from the thin filament (actin) and the muscle is in relax state Detachment of myosin ATP bind head from the G-actin after the binding of ATP to the myosin head Myosin will remain in its detached position if its head is still bound by ATP (even after the binding of Ca2+ to the low-affinity sites on TnC and exposing the myosin binding site located on G-actin molecule) Contraction of skeletal muscle fiber Sliding filament theory of contraction After ATP is hydrolyzed to ADP and inorganic phosphate (Pi), the myosin head rotates to the “cocked back” position and binds to the actin’s myosin binding site, forming a crossbridge TroponinG-actin TN Myosin head ATP binds. Tropomyosin Pi ADP “cocked back” position Contraction of skeletal muscle fiber Sliding filament theory of contraction Inorganic phosphate (Pi ) is then dissociated from the myosin head, causing the myosin head to rotate 45o and to perform a power stroke, pulling the thin filament along with it (sliding filament theory) and resulting the shortening of sarcomere → contraction It pulls the actin filaments Troponin G-actin towards the M line of the sarcomere, shortening the sarcomere length TN TN Myosin head Tropomyosin ADP Pi ADP Myosin head rotates Pi 45o from its cocked position after the “cocked back” dissociation of Pi Power stroke position from the myosin head Contraction of skeletal muscle fiber Sliding filament theory of contraction Once the power stroke is finished, ADP leaves the myosin head ✓ Myosin head will remain attach with the actin in a contracted state until a new ATP is bound to the myosin head In the absence of ATP, muscle will be in a contracted (rigor) state, characteristic of rigor mortis (stiffening of the dead body) Contraction of skeletal muscle fiber Sliding filament theory of contraction When a new ATP molecule is bound to the myosin head after contraction, the myosin head will detach itself from the actin and to restore itself back into its initial position (detachment of the crossbridge) i.e. the muscle is back to the relax state ATP binds. Contraction of skeletal muscle fiber Tight Binding in the Rigor State G-actin molecule Myosin binding sites Myosin releases ATP binds to myosin. ADP at the end Myosin filament Myosin releases actin. of the power stroke. ATP binds. ADP releases. Myosin hydrolyzes ATP. Energy from Contraction- relaxation ATP rotates the The releases of Pi myosin head to the enable the myosin The Power Stroke Sliding filament cocked position. Actin filament moves toward M line. head to swivel, Myosin binds to actin. generating the power stroke. Head ADP swivels. Pi Myosin ADP and Pi releases Pi. remain bound. Contraction of skeletal muscle fiber The contraction cycle repeats itself if: ✓ Another electrical signal is sent from the somatic motor neuron to activate the muscle unit ✓ Release of Ca2+ from the sarcoplasmic reticulum (SR) to elevate the cytosolic Ca2+ levels ✓ ATP is available The myosin heads will keep rotating back and forth with each power stroke, pulling the thin filaments toward the M line Contraction of skeletal muscle fiber Muscle contraction-relaxation cycle is a direct result from an attachment–detachment cycle between the myosin heads and actins Tension generated in a muscle fiber is directly proportional to the number of crossbridges form between the thin and thick filaments Excitation-contraction (EC) coupling Contraction-relaxation cycle of skeletal muscle is regulated by troponin, and is closely associated with the binding of Ca2+ to TnC : ✓ Contraction begins in response to the increase in sarcoplasmic Ca2+ concentration ✓ Relaxation occurs when sarcoplasmic Ca2+ levels decreases i.e. [Ca2+], not the action potential, is the signal for muscle contraction and relaxation The process by which electrical excitation of the surface membrane in muscle triggers an increase of [Ca2+]i is known as excitation-contraction coupling Excitation-contraction (EC) coupling There are 4 main events in excitation-contraction coupling: 1) Ach is released from the somatic motor neuron 2) Ach initiates an action potential in the muscle fiber 3) Action potential travels along the sarcolemma into the T-tubules and triggers Ca2+ release from the sarcoplasmic reticulum (SR) 4) Ca2+ binds with TnC and initiates muscle contraction Excitation-contraction (EC) coupling Sequence of events for excitation-contraction coupling: Somatic motor neuron Axon terminal of somatic releases ACh at motor neuron neuromuscular junction Muscle fiber ACh ACh binds to receptor on ial Action pot Na+ the motor end plate Motor end plate RyR (muscle fiber) T-tubule Ca2+ Sarcoplasmic Binding of Ach to the reticulum Z disk DHP Troponin receptor activates the Na+ Actin Tropomyosin M line Myosin head Myosin thick filament channel, increases the permeability of Na+, initiates an action potential that travels along sarcolemma of the muscle fiber Excitation-contraction (EC) coupling Sequence of events for excitation-contraction coupling: Muscle action potential travels along T-tubule RyR the sarcolemma into DHP Ca2+ released in t-tubule and initiates conformation changes Myosin thick filament of DHP receptor Distance actin moves DHP = dihydropyridine receptor DHP receptor opens RyR (L-type calcium channel) (Ca2+ release channel) in RyR = ryanodine receptor (Ca2+- release channel) sarcoplasmic reticulum, and releasing Ca2+ into cytoplasm Excitation-contraction (EC) coupling Sequence of events for excitation-contraction coupling: Ca2+ binds to troponin C T-tubule RyR (TnC), allowing actin- DHP myosin binding Ca2+ released Myosin heads execute Myosin thick filament power stroke Distance actin moves DHP = dihydropyridine receptor Actin filament slides (L-type calcium channel) RyR = ryanodine receptor toward center of (Ca2+- release channel) sarcomere (contraction) To end a contraction, Ca2+ must be removed from the sarcoplasm Excitation-contraction (EC) coupling Sequence of events for excitation-contraction coupling: SR pumps Ca2+ back into its lumen using sarcoplasmic Ca2+-ATPase, also called 2+ Ca releases ATP Ca2+ sarco-endoplasmic reticulum Myosin thick filament calcium ATPase (SERCA) Distance actin moves As the cytosolic free Ca2+ concentration decreases, Ca2+ is released from troponin C (TnC) Release of Ca2+ from TnC allows tropomyosin to slide back to its inhibition state and blocks the actin’s myosin binding site (relaxation) Myosin heads is released (after ATP binding), elastic elements pull filaments back to their relaxed position Timing of excitation-contraction coupling ❖ Electrical comes before mechanical Motor Neuron Action Potential Short delay Muscle fiber +30 Neuron between the Action potential from CNS membrane potential in mV muscle action −70 Time potential & the Motor end plate Recording electrodes Axon Muscle Fiber Action Potential beginning of terminal +30 muscle tension Muscle fiber Muscle action membrane potential potential in mV development −70 2 msec Time Tension is the magnitude of a Development of Tension During One Muscle Twitch force Relaxation Contraction phase Latent phase Muscle tension is the pulling force Tension period exerted by muscle to counteract or 10–100 msec Time move a load which requires energy Timing of excitation-contraction coupling Action potential Tension Sarcoplasmic Ca++ → This short delay (latent period) represents the time required for the binding of calcium to troponin and the initiation of crossbridges formation Muscle metabolism ❖ ATP is required to power the muscle contraction cycle Availability of ATP reserve within the skeletal tissues at resting state can only sustain muscle contraction for a few seconds There are 3 additional ways for the muscle fibers to extract more ATP: 1) From creatine phosphate 2) By anaerobic cellular respiration 3) By aerobic cellular respiration Creatine phosphate Also known as phosphocreatine (PCr) → Most of the excess ATP within the muscle fibers during resting state is being used to synthesize creatine phosphate ATP is required for muscle contraction Excess ATP ATP in demand (resting state) (exercising) Tight Binding in the Rigor State G-actin molecule Myosin releases ADP at the Myosin Myosin releases actin after end of the power stroke binding sites ATP binds to myosin Myosin filament ATP binds Contraction- relaxation The Power Stroke Actin filament moves toward M line. Sliding filament Head swivels Ca2+ ADP (power stroke) Power stroke signal Pi ADP and Pi remain bound. begins when tropomyosin moves off the binding site. Myosin hydrolyzes ATP into Pi Myosin releases Pi and ADP using ATPase and rotates the myosin head to the cocked position Creatine phosphate Creatine phosphate is 3 to 6X more plentiful than ATP in the sarcoplasm of a relaxed muscle fiber → At rest, the enzyme creatine kinase (CK), also known as creatine phosphokinase (CPK), catalyzes the transfer of one phosphate (Pi) from ATP to creatine in forming creatine phosphate and ADP → During muscle contraction or with high level of ADP, CK catalyzes the transfer of phosphate (Pi) from creatine phosphate back to ADP and to form ATP (process known as phosphorylation) CK PCr + ADP Creatine + ATP Creatine phosphate also known as phosphocreatine (PCr) Creatine phosphate Formation of ATP from creatine phosphate occurs very rapidly and is the 1st source of additional energy at the beginning of the muscle contraction → The initial amount of ATP plus those released from the creatine phosphate provide enough energy for muscles to contract maximally for about 15 seconds Anaerobic cellular respiration A process of ATP production requires no oxygen → Glucose is used to generate 2 molecules of ATP in the absence of oxygen during heavy exercising → Glucose from either the blood through facilitated diffusion or catabolization of glycogen (glycolysis) within muscle fibers Anaerobic cellular respiration Normally, pyruvic acid will enter into the mitochondria to produce more ATP if oxygen is available → With the lack of oxygen during heavy exercise, pyruvic acid will convert to lactic acid → About 80% of the lactic acid produced will diffuse back to the blood circulation and is converted back to glucose in liver → ATP produced by muscle fibers anaerobically can sustain muscle contraction maximally for about 30 to 40 seconds Aerobic cellular respiration Occurs during rest or light to moderate exercise With adequate oxygen supply to the contracting muscle fibers by: → Circulating blood (with hemoglobin as the oxygen-binding protein) → Myoglobin in the muscle (oxygen-binding protein in muscle fibers) Aerobic cellular respiration → With adequate supply of oxygen, pyruvic acid enters the mitochondria where it is completely oxidized to produce 36 molecules of ATP, CO2, H2O, and heat → Substrates utilized by the mitochondria within the muscle fibers for aerobic ATP production include pyruvic acid from glycolysis, fatty acids from adipose tissues, and amino acids from protein breakdown → ATP produced by muscle fibers aerobically can sustain muscle contraction maximally for minutes to hours Aerobic cellular respiration Oxygen debt Oxygen debt refers to the additional oxygen, over and above the resting oxygen consumption, that is taken into the body after exercise → Heavy breathing and elevated blood pressure above the resting rate continue for some time after exercise Oxygen debt The extra oxygen acquired is to: 1) Convert lactic acid back into glycogen for storage 2) Resynthesize PCr (phosphocreatine or creatine phosphate) and ATP in muscle fibers 3) Replace the oxygen removed from myoglobin 4) Sustain the increase rate of chemical reactions resulting the elevated body temperature 5) Sustain the extra work performs by organs, such as heart and lungs, after exercise has stopped 6) Allow the extra energy consumption for tissue repair Sample questions 1) Contraction of myofibrils within a muscle fiber begins when: a) sodium enters the muscle fiber. b) acetylcholine binds to ligand gates. c) calcium is released from the sarcoplasmic reticulum. d) an action potential travels t-tubules. 2) Which correctly lists the sequence of structures that action potentials must move through to excite skeletal muscle contraction? a) sarcolemma, somatic neuron, T tubules. b) T tubules, sarcolemma, myofilament. c) muscle fiber, somatic neuron, myofibrils. d) somatic neuron, sarcolemma, T tubules. Sample questions 3) Skeletal muscle contraction is triggered when calcium is released from: a) myofibrils. b) mitochondria. c) terminal cisterns of sarcoplasmic reticulum. d) T-tubules. 4) During muscle contractions , thin filaments are pulled towards the: a) Z disc. b) H zone. c) M line. d) A band. Sample questions 5) What energizes the myosin head? a) Acetylcholine. b) Calcium ions. c) Phosphate release. d) ATP hydrolysis reaction. 6) ____ is released into the neuromuscular junction. It then binds to the _____ to stimulate the entering of ____ into the muscle fiber cell, which then initiates the generation of an ____ that travels alone the _____ deep into the muscle fiber. a) ACh, Ach receptor, sodium ions, action potential, t-tubule. b) Calcium ions, DHP receptor, Ach, action potential, t-tubule. c) Sodium ions, RyR receptor, Ach, action potential, t-tubule. d) ACh, DHP receptor, calcium ions, action potential, t-tubule. Sample questions 7) Concentration of creatine phosphate: a) is constant at all time for any given muscle. b) is the same inside the muscle for all 3 types of muscle. c) is at its highest for a given muscle during exercise. d) decreases when there is a high demand for ATP. 8) Which enzyme catalyzes the transfer of a phosphate group from creatine phosphate back to ADP and to form ATP? a) Creatinase. b) Phosphatase. c) Creatine kinase. d) ATPase. Sample questions 9) Majority of the lactic acid produced during anaerobic cellular respiration will: a) be converted back to glucose in the liver. b) enter into mitochondria and convert back to glucose. c) be converted into urine by the kidneys. d) be eliminated by the lungs. 10)Myoglobin: a) is the end product of anaerobic cellular respiration. b) has the ability to bind to oxygen. c) is most abundance in fast-twitch glycolytic fibers. d) is the main component within the red blood cells. Answer to sample questions 1) c 2) d 3) c 4) c 5) d 6) a 7) d 8) c 9) a 10)b