Corrosion Prevention by Protective Coatings PDF 3rd Edition
Document Details
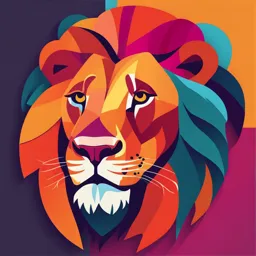
Uploaded by RenewedSymbol8188
2014
Louis D. Vincent
Tags
Related
- Corrosion Chemistry Notes PDF
- Corrosion Principles and Anti-Corrosion Treatments in Vehicles PDF
- Module 5.1 Corrosion Prevention and Control PDF
- Engineering Materials Science and Engineering 7 Corrosion and Control PDF
- Material Engineering & Testing: Corrosion Prevention and Control PDF
- Corrosion Notes - Applied Chemistry PDF
Summary
This book details corrosion prevention techniques using protective coatings. It is a third edition, updated for current technologies and practices.
Full Transcript
Corrosion Prevention by Protective Coatings Third Edition Charles G. Munger Louis D. Vincent, Ph.D. Associate Author ©2014 NACE International i NA...
Corrosion Prevention by Protective Coatings Third Edition Charles G. Munger Louis D. Vincent, Ph.D. Associate Author ©2014 NACE International i NACE International ©1984, 1999, 2014 NACE International All rights reserved. First edition 1984. Second Edition 1999. Third edition 2014. Printed in the United States of America. ISBN 1-57590-262-1 Reproduction of contents in whole or part or transfer into electronic or photographic storage without permission of copyright owner is expressly forbidden. Neither NACE International, its officers, directors, nor members thereof accept any responsibility for the use of the methods and materials discussed herein. No authorization is implied concerning the use of patented or copyrighted material. The information is advisory only, and the use of the materials and methods is solely at the risk of the user. Acknowledgments It is amazing how much a person can learn from colleagues in the wonderful world of protective coatings. Such has been the case as I set out once more to update and expand the NACE International book, Corrosion Prevention by Protective Coatings. I would be remiss in my duties if I did not mention several people who have been a tremendous influence on my career and in particular in providing valuable information and technology for this Third Edition of what is commonly known as The Munger Book. First of all is Charles G. Munger, “Chuck” as he is fondly remembered. He was my mentor when I first started out in the protective coatings industry, as well as a true friend. Carl Angelof and Todd Williams of Bayer Material Sciences for some much needed new information on polyurethanes, polyureas, and polyaspartics. Pete Englebert of Job Safety Associates, Inc. for his excellent revision and update of Chapter 16, “Safety.” Hasan Sabri of Kuwait Oil Company for almost single handedly guiding me through the creation of the new Chapter 23, “Pipeline Coatings and Linings.” Terry Greenfield of Corrometric Services, Inc. for his excellent Chapter 21, “Computer-Assisted Coatings Project Management Programs.” John Kelly of Akzo Nobel for all his expertise in anti-fouling and foul release coatings contained in Chapter 22, “Coatings for Marine Vessels.” Alex Szokolic and R.A. Francis, Australian colleagues, for their broad knowledge of the history and development of inorganic zinc coatings. All of the students in NACE Coating Inspector Program (CIP) and Protective Coatings Specialist courses that I have taught over the years. I learned so much from their real-world practical experience. Last, but certainly foremost in my thoughts, is Mary Ann Vincent, my lovely wife since 1952 for her love and patience when I was cranky and short-tempered while grinding away at the computer on this, my last update and revision of Corrosion Prevention by Protective Coatings. It is my fervent hope that someone will take up the gauntlet at some point in the future to make new updates and revisions as new coatings technology becomes a reality. NACE International 15835 Park Ten Place, Houston, TX 77084 +1 281-228-6200 www.nace.org ii Author’s Preface for the Third Edition of Corrosion Prevention by Protective Coatings There has been a tremendous increase in the introduction of new technology in the coatings industry since the publication of the second edition of Corrosion Prevention by Protective Coatings in 1999. That has been the driving force behind my desire to update Charles G. Munger’s original work once again in order to keep his pioneering work alive as a valuable reference work for anyone involved in the prevention of corrosion by the proper use of protective coatings. For the guidance of the reader of this third edition of the book, I have included references to new or improved technologies in the following chapters: Chapter 1: Protective Coating Specialist certifications, waterjetting, smart coatings Chapter 2: Updates of NACE standards SP0198-2010, “Corrosion Control Under Thermal Insulation and Fireproofing Materials,” and SP0178-2007, “Design, Fabrication and Surface Finish Practices for Tanks and Vessels to be Lined for Immersion Service” Chapter 4: IMO Performance Standard for Protective Coatings (PSPC) regulations, polyaspartic resins, nano-sized pigments, the International Convention on the Control of Harmful Anti-fouling Systems on Ships (AFS) banned the use of tin-containing anti-fouling coatings in 2001 Chapter 5: Polyaspartic resins; nano-sized pigments; latest technology in polyurethanes, polysiloxane, and polyureas. Chapter 6: High ratio waterborne inorganic zinc, updated ASTM standards Chapter 7: A new (2011) two-component, high-build, high-temperature resistant “cold spray” applied coating based on titanium modified inorganic copolymer technology pigmented with aluminum flake that is capable of providing corrosion protection under thermal insulation operating in thermal cycling conditions between –321 °F (–196 °C) and 752 °F (400 °C) without the need of heat curing Chapter 9: Waterjetting, updated NACE/SSPC surface preparation standards Chapter 11: International Concrete Repair Institute (ICRI) Guideline No. 310.2 (1997; reapproved 2002) Chapter 12: The International Convention on the Control of Harmful Anti-fouling Systems on Ships (AFS) banned the use of tin-containing anti-fouling coatings in 2001; updated ASTM and ISO standards Chapter 16: An update on Occupational Safety & Health Administration (OSHA) safety regulations Chapter 19: Coatings under thermal insulation Chapter 21: A total update of computer-assisted coatings project management Chapter 22: A completely new chapter on marine coatings including new anti-fouling and foul release coatings introduced in 2013 Chapter 23: A completely new chapter on pipeline coatings focusing on modern fusion bonded epoxies, polyethylenes, and polypropylenes v Contents Acknowledgments ii Preface v 1 Introduction to Corrosion 1 Introduction Historical Background Coating Market Projections Coatings Technologies Coatings Economics Coating Manufacture Other Coating Terms Coatings Complexities and Variables Types of Coatings The Finished Product The Development of Protective Coatings The Future of Protective Coatings 2 Corrosion as Related to Coatings 19 Corrosion of Materials Other Than Metal Early Corrosion Studies Fundamentals of Corrosion Galvanic Corrosion Chemical Corrosion Mill Scale Filiform Corrosion Pitting Corrosion Corrosion Under Insulation Atmospheric Corrosion Methods of Corrosion Control 3 Essential Coating Characteristics 47 Coating Function Essential Coating Properties Chemical Resistance Proper Adhesion Abrasion Resistance Ability to Expand and Contract Weather Resistance Resistance to Dirt Pickup Resistance to Bacteria and Fungus Pleasing Appearance Age Resistance Easy Application Additional Coating Properties Types of Exposure Measurable Coating Characteristics 4 Coating Fundamentals 63 Basic Coating Concepts The Coating System Basic Coating Formation Coating Component Functions Basic Coating Components 5 Corrosion-Resistant Organic Coatings 89 Natural Air-Oxidizing Coatings Synthetic Oxidizing Coatings Vinyl Organisols and Plastisols Co-Reactive Coatings Heat- Condensing Coatings 100% Solids Coatings 6 Corrosion-Resistant Zinc Coatings 127 Protection by Zinc Coatings Application of Zinc Coatings Zinc Dust Coatings Characteristics of Organic Zinc-Rich Coatings Inorganic Zinc Coatings Development of Inorganic Zinc Coatings Composition of Inorganic Zinc Coatings Pigmentation Types of Inorganic Zinc Coatings Advantages of Inorganic Zinc Preconstruction Primer Single Package Inorganic Zinc Coatings Characteristics of Inorganic Zinc Coatings Types of Zinc-Rich Coatings Comparison of Zinc-Rich Coatings Topcoating Comparison Summary Annex 6A: A Short History of Water-Based High-Ratio Potassium Silicate Inorganic Coatings 7 Structural Design for Coating Use 171 Principle of Design for Coating Use Interior and Exterior Design Coating Problems Related to Design 8 The Substrate—Importance of Coating Life 187 Types of Substrates Types of Contamination 9 Surface Preparation 199 Types of Adhesion Surface Preparation Objectives Development of Surface Preparation Techniques Types of Contamination Types of Surface Preparation Concrete Surfaces Other Influences in Surface Preparation 10 Application of Coatings 237 Type of Coating Preparation for Coating Application Application Methods Application Problem Areas Cost of Application Annex 10A: Training and Certification of Application Specialists 11 Coatings for Concrete 277 Introduction Properties of Concete Composition of Concrete Problems in Coating Concrete Properties Required for Coatings Used on Concrete Reasons for Coating Concrete Types of Coatings for Concrete Surface Preparation of Concrete Prior to Coating iii 12 Coating Selection 293 Introduction Considerations in Coating Selection Evaluating Operating Conditions 13 Coatings and Cathodic Protection 313 Introduction Cathodic Protection Coatings Consequences of Poor Coating Selection Testing 14 Coating Failures 333 Introduction Specification-Related Failures Formulation-Related Failures Inorganic Coatings Failures Due to Coating Selection Repairing and Recoating Substrate-Related Failures Surface Preparation-Related Failures Application-Related Failures Design-Related Failures Adhesion-Related Failures Failures Due to Exterior Forces 15 Coating Repair and Maintenance 361 Introduction Primary Repair Considerations Repair of Failures Repair of Coatings Appendix 15A: Procedures for Adhesion Test 16 Safety 387 Introduction Changes in the Coating Industry Primary Hazards Health Hazards Appendix 16A: References—Safety and Environmental Control Appendix 16B: A Manual for Painter Safety 17 Specifications 423 Parts of a Specification Appendix 17A: Typical Offshore Work Specification 18 Inspection and Training 443 Variables Involved in Quality Control Types of Coating Inspectors What Should a Qualified Inspector Know? Areas of Coating Inspection Other Equipment 19 Typical Uses of High-Performance Coatings 463 The Chemical Industry Inorganic Chemical Plants Organic Chemical Plants Pulp and Paper The Mining Industry The Steel Industry The Power Industry The Food Industry Sewage Treatment The Transportation Industry Coatings Under Thermal Insulation 20 Elastomeric (Rubber) Linings 493 Curatives and Vulcanizing Pigments Types of Rubber Linings Vessel Design Surface Preparation Cure Repairs Safety Reference Documents and Standards 21 Computer Assisted Coatings Program Management 497 Introduction The Process Management Systems Describing the Program Facilities Grading Systems Bringing Data Together for Management Action Planning and Budgeting Monitoring Effectiveness of Corrosion Prevention Systems 22 Coatings for Marine Vessels 507 Introduction Abrasion-Resistant Coatings Antifoulings and Foul Release Coatings Cargo Tank Linings Ballast Tank Linings Cofferdam and Void Coatings Deck Coatings—Including Heli-Deck Surfaces Hull Coatings—Freeboard Area Potable Water Tank Linings Shop Primers Universal Primers Zinc-Rich Coatings Organic Primers and Tie-Coats Heat-Resistant Coatings Spray Metallizing Process (Thermal Spray) Maintenance Painting Programs Shipboard Corrosion Assessment Training (S-CAT) 23 Pipeline Coatings and Linings 519 Introduction Desirable Characteristics of External Pipeline Coatings Typical Factors to Consider When Selecting an External Pipeline Coating Coating Systems for Buried Pipes Fusion-Bonded Epoxy (FBE) Three-Layer High-Density Polyethylene/ Polypropylene High-Building Polyurethane Coatings High-Build Epoxy Coatings Coating Application—Field Joints Coating of FBE Joints and Fittings Underground Pipe Hot Polyurethane Foam Insulation Index 537 iv Introduction to Corrosion Introduction TABLE 1.1: NACE International Education and Training Courses as of 2014 Corrosion and corrosion engineering constitute a science that has Basic Corrosion only developed within the lifetime of many people working in the field today. This does not mean that corrosion is strictly a Basic Internal Corrosion for Pipelines modern phenomenon, for it has, on the contrary, existed since Chemical Treatment Specialist humans first discovered ways to make metal from ores. It does, Coating Inspector Program (CIP) Level 1 however, mean that prior to 1950, the existence of corrosion CIP Level 2 was passively accepted, along with death and taxes, as inevita- CIP Level 2—Maritime ble. In fact, many plant managers in this early period insisted that there was no corrosion in their plants; a little rust, which CIP One-Day Bridge eventually called for the replacement of the plant’s structures CIP Level 3 Peer Review maybe, but never any corrosion. Such evasive attitudes, however, Coatings in Conjunction with Cathodic Protection (CP) have changed over the years due to a better understanding of Corrosion Control in the Refining Industry the subject and increased awareness of replacement economics. Corrosion Specialist More recently, the impact of ever more stringent government regulations regarding worker safety and environmental protec- Corrosion in the Water and Wastewater Industry (online training) tion have increased the cost of protective coatings maintenance, CP Interference which in turn has heightened the awareness of the economic CP 1—Cathodic Protection Tester impact of improperly designed and applied coating systems even CP 2—Cathodic Protection Technician further. This, in turn, has created an increasing demand for cor- CP 2—Cathodic Protection Technician-Maritime rosion prevention specialists, now known as corrosion engineers. In the protective coatings industry, the growing awareness of the CP 3—Cathodic Protection Technologist critical function that coatings play in the prevention of corrosion CP 4—Cathodic Protection Specialist has led to continuing education courses with the certification of Designing for Corrosion Control the successful participants as Protective Coatings Specialists. Direct Assessment—Pipelines In the past, there was no formal training for corrosion engi- In Line Inspection—Pipelines neers. They developed into specialists from their various roles as chemists; metallurgists; physicists; and chemical, civil, mechan- Internal Corrosion for Pipelines—Basic ical, maintenance, industrial, and piping engineers; as well as Internal Corrosion for Pipelines—Advanced from their roles as experts in petroleum refining; petrochemi- Introduction to Coating Inspection (online training) cals; paper, water, sewage, and ship operation; and other indus- Marine Coating Technology trial operations that are adversely affected by corrosion. While Materials Selection/Design Specialist today there are some graduates with corrosion engineering courses as part of their degree programs, the majority were still Nuclear Power Plant Training for Coating Inspectors initially trained in other engineering sciences and have come Offshore Corrosion Assessment Training (O-CAT) to specialize in this field because of their experiences with cor- Pipeline Coating Applicator Training rosion and their developing interest in its prevention. Despite Pipeline Corrosion Assessment Field Techniques (P-CAFT) their interest and expertise in the field of corrosion, and as a Pipeline Corrosion Integrity Management consequence of their training in other disciplines, the science and technology of coatings is generally foreign to them. This, Protective Coating Specialist (PCS) 1—Basic Principles however, is understandable given that high performance coat- PCS 2—Advanced ings represent a relatively recent (i.e., prior to 1950) and special- PCS 3—Management ized branch of a much broader, established field. Pipeline Corrosion Assessment Field Techniques NACE International has lead the way in providing continuing Pipeline Corrosion Integrity Management (PCIM) education courses for corrosion engineers and personnel of all disciplines with the courses listed in Table 1.1. Shipboard Corrosion Assessment Training (S-CAT) Introduction to Corrosion 1 FIGURE 1.1: A painting found in an Egyptian tomb reveals their use of FIGURE 1.2: An Egyptian painting illustrating that most paints and pig- synthetic blue pigment along with natural earth tones. ments of this era were used for decorative purposes. While the general area of coatings (or paint) has only come into its own as an industry during the Modern Age (post-World War II era), the discovery and development of materials used by that industry have it roots in more primitive times. A general survey, there- fore, of the emergence of such materials may serve as a practical prelude to the development of today’s more specialized protective coatings, especially since some of the early materials and even attitudes have carried over into the present industry. The following historical analysis can thus provide a valuable background from which to evaluate many of the materials, procedures, and processes common in today’s protective coating field. Historical Background Thousands of years before their protective qualities were discovered, coatings were used for decorative and identification purposes. The earliest known paintings, which were found in caves in France and Spain, were made from naturally occurring iron oxides.1 These were apparently applied without a binder by merely rubbing the iron oxide into the surface of a cave’s interior. This, then, was the primitive basis for the subsequent development in paints, which took place primarily in two separate areas of the world. Early Materials Egypt developed the first synthetic pigment, which was known as Egyptian Blue, and was made from lime, sand, soda ash, and copper oxide heated together and ground to a relatively fine powder. This was developed in addition to a number of natural pigments that were already in use2-4 (Figures 1.1 and 1.2). At a somewhat later date, the Egyptians (as well as the Chinese who earlier developed calcined or fired pigments and some organic pigments(1)) began the use of additional paint vehicles. These included gum Arabic, egg white, gelatin, beeswax, and glue. This led to the Egyptian development of the first actual protective coating, which involved the use of various pitches and balsams to coat their ships.1,4 The Chinese, as well as the Koreans and Japanese, used a lacquer for the decoration of buildings, instruments, and weapons.1 Not much is known as to whether this early lacquer was of one type of material or several. It is known, however, that at some point, the Japanese developed a coating made from the sap of a “varnish tree.”4 This was used in much of their early decorative painting and in the lacquer ware that we know most about today (e.g., antique trays, vases, boxes, and small bottles and containers). The original sap required cleaning and filtering and was quite poisonous (similar to poison sumac and poison ivy or oak in its effects). When applied in relatively thin layers and kept in a damp, humid atmosphere, it cured or polymerized into a dense, high-gloss coating, which had excellent resistance to aging and no poisonous effects. 2 Introduction to Corrosion The Romans, during this same era, learned the techniques of mak- ing paint from the Egyptians, many examples of which can still be seen in the ruins of Pompeii. While the Romans used essentially the same materials as the Egyptians, they also developed several artificial colors. These materials were primarily lead based since the Romans had extensively developed the technology of mining and extracting lead from ores. The Romans also copied the Egyptians in the use of a protective coating for their vessels, adding wax to the Egyptian pitch combination.4 The American Indians of the Canadian West Coast used a variety of organic materials for their pigments. These includ- ed charcoal, lampblack, graphite, and powdered lignite for black; diatomite mined from the bottom of shallow lakes, or calcined deer bones or antlers for white; calcined yellow ocher or roasted hemlock fungus for reds; yellow ocher or ground hemlock fungus for yellow; and copper carbonates and Peziza (the mycelium of a fungus) to prepare blues and greens.4 Salmon eggs, both fresh and dried, were used for vehicles, which were prepared by chewing the eggs with the bark of red cedar. Fish oil from eulachon (Thaleichthys pacificus) or from perch was also used. Boiling the singed skins of mountain goats made sizes or mixing saliva with Peziza fungus, and mountain goat fat was used as the vehicle for their cosmetics.4 The early American Indians also made rock paintings, or pictographs, which have lasted for centuries (Figure 1.3). These were all done in earth colors and made mostly from iron oxide, which was probably rubbed into the rock with fingers and twigs.4 Burma and Thailand were the original producers of shellac, which is possibly the oldest known clear finish. The shellac resin, which is still imported from there today, is made by the lac bug, an insect that during its life cycle attaches to small branches of a variety of the fig tree. This insect converts the tree’s sap into a brittle reddish-brown resin with which it covers itself. The resin is gathered, crushed, washed, and bleached for use by the present paint industry. In its pure state, it is colorless, odorless, and edible. Emerging Technology The late eighteenth century saw the slow emergence of the paint FIGURE 1.3: In this centuries-old Algonquian pictograph, red pigment and varnish industry. Although an actual varnish factory was report- (finely ground iron oxide) is mixed with vegetable oil, blood, and egg white. edly established in England at this time,4 paint was still made by in- Source: America’s Fascinating Indian Heritage (Pleasantville, NY: Reader’s dividuals in small volumes for their own use and according to their Digest Association, Inc., 1978), p. 140. own method. Much of what was made adhered primarily because of the affinity of the pigment for the surface, and much of it could be rubbed from the surface with a wet finger. By the early 19th century, some power-driven equipment was used to manufacture paint. These manufacturers, however, only used the equipment to manufacture paint ingredients from which the individual painter combined these ingredients into a usable medium of his or her own formulation. It was not until the latter part of the century that paint manufacturers put their first prepared paints on the market. This early manufacturing process involved grinding with oils or varnishes on simple stone mills, which became the uni- versal machines for dispersing pigments. Some of these mills remained in use until World War II, which indicates that the technology and business of making paint was extremely primitive and still in its infancy well into the twentieth century. Table 1.2 provides a more detailed summary of the development of coatings up to this point of an emerging industry. A Coating System The railroad made a significant contribution to the development of protective coatings in the early 1900s. Bridges were a vital part of the rail system and many of the major ones were constructed with riveted steel; thus, it was necessary that they be given maximum corrosion protection. One of the most effective systems consisted of a red lead-linseed oil primer applied in one or more coats, followed by a linseed oil-graphite topcoat applied in two or more coats. The ability of the linseed oil to penetrate and wet the mill scale on the surface of steel beams, plates, and structures played a significant part in the ability of the coating system to resist corrosion. This marked the first of the truly protective coatings and created the dark gray color characteristic of these steel bridges. This first system provided good long-life corrosion protection, except in extremely corrosive marine or industrial conditions. The coating was brush-applied and thoroughly wet the steel surface. The system’s heavy red lead primer offered some corrosion inhibition and the graphite topcoat increased water resistance, with its flake structure protecting the vehicle from weathering. This type of coating became a standard of the day for exposed steel structures such as the one shown in Figure 1.4. As long as the making of paint was considered more of an art than a science, progress in the industry was slow. It was not until the early 20th century that an increased emphasis on science and chemistry in particular, brought a new era in the coating industry and was recognized. Until the late 1920s, however, the number of chemists in paint factories was still limited and their sphere of activity restricted. Coating Introduction to Corrosion 3 TABLE 1.2: Historical Development of Paint 15000 BC First known painting: caves at Lascaux, France and Altamira, Spain. 8000–6000 BC Egypt: First synthetic pigment, Egyptian Blue; natural pigments used were red and yellow ochers, cinnabar, hematite, orpiment (yel- low), malachite, azurite, charcoal, lampblack, and gypsum; vehicles used were egg white, gelatins, and beeswax; developed crude brushes to apply paint. 6000 BC Asia: Calcined (fired) pigments and organic pigments. Vehicles used were egg white, beeswax, and gelatins. Chinese lacquer is pre- historic. 1500 BC Egypt: Imported indigo and madder to make blue and yellow, developed first protective coating, pitches and balsams to coat boats. 1000 BC Egypt: Developed varnish from the gum of the acacia tree (gum acacia). 1122-221 BC Chinese buildings decorated with lacquer inside and out, lacquer used on carriages, wagons, and harnesses, lacquer also used in Japan and Korea. Roman Era Same essential materials as Egypt. Also, several artificial colors (white lead, litharge, red lead, yellow oxide of lead, Verdigris, and bone black). Pitch used for tarring ships. Pitch was used on ship bottoms. 400 AD Japan: Japanese lacquer-sap from “Varnish Tree,” sap also used to waterproof drinking vessels. 600 AD First suggestion for use of vegetable oil in varnish. 1100 AD First written description for preparing an oil varnish. Dissolved molten rosin in hot oil. Middle Ages Considerable use of paint to protect wood. American Colonists Made paint using sage and skimmed milk with earth pigments. 1773 Watin first described the paint and varnish industry. Copals and amber used as resins and turpentine as thinner. One lb of resin used with ¼ to ½ lb of oil. 1790 First varnish factory established in England. 1804 First classes of varnish described by Tingrhy. 1883 J. Wilson Neil: first to give details of varnish manufacture. 1840 First reference to use of zinc as a protective coating. 1850 Zinc oxide introduced in white pigment in France; was one of first steps towards a change in the paint industry. 1867 First prepared paint on the market. 1736-1900 Watin book on varnish formulas: varnish makers standard up to 1900, reprinted 14 times. 1800-1900 Red lead-graphite linseed oil paint, first protective coating system. Sources: Encyclopedia Britannica, Macropedia, Vol. 13, Paints, “Varnishes and Allied Products,” pp. 886-889, 1974; World Book Encyclopedia, Vol. 15, “Paint,” p. 24, 1978; Mat- tiello, Joseph J., Protective and Decorative Coatings, Vol. 1, “Introduction, Rise of the Industry,” John Wiley & Sons, Inc., New York, NY, pp. 1-8, 1941; Neil, J. Wilson, Manufacturers of Varnishes, Vol. 49, Part 2, Trans. Roy. Soc. Arts, pp. 33-87, 1833; Mallet, R., British Assn. for Advancement of Science, Vol. 10, pp. 221-288 (1840). technology consisted largely of empirical data obtained through trial and error. White lead in oil remained standard for exterior house paint, although ready-mixed paint containing white lead and zinc oxide was making headway. Titanium dioxide pigments were still around the corner, and choices in pigments were ex- tremely limited, or in some cases nonexistent. The principal varnish oils used at this time were linseed and tung. Although many varnish makers resented the intrusion of so-called upstart chemists, new “wonder” varnishes were nevertheless being pro- duced. These varnishes, which dried faster and resisted turning white in water, were developed through a combination of tung oil and ester gum made by heat-reacting rosin and glycerol. The first completely synthetic resin introduced was made from phe- nol-formaldehyde,5 but even this development was considered only a minor one. It was not until at least a decade later that significant improvements were made following the availability of 100% oil-soluble phenolic resins. These vastly upgraded the var- nishes of the time by increasing flexibility, decreasing the drying time, and making them much more durable to both weather and water. World War I produced nitrocellulose, which was also a com- FIGURE 1.4: Complicated bridge structure, all of riveted construction with pletely synthetic resin. It was made by reacting cellulose with openwork vertical supports and multiple angle braces, shows clean, rust- sulfuric and nitric acids, and was produced as an explosive in free trusses. extremely large quantities during World War I. Thus, as the war 4 Introduction to Corrosion came to a close, there were large unwanted volumes of nitrocellu- lose still available. The material was a hazard to keep in inventory as well as a disposal hazard. DuPont chemists, however, soon deter- mined that if the material were put into solution it would form a clear, continuous film. This discovery was the beginning of the lac- quer industry, which had a major impact on the automotive industry. Prior to the early 1920s, all automotive finishes were of a varnish type, requiring multiple coats with long drying times. Nitrocellulose, however, dried as soon as the solvent evaporated, leaving a clear film that could be easily pigmented. In addition, nitrocellulose in solu- tion form was no longer an explosion hazard. The principal varnish oils used at this time were linseed and tung. Although many varnish makers resented the intrusion of so-called upstart chemists, new “wonder” varnishes were nevertheless being produced. These varnishes, which dried faster and resisted turning white in water, were developed through a combination of tung oil and ester gum made by heat-reacting rosin and glycerol. The first completely synthetic resin introduced was made from phenol-form- aldehyde,5 but even this development was considered only a minor one. It was not until at least a decade later that significant improve- ments were made following the availability of 100% oil-soluble phe- nolic resins. These vastly upgraded the varnishes of the time by in- creasing flexibility, decreasing the drying time, and making them much more durable to both weather and water. FIGURE 1.5: Riveted tank car coated with chlorinated rubber primer, two vinyl chloride acetate silica-filled intermediate coats, and two or more Binders clear vinyl chloride acetate finish coats. The synthetic resin developments, which came out of this field of organic chemistry, became the basis for protective coatings used from that time on. Alkyd resins, chlorinated rubber, vinyl copolymers, acrylics, and methyl-cellulose were all developed during this period. While these materials were extensively tested in coating formu- lations during this period, progress was still slow. A number of other developments were necessary before the new coating materials could be successful. One such development was that of proper solvents. The vinyl resins were soluble only in extremely strong solvents and, while acetone was available, its evaporation rate was so rapid that satisfactory films could not be formed from the acetone solution. Many of the new resins were brittle as well, and required the addition of some softening agent in order to provide a durable surface film. It was actually the development of solvents and plasticizers, occurring at the same time as that of synthetic resins, that made coatings possible. Some early vinyl coatings, as well as some made from chlorinated rubber, were developed in the 1930s. Both had good chem- ical resistance. The original vinyl copolymers, however, would only adhere to a very porous surface and were therefore not satisfactory for application to steel surfaces. When used alone, chlorinated rubber was likewise unsuitable because it was an extremely brittle, hard, and horny-textured substance. It was soon discovered, however, that its addition to oils and alkyd resins increased drying speed and resistance, and conversely plasti- cized the chlorinated rubbers. In later years, the modification with acrylic resins further increased the color and gloss retention prop- erties of chlorinated rubber coatings. It is interesting to note that the first satisfactory primer for vinyl resin was actually one developed from chlorinated rubber. Not only did it adhere to steel, but the combination of a chlorinated rubber primer and vinyl copolymer body coats and topcoats formed the first of the really chemical-resistant protective coatings. There were, however, some application problems since both materials dried rapidly. The material nevertheless provided the most resistant protective coating available at that time and was used as a chemical-resistant coating on into the early 1940s. This coating system found application in the chemical, sewer, and marine industries, and as a lining for wine tank cars (Figure 1.5) and for bait and fish storage tanks in the tuna industry. The new chlorinated rubber-vinyl copolymer combination thus represented the first major breakthrough in the development of a corrosion-resistant coating Surface Preparation A second breakthrough, which also occurred at this time, was the increased use of sandblasting. Although sandblasting was a well- known technique in the 1930s, it was considered a messy process in which very few plant managers wanted to be involved. It was soon recognized, however, that a sandblasted surface was necessary for proper performance of the new synthetic resin coatings. This discovery came when the U.S. Navy realized that in order to prolong the life of their ships at sea, it would be necessary to im- prove ship coating effectiveness. They soon found that the chip-and-scrape procedures were not adequate in achieving the kind of surface preparation essential to improved coating life. Thus, the Navy’s eventual acceptance of sandblasting as a standard method of surface preparation was the beginning of a more general use of proper surface preparation. This new practice of thoroughly cleaning a steel surface prior to coating application, in addition to the improved adhesion of vinyl coatings to steel, provided the foundation for the protective coating industry. A significant breakthrough in surface preparation began in 1975 with the commercialization of the first waterjet pump by Flow Corp. In 1975 a method of adding garnet abrasives into the waterjetting stream increased its cutting efficiency. It should be recognized that waterjetting alone does not create profile on a surface, but it does a very efficient job of cleaning existing profile. As this technology continued to develop and the power of the waterjet pumps increased to 72,000 psi or more, waterjetting created a major advance in surface protection for maintenance operations in both industrial and marine structures. Many contractors and owners shifted away Introduction to Corrosion 5 from dry abrasive blasting to either pure waterjetting (only water) or water blasting (water and abrasive combinations) in order to meet increasingly stringent governmental regulations regarding health, safety, and hazardous waste disposal. This new technology created a need for new surface preparation standards since a surface cleaned with waterjetting looks different than the same level of cleanliness developed with dry abrasive blasting. The development of pictorial standards by major coatings man- ufacturers such as Hempel and International Paint that were eventually transformed into industry-wide visual standards by SSPC and NACE, along with the development of surface-tolerant epoxy coatings by most global scale manufacturers, have made waterjetting the fastest growing surface preparation method since the year 2000. Protective Coatings This period produced two other breakthroughs, which added to the beginning revolution in corrosion control through protective coatings: the Australian development of the first inorganic zinc (IOZ) coating (stoving grade) followed by an American refinement involving the use of a post-curing solution rather than heat curing, and, at about the same time, the European development of organic zinc-rich coatings. Both events were significant in that they eventually led to the use of zinc primers for almost all high-performance protective coating systems. Their value, however, was not commonly recognized until a practical method of applying the coatings to existing structures was developed more than a decade later. While World War I marked the beginning of what is now the paint industry, World War II provided the impetus behind the devel- opment of the protective coating industry. The need to conserve labor, to lengthen the time a ship could be at sea without returning to drydock, to protect structures against corrosion that developed from rapidly expanding chemical and fertilizer industries, and to conserve existing plants by preventing their deterioration by corrosion, all pushed the development of the protective coating industry away from paint and toward the more resistant polymers and film-forming materials. Shortly after World War II, the development of another type of material, epoxy resins, had a major impact on the protective coating field. These materials reacted in place to form a protective coating that was easier to apply and had good adhesion and acceptable resistance to corrosion. The epoxies were originally amine cured. Later, however, polyamide epoxy coatings were developed, which provided increased adhesion, some flexibility, and increased water and chalk resistance as compared to earlier products. Today there are more than 75 categories of epoxy coatings with numerous different curing agents. Polyurethane coatings were also developed during this period, but the early polyurethane resins were considered inferior to epoxy because of their poorer water resistance and tendency to yellow over a period of time. Development of newer, higher solids polyure- thane resins beginning in the 1990s eliminated the yellowing tendency and increased their flexibility. Water resistance remains weaker than epoxies. Further refinements of the polyurethane technology resulted in the introduction of very high-build, direct-to-metal products classified as polyaspartics. Polyisocyanate resins first became available in 1952. However, the polyisocyanate build coats and finish coats did not make a major impact on the coatings industry until the marketing of an epoxy modified polysiloxane coating with excellent film strength, color, and gloss retention capabilities in 1994. More recently, polyurethane-modified polysiloxane coatings have been introduced with the same level of color and gloss resistance but increased flexibility compared with the epoxy polyisiloxanes. A major breakthrough in the IOZs field came with the development of self-curing IOZ coatings. These materials eliminated the difficulty of applying the curing agent to the post-cured products, and their rapid cure to insolubility eliminated much of the difficulty with weather during the application stage. While the first self-curing IOZ coatings were water-based, the later development of solvent borne IOZ coatings based on ethyl silicate had a major impact on the protective coating field. Most of the coating products developed during the World War II period remain in present use, with the exception of those that do not meet current standards for volatile organic compounds (VOC). VOC emissions control was an outgrowth of the Clean Air Act of 1978 and has led to major changes in volume solids of organic coatings. While improvements and developments in both organic and inorganic coatings continue to be made, there has been no breakthrough in coating technology since World War II that has had the impact of the IOZ and epoxy coatings, with the possible exception of aliphatic polyurethane topcoats. Significant improvements in epoxy technology has extended their use into both aggressive immersion situations and onto marginally prepared rusty surfaces. Dr. Otto Bayer first created polyurethane polymers in 1937. Polyurethane foams were created in 1941. Coatings development since 1955 has made a significant impact on the use of protective coatings. Whereas the epoxy coatings had excellent durability and chemical resistance (dependent upon the formulation), all of them suffered from the poor resistance to ultraviolet attack on the epoxy resin. This created unacceptable aesthetics in the form of chalking and loss of gloss and color. The first attempts to rectify this shortcoming involved the use of vinyl/acrylic topcoats over the intermediate coat of epoxy. While this improved the retention of color and gloss, the period of retention was still not acceptable. With the advent of two-component aliphatic polyurethane topcoats in the late 1960s, a major improvement in color and gloss retention became available along with a corollary increase in chemical and solvent resistance of the topcoat compared to vinyl/acrylics. The result has been that current versions of aliphatic polyurethanes are the topcoats of choice in protective coating systems since the 1990s. Another significant development in polyurethane technology that also occurred in the 1970s and 1980s was single-component, mois- ture-cured polyurethane systems. First developed in Europe by Bayer AG, these began to be tested on bridges in the United States in 1973. A trio of steel truss type bridges in Pittsburgh, Pennsylvania were painted beginning in 1978 and showed less than 5% corrosion in 1997. Aimed primarily at the maintenance market, systems commonly consist of an aromatic polyurethane primer, either an aromatic or an aliphatic polyurethane intermediate coat, and aliphatic polyurethane finish coat. Primers usually contain IOZ, aluminum, or inhibitive pigments such as various oxides and phosphates. Intermediate coats usually contain some type of barrier pigment such as micaceous iron oxide. Immersion resistant systems were originally based on coal tar pitch modifications Because of the concerns about the potential carcinogenic properties of coal tar pitch, more recent immersion-resistant epoxies are based on hydrocarbon resins. The major advantage of moisture cure polyurethane technology has been its ability to be applied over marginally prepared surfaces at relatively high humidity levels. Two major technology improvements occurred in the 1990s: novolac-modified epoxies for tank linings and siloxane modifications for both linings and chemical/atmospheric environments. The novolac epoxies have had the greatest impact and are now available in 6 Introduction to Corrosion TABLE 1.3: Significant Developments in Paint and Coatings Since 1900 1909 First completely synthetic resin introduced by Dr. Leo H. Bakeland. Made from phenol-formaldehyde. Was insoluble in oil and not useful until modified in 1920–24. Discovery was the beginning of the Bakelite Corp that became Union Carbide and is now Dow Chemical. 1918 First sale of titanium pigment. 1920–1925 Cellulose nitrate and acetate developed for the paint field. Alkyd resins developed. 1928 100% oil soluble phenolic resin developed. 1929 Urea formaldehyde resin developed. 1930 Chlorinated rubber developed. 1933 Vinyl copolymers developed from vinyl chloride, vinyl acetate, vinyl alcohol, and acrylic. 1935 Ethyl cellulose and cellulose acetobutyrate developed. 1938 First vinyl protective coating developed and used without baking. Five-coat systems: chlorinated rubber primer, two vinyl body coats, and two vinyl seal coats. 1939 First IOZ coating developed in Australia. 1940 Organic zinc-rich coatings started in England. 1941 Polyurethane foams created by Bayer in Germany. 1942–1945 Sandblasting specified as surface preparation on Naval ships. Vinyl wash primer developed. Vinyl tripolymer resin developed which provided good adhesion to steel. Silicone resins developed. 1945 First self-priming vinyl coating developed and used. 1948 Styrenated oils and alkyds developed. 1952–1955 First practical IOZ coating developed and used in U.S.A. Epoxy resins appeared as coating raw materials, amine cured. Polyurethane coatings in U.S.A., were known. 1955–1960 Epoxy polyamide coatings developed. First water-base self-curing IOZ coatings. 1960–1965 Self-curing, ethyl silicate base, IOZ coatings used in the U.S.A. 1965–1970 Single-package IOZ coating developed. 1972 First self-polishing copolymer antifouling paint. 1970–1975 Two-component aliphatic polyurethane coatings. 1975–1980 Moisture cured polyurethane coating systems used in the U.S.A. 1881 First anti-fouling paint. 1980 Waterborne acrylic maintenance coatings. 1980-1990 100% solids epoxies and polyurethanes. 1985-1990 Waterborne epoxy coating systems. 1990-1995 Epoxy-novolac tank linings. Polysiloxane coating systems. Polyaspartic coating systems. Note: All of the above dates are approximate. Many materials shown were in the development stage for several years before entering the coating market. Sources: Clark and Hawley, The Encyclopedia of Chemistry, “Protective Coatings,” Reinhold Publishing Corp., New York, NY, pp. 785–789, 1957; Mattielo, Joseph J., Protective and Decorative Coatings, Vol. 1, “Introduction, Rise of Industry,” John Wiley & Sons, Inc., New York, NY, pp. 1–8, 1941. 100% solids versions. The siloxanes have been married to various resin backbones for specialized purposes including heat resistance and color and gloss retention. Table 1.3 provides an outline of the significant developments in paint and coatings since their emer- gence as an industry at the beginning of the 20th century. White Pigments Without the development of several other ingredients in coatings, the binders themselves would have been of little consequence. The improvement of the white pigments, for instance, which paralleled the development of binders, was a major improvement in the industry. White lead was the primary white pigment available up until the mid-1800s when zinc oxide was developed as a white pigment. The combination of white lead and zinc oxide was soon used almost exclusively for good exterior white paints. Titanium dioxide was introduced during World War I and also had a significant impact on the paint industry. As shown in Table 1.4, which lists the various white pigments in the order they were developed and used by the paint industry, titanium dioxide has 5 to 10 times the tinting strength and hiding power of the earlier white pigments. (Both pigment concentration and the type of binder influ- ence hiding power. Various grades of the same pigment type also differ in hiding power.) Significant improvements in the manufacturing process for titanium dioxide have yielded finer grades with greater resistance to degradation within the coating systems. Additionally, the advent of the pre-dispersion blends improved the manufacturing process of the finished paints and coatings at reductions in cost per gallon. Titanium products were originally combined with other inert pigments. The original titanium dioxide was the anatase form of the pigment. This material, which had a number of drawbacks, particularly caused paint to chalk very readily and heavily. The rutile form of titanium dioxide was finally introduced in the early 1940s, and from that time on, served as a major white pigment for both the paint and the new protective coating industry. It was used in the protective coatings field primarily because of its very inert chemical characteristics. Titanium dioxide is inert to most acids and alkalis and is therefore incorporated into many chemical-resistant coatings. Introduction to Corrosion 7 TABLE 1.4: Tinting Strength and Hiding Power of White Pigments Tinting Strength Hiding Power (ft2/lb) Hiding Units Basic Lead Carbonate 100 15 1.00 Basic Lead Sulfate 85 13 0.87 Zinc Oxide 200 20 1.33 Zinc Oxide (35% Leaded) 170 20 1.80 Lithopone 260 20 1.33 Titanium Barium Pigment (a) 380 40 2.67 Titanium Barium Pigment (b) 430 46 3.07 High Strength Lithopone 400 44 2.93 Titanated Lithopone 400 44 2.93 Titanated Magnesium Pignment 440 47 3.13 Zinc Sulfide 540 58 3.87 Lead Tinanate 570 60 4.00 Titanium Dioxide 1,150 115 7.00 Source: J.J. Mattielo, Protective and Decorative Coatings, Vol. I, “Introduction, Development of Industry Today” (New York, NY: John Wiley & Sons, Inc., 1941) p. 13. Solvent Development The development of synthetic resins and white pigment was also paralleled by the development of other types of pigment, solvents, and plasticizers. The large-scale use of nitrocellulose lacquers is attributed not only to the successful production of lower viscosity nitrocellulose (which was easier to use), but also to a large supply of n-butyl alcohol, which accumulated as a fermentation process byproduct in the production of acetone during World War I. This butyl alcohol was easily converted to butyl acetate, a very desirable nitrocellulose solvent. With nitrocellulose available as a film-former, butyl alcohol and butyl acetate could be produced in quantity as solvents. The nitro- cellulose lacquer industry was thus able to increase its volume from 1 to 70 million gallons in 25 years. This provided the impetus for the development of other solvents (ketones, esters, cellosolves, nitroparrafins, chlorinated hydrocarbons, and specialty solvents such as diacetone alcohol) that were eventually used in the protective coatings industry. They also allowed the use of very high molecular weight resins and combinations of resins, which otherwise would have been impossible. Distinction in Terms Up to this point, the words “paint” and “protective coatings” have been used rather loosely. Much discussion has revolved around the use of these two terms and there is little doubt that much of the terminology and many of the uses, basic materials, and even manufac- turing processes are similar for both paint and protective coatings. On the other hand, there certainly is a case to be made supporting a distinction between the two materials, despite the opinion, particularly of many conventional paint suppliers, that paint and coatings are essentially one and the same. A majority of suppliers of protective coatings agree to the distinction, however, pointing to not only vast differences in composition, but also in use. This perspective is made even clearer by a statement from the Encyclopedia of Chemistry5 that states: Films prepared from oils alone are totally inadequate for protective coatings and combinations of natural resins and derivatives of rosin do not meet the growing needs of industry for protective coatings with adequate resistance to acids, alkalies and weathering and with retention of high elasticity under stress and aging. To meet this need the chemical knowledge of polymerization has been utilized during the past 30 years to develop synthetic resins useful in the surface coating field. Other references, however, make little distinction between the words “paint” and “coating.” In fact, they are often used interchange- ably (i.e., all paints are coatings and all coatings are paints). The definition of a coating taken from the “Glossary of Terms” in the Man- ual for Coatings of Light Water Nuclear Plants, for example, is “Coatings (paints) are polymeric materials that applied in fluid stage, cure to a continuous film.6 This, of course, could serve as a general definition for either a paint or coating because it is a very broad and simple statement. When considering coatings in relation to corrosion, however, it becomes necessary to more specifically define the two terms. Definitions Paint may be defined as any liquid material containing drying oils alone or in combination with natural resins and pigments which, when applied to a suitable substrate, will combine with oxygen from the air to form a solid, continuous film over the substrate, thus providing a weather-resistant decorative surface. Paints continue to oxidize over their entire lifetime and gradually become porous to oxygen, water, and ions that may be deposited on the surface. Thus, they provide less permanent protection against corrosion than the more sophisticated protective coating. Paint may also be defined as any liquid material containing aqueous dispersions of latex, polyvinyl acetate, or acrylic or modifications thereof, in combination with pigments, plasticizers, and additives, which when applied to a suitable substrate, will coalesce into a solid continuous film over the substrate, thus providing a weather-resistant decorative surface. These paints are semipermeable, thus allow- ing moisture vapor to penetrate and release from the dried film. A protective coating is chemically a substantially different material. It outperforms paint in adhesion, toughness, and resistance 8 Introduction to Corrosion to chemicals, weather, humidity, and water. A protective coating is any material composed essentially of synthetic resins or inorganic silicate polymers which, when applied to a suitable substrate, will provide a continuous coating that will resist industrial or marine envi- ronments. A protective coating should prevent serious breakdown of the basic structure in spite of abrasion, holidays, or imperfections in the coating. In order to provide corrosion protection, the protective coating must also: (1) Resist the transfer or penetration through the coating of ions from salts that may contact the coating; (2) Resist the action of osmosis; (3) Expand and contract with the underlying surface; (4) Have and maintain a good appearance, even under extreme weather conditions. In addition, it must be able to fulfill these requirements over a period of time long enough to justify its price, surface preparation, and application costs. In the context of this definition, the protective coating may be formed solely by the evaporation of solvents leaving a resinous film (lacquer), by condensation or internal chemical reaction (epoxy), by reaction with products in the air (alkyd, polyurethane, or IOZ coating), by coalescence (emulsions, plastisols, or dispersions), by heat as a hot-melt (coal tar or asphalt), as a powder that is melted on the surface (fusion-bonded epoxy), or by applying a preformed sheet of resinous coating material over the structure (e.g., vinyl or polyethylene sheet). Naturally, there are exceptions to these definitions, including some alkyds, chlorinated rubbers, and epoxy esters, which are a combi- nation of both oils and synthetic resins. These materials combine some of the properties of both paints and coatings and are very useful for some purposes. On the other hand, since they contain drying oils, their properties are limited because of the chemical nature of oils, and their use is limited to less severe exposures than those of most protective coatings. For further clarification, the definition of a protective coating can be divided into two additional parts, according to the intended use of the material. The Encyclopedia of Chemical Technology makes the distinction as follows: A resistant coating is a film of material applied to the exterior of structural steel, tank surfaces, conveyor lines, piping, process equipment or other surfaces which is subject to weathering, condensation, fumes, dusts, splash or spray, but is not necessarily subject to immersion in any liquid or chemical. The coating must prevent corrosion or disintegration of the structure by the environment. A resistant lining is a film of material applied to the interior of pipe, tanks, containers or process equipment and is subject to direct contact and immersion in liquids, chemicals, or food products. As such, it must not only prevent disintegration of the structure by the contained product, but must also prevent contamination of the contained product. In the case of a lining, preventing product contamination may be its most important function.7 In the context of these definitions, paint would seldom, if ever, be used as a lining. On the other hand, a protective coating may well be used for decoration purposes only, and thus might qualify under the definition of paint. Purposes The range of reasons for using paint extends beyond the historically prevalent one of decoration, although appearance remains a primary consideration. A chapter in the Facilities and Plant Engineering Handbook, entitled “Painting,” thoroughly summarizes its pur- poses as follows: Protection The major consideration in painting is preservation of the structure or equipment from the environment. Typical causes of failure are rainfall, water, vapor, sunlight, temperature variations overnight and between seasons, mildew, and rot. Other less prevalent causes include salt water and vapor, chemicals and chemical fumes, air pollution, and abrasive wear by traffic. Paint acts as a shield protecting the substrate from these elements. Proper paint choice and painting practices will definitely extend the life of the painted object and markedly reduce repair costs. Appearance The most important reason for painting (in mild environments) and second most important reason outdoors is the decorative value of paint in producing pleasant and attractive surroundings. The wide choice of colors, even in metallic finishes, the gloss and texture available, as well as its ease of application, make paint the ideal method of producing or changing the appearance of all surfaces on structures including floors as well as operating equipment and even furniture. Paints can be applied to almost all surfaces and substrates and to old painted surfaces as well, thus enabling the production of any aesthetic effect desired even when structural modifications are made (e.g., installation of new walls, partitions, and doorways). Furthermore, paint will readily cover these additions or changes so that they do not stand out. Sanitation and cleanliness Painted surfaces are generally relatively smooth and nonporous so that they are easily kept clean. The coating of rough and porous surfaces seals out dirt and other foreign matter that would otherwise be difficult to remove. Furthermore, light-colored painted surfaces, by contrast, will reveal the presence of dirt, grease, and other undesirable substances and thereby indicate that better housekeeping practices are in order. Therefore, painting for sanitation and cleanliness is especially important in food processing areas and hospitals. Illumination White and pastel-colored paints, when applied to ceilings, are highly efficient in natural and artificial light. Therefore, they can be used to advan- tage to brighten rooms and work areas and also to reduce lighting costs, when used to cover darker substrates or old paint. On the other hand, darker colors can be used to reduce and soften illumination, where so desired (e.g., in private offices). Furthermore, low-gloss (flat) finishes will soften and diffuse illumination, thus reducing glare. Efficiency Improved illumination and reduction of glare both aid markedly in improving efficiency of personnel in the area. The ability to use a variety of colors, as well as combinations, also will lead to more pleasant surroundings, another aid to improvement in morale and productivity. Paint can also be used to color-code areas, equipment, piping, and valves, and traffic paints can be used to guide traffic flow, all of which improve efficiency and reduce errors. Visibility and Safety Increased illumination improves general visibility in the area, thus also preventing potential accidents. However, colored paints can be used alone or in combination to designate dangerous areas and safety equipment such as fire extinguishers. Certain combinations, such as yellow stripes or letters on a black background or orange combined with white, are visible at much greater distances than single colors. The latter are used on TV, radio, and telephone relay towers to warn aircraft, for example.8 Introduction to Corrosion 9 Asia/Pacific: 31% China: 24.5% Western Europe: 28% Other Regions: 21.2% North America: 25% North America: 19.4% Eastern Europe: 7% Other Asia/Pacific: 18.9% Latin America: 4% Western Europe: 15.9% Middle East: 4% Africa: 1% FIGURE 1.7: World paint and coatings demand by region, 2010 FIGURE 1.6: Global coating market share as of 2005. Source: Infinity (35.1 million metric tons). Source: The Freedonia Group, Inc. Research. The above summary, however, does not cover all of the reasons for using points, as it does not address the more severe problems for which protective coatings are produced. Protective coatings also act as a barrier, prevent fouling attachment, reduce or increase fric- tion, reduce abrasion, reflect or absorb heat, and, most importantly, prevent disintegration or failure of the substrate by various forms of corrosion (e.g., marine, atmospheric, chemical, or underground corrosion). Thus, the specific purpose of a protective coating is to provide a film that will separate noncompatible materials or conditions. Protective coatings, or in this case linings, may also be used to prevent contamination, as in the case of atomic power plants where coatings are used to prevent radioactive contamination of the underlying surface. On the other hand, as a lining for a tank car con- taining caustic, wine, sugar, syrup, or petroleum products, the coating provides a film separating the steel surface from the contained material. Fire-retardant coatings also apply these principles in that they act as a barrier to heat as well as to oxidation. From a corrosion standpoint, the advertising slogan, which was popular a number of years ago, “Save the surface and you save all,” aptly applies. The paint industry is a technically complex one as well, which has grown in accordance with the 20th century’s tremendously increas- ing production of objects and structures requiring paint or protective coatings. The corresponding increase in paint products has been achieved through intensive efforts of not only paint companies, but of major chemical companies and raw materials suppliers as well. The dye industry has contributed technology and information needed to upgrade and evaluate pigments. The plastics industry, which developed around organic chemical technology, has contributed their development of polymers and gigantic molecules containing hundreds of repeating basic molecules. Thermodynamics has proved a useful tool for understanding the chemical reaction of paint ingredients and the more subtle interactions involved in solution compatibility and pigment wetting. Rheology, the science of the de- formation and flow of matter, has also had a great impact on the paint industry. Hundreds of new types of coating materials have evolved as well. These include resins, solvents, diluents, plasticizers, pigments, dryers, foam control agents, adhesion promoters, and fire-retardant chemicals and pigments. These materials have helped to achieve corrosion resistance, heat stability, and new methods of application. Paint manufacture has thus progressed from the state-of-the-art to that of a science deeply rooted in the technically complex chemical industry. Coating Market Projections According to a report published by Infiniti Research,9 the global coatings market is forecast to increase at a compound annual 10 Introduction to Corrosion growth rate of 5.46% through 2015, with the industry set to hit 8.7 Total Value of Market for Smart Materials for Coatings billion gallons and $107 billion by the year 2017. Production within 3,500 the global coatings market is witnessing a major shift away from the 3,000 developed regions of Europe and the United States to the develop- 2,500 ing economies such as Asia. Sustained demand from the developed $ Millions 2,000 countries and the burgeoning demand from the developing econo- 1,500 mies are contributing to the overall expansion of the coatings market 1,000 worldwide. Key factors contributing to growth within the coatings 500 market include the recovery of global economies from the recent economic turmoil, rapid industrialization, increasing demand from 0 2011 2012 2013 2014 2015 2016 2017 2018 end-use sectors such as automotive and construction, the increasing ©NanoMarkets, LC stringency in regulations, rising competition, growth in unique for- mulations, and technological and product developments. FIGURE 1.8: According to a study, the market for smart coatings will The pie chart shown in Figure 1.6 depicts a major change in the Smart reach Coatings Market to Grow a size of U.S.$3 billion by 2018. Source: NanoMarkets, LC. global coatings market beginning about the year 2000. The market for smart coatings is forecasted to reach a size of USD 3 billion by 2018. Thursday, 24 February 2011 While the North American market remains significant, it is a relatively mature market. Overall, global coating sales were approxi- mately $87 billion in 2005. The Asian markets are predicted to grow at Thean 8 tofor10% market rate between smart coatings is forecasted2011 and to reach a size2015 of USDwhereas 3 billion by the 2018. overall Although global paint and coatings market is predicted grow at a rate of 5.46%. many A significant impetus of the most interesting forcoatings smart the Asian are onlymarket growth just beginning hasfrom to emerge been the industrial laboratories, research company NanoMarkets believes that these coatings have a huge future heavy demand for shipping and offshore drilling structures such as tension leg platforms, drill ships, and floating production storage revenue potential. With this in mind, the experts are publishing the first market analysis, titled and offloading (FPSO) operations for deep-water areas of the world. “Smart NewCoatings technologies such as foul release coatings and new regu- Markets 2011,” of the smart coatings business including an eight-year quantita- lations from the International Maritime Organization (IMO) have driven the by tive forecast marine industry application and materialgrowth. type. The Global Industry report provides forecastsAnalysts, Inc. on various market predicts that the global coatings market will reach U.S.$107 billion by sectors 2017.for 10 smart coatings, for instance, medical, naval, and military applications. The experts Global demand for paint and coatings is forecast to rise 5.4% per year to 45.6 expect million the sectors metric considered in thistons report in will 2015. Advances be generating hundredswill be driven of millions of dollarsby in a strong acceleration in world building construction, which is expectedrevenue and will therefore to rebound be well America in North worth a look and by a variety of firms Europe, Western looking for new opportuni- according ties in the materials space. At the present time, applications fall primarily into the medical and to “World Paint and Coatings,”11 a study from The Freedonia Group, Inc., a Cleveland-based industry market research firm. Figure 1.7 construction/architecture sector. shows global paint and coatings demand by region as of 2010. Healthy growth in global manufacturing activity will also fuel increased demand for coatings used in the production of motor vehi- cles and other durable goods, as well as industrial maintenance applications. Solvent-based paint and coatings will continue to see their share of the market decline in both developed and developing markets. The Asia/Pacific region will remain the leading consumer of paint and coatings through 2015, and will also see the most rapid gains. Above-average advances are also forecast for North America, rebounding strongly from the declining demand of the 2005-2010 period. While painting and coatings demand in Western Europe will see a similar recovery from recent declines, the region will be the world’s slowest-growing regional market. The market for smart coatings is forecasted to reach a size of U.S.$3 billion by 201812 (Figure 1.8). Although many of the most in- teresting smart coatings are only just beginning to emerge from industrial laboratories, research company NanoMarkets believes that these coatings have a huge future revenue potential. With this in mind, the experts published the first market analysis of the smart coatings business, titled “Smart Coatings Markets 2011,”12 including an eight-year quantitative forecast by application and material type. The report provides forecasts on various market sectors for smart coatings; for instance, medical, naval, and military applications. The experts expect the sectors considered in this report will be generating hundreds of millions of dollars in revenue and will therefore be well worth a look by a variety of firms look- ing for new opportunities in the materials space. At the present time, applications fall primarily into the medical and construction/ architecture sector. Coatings Technologies The technology of coatings is based on organic and, more recently, inorganic chemistry, which has expanded tremendously within the last century. A plant manufacturing a broad line of trade sales, maintenance, and industrial paints now requires at least 500 to 600 different raw materials. The companies concentrating in high-performance coatings such as the epoxies, vinyls, polyurethanes, inor- ganics, and so forth, also require several hundred raw materials (e.g., organic monomers, polymers, resins, pigments, dryers, extenders or non-hiding pigments, plasticizers, and solvents). A single formulation may require as many as 15 to 20 individual ingredients to create the chemical forces that are responsible for the finished product’s surface adherence. So many raw materials are needed due to the great diversity of finished products necessary to best serve the innumerable specific purposes for which coatings are applied. Most people think of paint in its relation to houses and buildings since a great deal of so-called ordinary paint is purchased only for appearance purposes. On the other hand, most objects we come in daily contact with are coated with materials that were developed for a specific purpose. Residential aluminum siding, for example, is factory coated through a coil coating process. The finish is applied and hard baked in less than a minute, and is expected to last 15 years once the siding is applied to the house. Some of the most sophisticated coating systems are applied to automobiles. In the 1920s, as many as 10 or 15 coats of lacquer were applied to automobiles. While the finely rubbed finish was beautiful when new, it lasted only a relatively short time before it started to dull, chalk, and, in many cases, change color. Today, only two or three coats of paint are applied, an outstanding gloss is obtained, color possibilities are almost innumerable, most colors will maintain their gloss and shade for a number of years, and little, if any, hand rubbing is necessary. Zinc-rich primers are applied to car frame structures by a dipping process that effectively coats all interior and exterior surfaces simultaneously. Robotic spray equipment applies colored body coats of polyurethane followed by clear coats of ultraviolet-resistant polyurethane. Coatings Economics It is easy to see the importance of protective coatings to the corrosion engineer. To put it in perspective, high-performance coatings Introduction to Corrosion 11 are used to protect structures that serve as the production facilities of the world. The value of both the old and new structures is escalat- ing at a rapid rate, making it essential to have them protected against Infrastructure corrosion with high-performance coatings, which provide a barrier 16.4% to keep these essential structures intact and free from failure. $22.6 billion In 2002, a study entitled “Corrosion Costs and Preventive Strategies in the United States,”13 was conducted from 1999 to 2001 by CC Tech- nologies Laboratories, Inc., with support from the FHWA and NACE (Figure 1.9). It revealed that corrosion costs amount to approximately Government Utilities $276 billion in the United States, which represents 3.1% of the Gross 14.6% 34.7% Domestic Product (GDP). As of February 2013, corrosion costs have $20.1 billion $47.9 billion been updated to $476 billion, 30% of which can be saved by implement- ing known corrosion mitigation technology. Studies in other countries such as the United Kingdom, Australia, Japan, etc., have placed the cost of corrosion at between 3 and 6% GDP in the individual countries. However, the true cost of corrosion cannot be reckoned in any money sum representing replacements and maintenance. We have Production & to visualize cases where some plant or machine that had been work- Manufacturing Transportation ing perfectly is suddenly brought to a standstill by corrosion break- 1.5% down.14 12.8% $29.7 billion A good example of the use of coatings to reduce corrosion cost $17.6 billion is in refined oil tankers. In the 1930s and 1940s, tankers were built with an expected life of approximately 15 to 20 years. The first part of this life (approximately seven years) was in refined oil service. By FIGURE 1.9: Breakdown of direct corrosion costs by industry. the end of this service, the bulkheads in the tanker were corroded When extrapolated to the total U.S. economy, the annual costs of corrosion to one-half of the original steel’s thickness. Any additional corro- totaled $276 billion. Source: FHWA, 2002. sion would have reduced the bulkhead below the point of minimum safety. Thus, the ships were usually transferred for the remainder of their existence to black oil service, which for the most part is much less corrosive than the refined products. Today, however, the life of the tanker is considered 28 to 30 years with no allowance for interior corrosion. This has been accom- plished through the use of high-performance IOZ coatings that have proven that with proper application they can maintain bulkheads of a refined oil tanker without loss of metal for 20 years. The entire 30-year life of the refined oil service vessel is thus used in hauling the refined products for which it was designed. The coating, in this case, not only allows the continued transportation of the higher-value cargo, but it also reduces the tank’s interior maintenance costs to a minimal amount. In an article in the Marine Engineering Log of August 1974,15 an example is cited of three 71,000-ton vessels that were treated in 1964 with interior and exterior coatings. As of 1974, the coating was almost perfect, 95% to 97% intact; the value of the internal coating has been proved years ago—when coating eliminated the extensive steel renewals previously required. Today, the bulkhead replacement costs mentioned in the article would amount to approximately $200 to $250 per ft2, compared to $8 to $10 per ft2 for a protective coating with a 20-year life span. In the case of chemical tankers, the increase in purity of chemical products has made them more aggressive, thus requiring ever more sophisticated coatings and linings. The life of a chemical tank lining rarely exceeds 10 years without extensive repairs and the cost per ft2 commonly exceeds $12. Thus, in a sense, coatings serve as an insurance policy on the life of the structure. The coating cost amounts to a very small percentage of any structure’s total cost, and this small incremental cost protects the structure against disintegration for many years. Coating Manufacture Coating manufacture started when the first prepared paint was put on the market in 1867. This first product was primarily a mixture of white lead and linseed oil ground together on a burr stone mill. These mills usually consisted of two slabs of granite or similar hard natural stone cut so that the two flat surfaces rubbed together, with the grooves carrying the undispersed paint material in between them. Several runs through the mill were made before the required fine texture or proper pigment dispersion was obtained. Much of the paint manufacturing process involves the dispersion of pigments. The following is a review of the manufacturing process extracted from Unit 1 of the Federation Series on Coating Technology.16 In the manufacture of pigmented products, the most critical operation is the incorporation of the pigment in the binder or vehicle, as the case may be. If the ultimate pigment particles are too coarse, it is necessary to reduce them in size by a true grinding process. Most of the pigments used today are sufficiently fine that no grinding is required. However, they contain clusters or agglomerates that must be broken down to the separate particles, which must then be wetted by the binder. This process is correctly designated as disper- sion. Some kinds of milling equipment perform both grinding and dispersion, while other types disperse only. In the former category were the stone mills, which were the most common milling equipment 60 years ago. A few plants bore with them until about 30 years ago. They were doomed to the scrap pile by slow production, high maintenance, variable results, and need for close attention. Three roll steel mills appeared about 60 years ago. They operate with a small clearance between rolls and a speed differential be- tween successive rolls. If used for grinding, they are inefficient and the rolls are etched. Their only proper use is for dispersion. Since dispersion depends on the shearing action produced by the differential in roll speed, a high viscosity vehicle is essential to good results. Although largely displaced by newer, more efficient equipment, roller mills with three to five rolls continue in limited use. Next 12 Introduction to Corrosion Figure 1.10: Modern paint manufacturing plant showing large scale-sup- Figure 1.11: Manufacturing and packaging area of a large paint plant showing ported mixing vessels and pipelines for raw materials and semi-processed overhead storage of liquid materials, pipelines, automatic canning equipment, liquids. Photo courtesy of Ameron, Inc., EFD Facilities, Wichita, KS. and end product conveyors. Photo courtesy of Ameron, Inc., EFD Facilities, Wichita, KS. came pebble mills and, a little later, steel ball mills, the latter for dark colors only. Collision between the pebbles or balls and with the shell provides sufficient impact to reduce particle size as well as dispersion. Frequently, they have been used when dispersion only is required. Pebble and ball mills require little attention and are more economical than earlier mills. In general, they do not develop as high a gloss as roller mills. Although pebble and steel ball mills