Nuclear Imaging-The Scintillation Camera PDF
Document Details
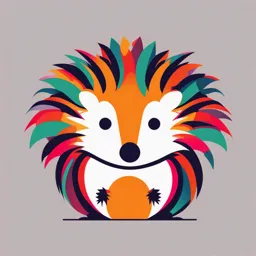
Uploaded by VersatileLongBeach
Elias Estephan, Ph.D.
Tags
Related
- General Overview of Radiology (TG05-CG13) (V2) PDF
- Tomographic Nuclear Imaging Lecture Notes PDF
- Invasive and Noninvasive Cardiology Diagnostic Methods PDF
- I-131 Metastatic Survey PDF
- RCSI MAP.18 Gamma Camera & Positron Emission Tomography (PET) PDF
- Previous Exam Questions in X-ray, Nuclear Imaging & MRI PDF
Summary
This document provides detailed information about nuclear imaging and scintillation cameras. It covers topics such as design, operation, performance, and quality control. The document is suitable for a postgraduate-level audience.
Full Transcript
Nuclear Imaging-The Scintillation Camera 1. Planar Nuclear Imaging: The Anger Scintillation Camera Design and principles of operartion Performance Design factors determining performance Effects of scatter and attenuation on projection images Operation and...
Nuclear Imaging-The Scintillation Camera 1. Planar Nuclear Imaging: The Anger Scintillation Camera Design and principles of operartion Performance Design factors determining performance Effects of scatter and attenuation on projection images Operation and Routine quality control Computers, whole body scanning and SPECT Obtaining High-Quality Images 2. Computers in Nuclear Imaging 1 Nuclear Imaging-The Scintillation Camera Elias Estephan, Ph.D. Nuclear imaging produces images of the distributions of radionuclides in patients. nuclear imaging uses gamma rays, characteristic x-rays …… Image photon flux density at each point and direction the x- or gamma rays from the radionuclide at each portion of a patient are emitted isotropically Nuclear medicine instruments designed to image gamma ray and x-ray emitting radionuclides use collimators that permit photons following certain trajectories to reach the detector A heavy price is paid for using collimation-the vast majority (typically well over 99.95%) of emitted photons is wasted. 2 Nuclear Imaging-The Scintillation Camera Elias Estephan, Ph.D. nuclear imaging devices in routine clinical use utilize solid inorganic scintillators as detectors because of their superior detection efficiency. ……………………………….semiconductor detectors (cadmium zinc telluride)…………………..………………… The quality of a nuclear medicine image is determined not only by the performance of the nuclear imaging device but also by the properties of the radiopharmaceutical used accumulation , activity , half-life……….. 3 Nuclear Imaging-The Scintillation Camera Elias Estephan, Ph.D. 4 Nuclear Imaging-The Scintillation Camera Elias Estephan, Ph.D. 5 Nuclear Imaging-The Scintillation Camera Elias Estephan, Ph.D. Design and principles of operartion Detector and Electronic Circuits A scintillation camera contains a disk-shaped or rectangular thallium activated sodium iodide NaI(TI) crystal, typically 0.95 cm (3/8 inch) thick, optically coupled to a large number (typically 37 to 91) of 5.1- to 7.6-cm (2- to 3-inch) diameter photomultiplier tubes (PMTs). Between the patient and the crystal is a collimator, usually made of lead, that only allows x- or gamma rays approaching from certain directions to reach the crystal. 6 Nuclear Imaging-The Scintillation Camera Elias Estephan, Ph.D. No collimator no meaningful images The collimator septa (lead) intercept most photons approaching the camera from nonperpendicular directions Passing through the holes; many of these are absorbed in the sodium iodide crystal, causing the emission of visible and ultraviolet light PMTs: light photonselectrical signals further amplified by the preamplifiers the amplitude pulse produced by each PMT is proportional to the amount of light it received from an x- or gamma ray interaction in the crystal. 7 Nuclear Imaging-The Scintillation Camera Elias Estephan, Ph.D. 2D projection of the 3D activity distribution in the patient. The PMTs closest to each photon interaction in the crystal receive more light than those that are more distant, causing them to produce larger voltage pulses The relative amplitudes of the pulses from the PMTs following each interaction contain sufficient information to determine the location of the interaction in the plane of the crystal. 8 Nuclear Imaging-The Scintillation Camera Elias Estephan, Ph.D. Until the late 1970s, only analog circuitry. The pulses from the preamps were sent to two circuits. The position determining the centroid of these pulses X-position pulse and a Y-position pulseposition of the interaction in the plane of the crystal. summing energy (Z) pulse proportional in amplitude to the total energy deposited in the crystal. Z pulse single-channel analyzer (SCA) An interaction in the camera's crystal was recorded in the image only if a logic pulse was produced by the SCA. two to four SCAs for imaging radionuclides which emit useful photons of more than one energy. 9 Nuclear Imaging-The Scintillation Camera Elias Estephan, Ph.D. In the late 1970s, hybrid analog-digital scintillation cameras X, Y, and Z pulses are converted to digital signals by analog-to-digital converters (ADCs). sent to digital correction circuitsfor improving Spatial Linearity and Uniformity. the corrected digital X, Y, and Z signals are converted back to analog voltage pulses Energy discrimination is performed in the analog domain by SCAs.digital computer 10 Nuclear Imaging-The Scintillation Camera Elias Estephan, Ph.D. Today X, Y, and Z signals are corrected, using digital correction circuits, and energy discrimination is applied, also in the digital domain. 11 Nuclear Imaging-The Scintillation Camera Elias Estephan, Ph.D. Collimators commonly used collimator is the parallel-hole collimator, which contains thousands of parallel holes (round, square, or triangular but most are hexagonal made from lead foil) The septa must be thick enough to absorb most of the photons incident upon them. higher-energy photons use collimator with thicker septa. by reducing the size of the holes or lengthening the collimator spatial resolution↗ efficiency↘ compromise between the spatial resolution and efficiency (sensitivity) selection of parallel-hole collimators: "low-energy, high-sensitivity,“ "low-energy, all-purpose" (LEAP), "low-energy, high-resolution“…………….. The size of the image (parallelhole collimator) is not affected by the distance of the object from the collimator. collimator-to-object distance↗spatial resolution ↘ 12 Nuclear Imaging-The Scintillation Camera Elias Estephan, Ph.D. A pinhole collimator : produce magnified views of small objects whose orientation is reversed a small (typically 3- to 5-mm diameter) hole in a piece of lead or tungsten mounted at the apex of a leaded cone. The magnification of the pinhole collimator decreases as an object is moved away from the pinhole Away ↗ (d pinhole and camera = d object pinhole) the object is not magnified Away ↗↗ it is minified 13 Nuclear Imaging-The Scintillation Camera Elias Estephan, Ph.D. A converging collimator: focal point in front of the camera. the converging collimator magnifies the image : magnification↗as the object is moved away from the collimator. A diverging collimator : focal point behind the camera It produces a minified image : minification ↗ as the object is moved away from the camera If a diverging collimator is reversed on a camera, it becomes a converging collimator. diverging collimator and converging collimator are seldom used today A hybrid of the parallel-hole and converging collimator, called a fan-beam collimator, is used in single photon emission computed tomography (SPECT) 14 Nuclear Imaging-The Scintillation Camera Elias Estephan, Ph.D. Principles of Image Formation the photons from each point in the patient are emitted isotropically. Some photons escape the patient without interaction, some scatter within the patient before escaping, and some are absorbed within the patient. Many of the photon are emitted away from the detector. The collimator absorbs the vast majority of those photons that reach it. over 99.9% of the photons emitted during imaging are wasted. Of those reaching the crystal, some are absorbed in the crystal, some scatter from the crystal, and some pass through the crystal without interaction. 15 Nuclear Imaging-The Scintillation Camera Elias Estephan, Ph.D. these events depend on the energies of the photons and the thickness of the crystal. Of those photons absorbed in the crystal some are absorbed by a single photoelectric absorption, whereas others undergo one or more Compton scatters before a photoelectric absorption. Ways that x- and gamma rays interact with a scintillation camera. All of these, other than the ones depicted in the upper left, cause a loss of contrast and spatial resolution. However, interactions by photons that have scattered though large angles and many coincident interactions are rejected by pulse height discrimination circuits. 16 Nuclear Imaging-The Scintillation Camera Elias Estephan, Ph.D. In emission imaging, primary photons cannot be distinguished from scattered photons by direction it can be differentiated by energy, because scattering reduces photon energy reduce the fraction of counts in the image caused by scattered radiation. nuclear imaging devices must use pulse mode (the signal from each interaction is processed separately) for event localization and so that interaction-by-interaction energy discrimination can be employed 17 Nuclear Imaging-The Scintillation Camera Elias Estephan, Ph.D. Performance Measures of Performance performance of a camera with the collimatorsystem or extrinsic measurements. camera performance without the collimator intrinsic measurements. Uniformity is a measure of a camera's response to uniform irradiation of the detector surface. The ideal response is a perfectly uniform image. Intrinsic uniformity is measured by placing a point radionuclide source placed at least four times the largest dimension of the crystal away to ensure uniform irradiation of the camera surface and at least five times away System uniformity, which reveals collimator as well as camera defects, is assessed by placing a uniform planar radionuclide source in front of the collimated camera. A planar source should be large enough to cover the crystal of the camera. The uniformity of the resultant images may be analyzed by a computer or evaluated visually. 18 Nuclear Imaging-The Scintillation Camera Elias Estephan, Ph.D. Spatial resolution accurately portray spatial variations in activity concentration and to distinguish as separate radioactive objects in close proximity. The system spatial resolution collimator resolution and intrinsic resolution (camera) The collimator resolution FWHM of the radiation transmitted through the collimator It is not directly measured, but calculated from the system and intrinsic resolutions. The intrinsic resolution is determined quantitatively sheet of lead containing thin slits placed using a point source (positioned at least five times the largest dimension of the camera) the line spread function (LSF) is a cross- sectional profile of the image of a line source. RC FWHM spatial resolution ↗ when FWHM or R ↘ 19 Nuclear Imaging-The Scintillation Camera Elias Estephan, Ph.D. The system (Rs), collimator (Re), and intrinsic (Ri,) resolutions, are related by the following equation : It can be corrected for magnification the collimator and system resolutions degrade (FWHM of the LSF, corrected for collimator magnification, increases) with increasing distance between the line source and collimator. 20 Nuclear Imaging-The Scintillation Camera Elias Estephan, Ph.D. In routine practice, the intrinsic resolution is semiquantitatively evaluated : parallel line or four-quadrant bar phantom in contact with the camera face planar radionuclide source if (collimated )or a distant point source if the collimator is removed visually noting the smallest bars that are resolved 21 Nuclear Imaging-The Scintillation Camera Elias Estephan, Ph.D. Spatial linearity : ability to portray the shapes of objects accurately. It is determined from the images of a bar phantom, line source, or other phantom by assessing the straightness of the lines in the image. Multienergy spatial registration : ability to accurately position photons of different energies when imaged through different photopeak energy windows Ga-67 (93-, 185-, and 300-keV) gamma rays, is imaged the resultant image will be a superposition of images of different magnifications 22 Nuclear Imaging-The Scintillation Camera Elias Estephan, Ph.D. The system efficiency (sensitivity) of a scintillation camera is the fraction of x- or gamma rays emitted by a source that produces counts in the image. intrinsic efficiency collimator efficiency the fraction (f) of interacting photons accepted by the energy discrimination circuits: 23 Nuclear Imaging-The Scintillation Camera Elias Estephan, Ph.D. The collimator efficiency is the fraction of photons emitted by a source that penetrate the collimator holes The intrinsic efficiency (crystal) the fraction of photons penetrating the collimator that interact with the NaI(Tl) , is determined by the thickness of the crystal and the energy of the photons the thickness linear attenuation photo peak efficiency, defined as the fraction of photons reaching the crystal that produce counts in the photopeak of the energy spectrum: the system efficiency and each of its components range from zero to one. low-energy all-purpose (LEAP) parallel-hole collimators have efficiencies of about 2*10-4 and low energy high-resolution parallel-hole collimators have efficiencies of about 1*10-4. 24 Nuclear Imaging-The Scintillation Camera Elias Estephan, Ph.D. The energy resolution : ability to distinguish between interactions depositing different energies in its crystal. The energy resolution is measured by exposing the camera to a point source of a radionuclide, usually Tc-99m The energy resolution is calculated from the full-width-at-half-maximum (FWHM) of the photopeak. The FWHM is divided by the energy of the photon (140 keY for Tc-99m) and is expressed as a percentage. A wider FWHM implies poorer energy resolution. 25 Nuclear Imaging-The Scintillation Camera Elias Estephan, Ph.D. Scintillation cameras are operated in pulse mode and therefore suffer from dead-time count losses at high interaction rates. They behave as paralyzable systems The count-rate performance of a camera Some scintillation cameras with digital electronics can correctly process the PMT signals when two or more interactions occur simultaneously in the crystal, provided that the interactions are separated by sufficient distance. This significantly increases the number of interactions that are correctly registered at high interaction rates. 26 Nuclear Imaging-The Scintillation Camera Elias Estephan, Ph.D. Design Factors Determining Performance Intrinsic Spatial Resolution and Intrinsic Efficiency The intrinsic spatial resolution the random error associated with the collection of visible light photons and subsequent production of electrical signals by the PMTs. Approximately one visible light photon is emitted in the NaI crystal for every 25 eV deposited by an x- or gamma ray. 140-keV gamma 140,000/25 = 5,600 visible light photons 3,700 photons, reach the photocathodes of the PMTs 750 electrons divided among the PMTs, with the most being produced in the PMTs closest to the interaction. Because the processes are random, the pulses from the PMTs after an interaction contain significant random errors that, in turn, cause errors in the X and Y signals produced by the position circuit of the camera and the energy (Z) signal. These random errors limit both the intrinsic spatial resolution and the energy resolution of the camera 27 Nuclear Imaging-The Scintillation Camera Elias Estephan, Ph.D. energy photons↗ relative random errors↘ intrinsic spatial resolution↗ detecting the visible light photons ( QDE of PMT) is the most significant factor limiting the intrinsic spatial resolution (25% of the visible light contribute to the signal from the PMT). The size of the PMTs also affects the spatial resolution : PMT’s diameter ↘ the spatial resolution↗ 28 Nuclear Imaging-The Scintillation Camera Elias Estephan, Ph.D.. A thinner Nal crystal intrinsic spatial resolution ↗ less spreading of light scatters (compton ) ↘ offset from the site of the initial interaction↘ scatters preceding the photoelectric absorption increases with the energy of the x- or gamma ray. The intrinsic efficiency of a scintillation camera is determined by the thickness of the crystal and the energy of the incident x- or gamma rays. compromise between the intrinsic efficiency of the camera, which increases with crystal thickness, and intrinsic spatial resolution, which degrades with crystal thickness 29 Nuclear Imaging-The Scintillation Camera Elias Estephan, Ph.D. Collimator Resolution and Collimator Efficiency if diameters of the holes ↘and the lengths of the holes ↗ The spatial resolution ↗ (narrower FWHM of the LSF) collimator's efficiency↘. Limitation on scintillation camera performance because of the compromise between collimator efficiency and collimator resolution collimator-to-object distance ↗ The spatial resolution of a parallel-hole collimator ↘ (FWHM of the LSF ↗) linearly 30 Nuclear Imaging-The Scintillation Camera Elias Estephan, Ph.D. the efficiency of a parallel-hole collimator is nearly constant over the collimator-to-object distances used for clinical imaging. the number of photons passing through a particular collimator hole decreases as the square of the distance the number of holes through which photons can pass increases as the square of the distance. The width of the LSF increases with distance. Nevertheless, the area under the LSF (total number of counts) does not significantly decrease with distance 31 Nuclear Imaging-The Scintillation Camera Elias Estephan, Ph.D. 32 Nuclear Imaging-The Scintillation Camera Elias Estephan, Ph.D. System Spatial Resolution and Efficiency The system spatial resolution (corrected for magnification) ↘(FWHM ↗) as the collimator- to-object distance ↗ for all types of collimators The system efficiency with a parallel-hole collimator is nearly constant with distance. The ES with a pinhole collimator decreases significantly with distance. The ES of a fan-beam collimator increases with collimator-to-object distance 33 Nuclear Imaging-The Scintillation Camera Elias Estephan, Ph.D. Spatial Linearity and Uniformity Spatial nonlinearity is mainly due to interactions being shifted in the resultant image toward the center of the nearest PMT by the position circuit of the camera. tables of correction factors to correct each pair of X- and Y-position signals for spatial nonlinearities 34 Nuclear Imaging-The Scintillation Camera Elias Estephan, Ph.D. There are three major causes of non uniformity. 1. The first is spatial nonlinearities the systematic mispositioning of events imposes local variations in the count density. The linearity correction circuitry previously described effectively corrects this source of non uniformity. wavy lines represent straight lines in the object. locations shifted toward the center of the nearest PMT, causing an enhanced count density toward the center of the PMT 35 Nuclear Imaging-The Scintillation Camera Elias Estephan, Ph.D. 2. The second is that the position of the interaction in the crystal affects the magnitude of the energy (Z) signal. -caused by -local variations in the crystal in the light generation and light transmission to PMTs -variations in the light detection and gains of the PMTs. If not corrected, the fraction of interactions rejected by the energy discrimination circuits will vary with position in the crystal. These positional variations in the magnitude of the energy signal are corrected by digital electronic circuitry 36 Nuclear Imaging-The Scintillation Camera Elias Estephan, Ph.D. 3. The third is local variations in the efficiency of the camera in absorbing x- or gamma rays, such as manufacturing defects or damage to the collimator. Corretion : 1. acquiring an image of an extremely uniform planar source 2. A correction factor is determined. 3. Each pixel in a clinical image is multiplied by its correction factor The National Electrical Manufacturers Association (NEMA) publishes a document entitled Performance Measurements of Scintillation Cameras that specifies standard methods for measuring the camera performance. All manufacturers of scintillation cameras publish NEMA performance measurements of their cameras 37 Nuclear Imaging-The Scintillation Camera Elias Estephan, Ph.D. Effects of scatter and attenuation on projection images 2D projection of the 3D activity distribution in the patient. number of counts in each point in the image would be proportional to the average activity concentration along a straight line through the corresponding anatomy of the patient. There are three main reasons why nuclear medicine images are not ideal projection images - attenuation of photons in the patient - inclusion of Compton scattered photons in the image - the degradation of spatial resolution with distance from the collimator. photons from structures deeper in the patient are much more heavily attenuated than photons from structures closer to the camera face. Attenuation is more severe for lower energy photons than for higher energy photons 38 Nuclear Imaging-The Scintillation Camera Elias Estephan, Ph.D. Operation and Routine quality control Peaking : adjust energy discrimination windows to center them on the photopeak or photopeaks of the desired radionuclide. On this display, the energy window limits are shown as vertical lines. A narrower energy window provides better scatter rejection, but also reduces the number of unscattered photons recorded in the image. Peaking may be performed manually or automatically A scintillation camera should be peaked before first use each day and before imaging a different radionuclide. A small source should be used to peak the camera 39 Nuclear Imaging-The Scintillation Camera Elias Estephan, Ph.D. The uniformity of the camera should be assessed daily and after each repair. intrinsically by using a Tc-99m point source or Co-57 planar source (system) Uniformity images must contain sufficient counts , About 2 million counts for 30-cm (12- inch) diameter , about 3.5 million for 41 cm, and as many as 6 million counts for the largest rectangular head cameras. The uniformity test will reveal most malfunctions of a scintillation camera A- Uniformity images with digital spatial linearity and Z pulse correction circuitry B-The same camera, with an inoperative 40 Nuclear Imaging-The Scintillation Camera photomultiplier tube Elias Estephan, Ph.D. Multihole collimators are easily damaged by careless handling, technologists should inspect the collimators on each camera daily The spatial resolution and spatial linearity should be assessed at least weekly. four-quadrant bar phantom parallel-line phantom The efficiency of each camera head should be measured periodically. determining the activity of a source, recording the number of counts and counting time, and calculating the count rate per unit activity. Each camera should also have a complete evaluation at least annually, which includes not only the items listed above, but also multienergy spatial registration and count-rate performance 41 Nuclear Imaging-The Scintillation Camera Elias Estephan, Ph.D. Computers whole body scanning , and SPECT connected to digital computers. The computer is used for the acquisition, processing, and display of digital images and for control of the movement of the camera heads. Many large-FOV scintillation cameras can perform whole-body scanning. Some systems move the camera past a stationary patient, whereas others move the patient table past a stationary scintillation camera. Systems with two passes over each side of the patient. Newer large-area rectangular- head cameras can scan each side with a single pass Many modern computer-equipped scintillation cameras have heads that can rotate automatically around the patient and acquire images from different views--------- SPECT 42 Nuclear Imaging-The Scintillation Camera Elias Estephan, Ph.D. Obtaining High-Quality Images optimized for each type of study. Sufficient counts must be acquired so that quantum mottle in the image does not mask lesions. Imaging times must be as long as possible consistent with patient throughput and lack of patient motion The use of a higher resolution collimator may improve spatial resolution and the use of a narrower energy window scatter↘ may ↗ image contrast the camera heads should be as close to the patient as possible if not spatial resolution ↘ Collimators are easily damaged by collisions with imaging tables Patient motion and metal objects worn by patients or in the patients' clothing are common sources of artifacts 43 Nuclear Imaging-The Scintillation Camera Elias Estephan, Ph.D. 2. Computers in nuclear imaging Some manufacturers incorporate a computer for image acquisition and camera control in the camera itself and provide a separate computer for image processing and display, whereas others provide a single computer for both purposes. Digital Image Formats in Nuclear Medicine In nuclear medicine, each pixel represents the number of counts detected from activity in a specific location within the patient. Common image formats in nuclear medicine are 642 and 1282 pixels. Whole-body images are stored in larger formats If one byte (8 bits) is used for each pixel, the image is said to be in byte mode; if two bytes are used for each pixel, the image is said to be in word mode A single pixel can store as many as 255 counts in byte mode and 65,535 counts in word mode. 44 Nuclear Imaging-The Scintillation Camera Elias Estephan, Ph.D. Image Acquisition Frame Mode Acquisition Image data in nuclear medicine are acquired in either frame or list mode. Frame mode : All pixels within this image are set to zero. After acquisition begins, pairs of X- and Y-position signals are received from the camera. 45 Nuclear Imaging-The Scintillation Camera Elias Estephan, Ph.D. There are three types of frame-mode acquisition: static, dynamic, and gated. In a static acquisition, a single image is acquired for either a preset time interval or until the total number of counts in the image reaches a preset number. In a dynamic acquisition, a series of images is acquired one after another, for a preset time per image. Dynamic image acquisition is used to study dynamic processes 46 Nuclear Imaging-The Scintillation Camera Elias Estephan, Ph.D. too rapidly : too few counts to be statistically valid. if the dynamic process is repetitive, gated image acquisition may permit the acquisition of an image sequence that accurately depicts the dynamic process. Gated acquisition cardiac mechanical performance A- First, space for the desired number of images (usually 16 to 24) is reserved in the computer's memory. B-Next, time per cardiac cycle is determined. The time per cycle is divided by the number of images in the sequence to obtain the time T per image. C- Then the acquisition begins This process is continued until a preset time interval, typically 10 minutes, has elapsed, enabling sufficient counts to be collected for the image sequence to form a statistically valid depiction of an average cardiac cycle. 47 Nuclear Imaging-The Scintillation Camera Elias Estephan, Ph.D. list-mode acquisition the pairs of X- and Y-position values are stored in a list the list-mode data are reformatted into conventional images for display. The disadvantages of list-mode acquisition are that it generates large amounts of data, requiring more memory to acquire and disk space to store than frame-mode images, and that the data must be subsequently processed into standard images for viewing. 48 Nuclear Imaging-The Scintillation Camera Elias Estephan, Ph.D. Regions of Interest and Time-Activity Curves A region of interest (ROI) is a closed boundary that is superimposed on the image : drawn manually or automatically The sum of the counts in all pixels in the ROI is an indication of the activity in the corresponding portion of the patient. To create a time-activity curve (TAC), (dynamic or gated sequence) ROI must first be drawn on one image. ROI is then superimposed on each image the total number of counts within the ROI is determined for each image. the counts within the ROI are plotted as a function of image number. activity in the corresponding portion of the patient as a function of time. ROIs over both kidneys of a patient and time-activity curves 49 Nuclear Imaging-The Scintillation Camera Elias Estephan, Ph.D.