Radiation Biology Past Paper Notes 2024 PDF
Document Details
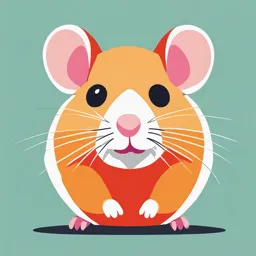
Uploaded by SalutaryHeliotrope9568
2024
Tags
Summary
These notes cover the effects of x-rays on humans, the process of ionization, and cellular responses to radiation. They explore atomic composition, molecular composition, and the roles of water, proteins, lipids, and carbohydrates in the body.
Full Transcript
**Notes for test 1 Radiation Biology** **Page 409** The effects of x rays on humans are the result of interactions at the atomic level Take the form of ionization or excitation of orbital electrons and the result of the deposition of energy in tissue When an atom is ionized, its chemical binding...
**Notes for test 1 Radiation Biology** **Page 409** The effects of x rays on humans are the result of interactions at the atomic level Take the form of ionization or excitation of orbital electrons and the result of the deposition of energy in tissue When an atom is ionized, its chemical binding properties change If the atom is part of a large molecule, the ionization may result in breakage of the molecule or relocation of the atom within the molecule The abnormal molecule may in time function improperly or cease to function, which can result in impairment or death of a cell The process is reversible Ionized atoms can become neutral again by attracting a free electron Molecules can be repaired by enzymes Cells and tissues can regenerate Early effects of radiation ========================== If the radiation response occurs within minutes or days after the exposure to radiation Late effects of radiation ========================= If the human injury is not observed for many months or years Radiobiology ============ The study of the effects of ionizing radiation on biological tissue The goal of radiobiology is the accurate description of the effects of radiation on humans so it can be used more safely in diagnosis and more effectively in therapy **Composition of the body** =========================== Radiation interacts with the body at the atomic level ===================================================== 410 The cell theory =================== The interaction at the atomic level results in molecular change which can produce a cell deficient in its normal growth and metabolism All plants and animal contain cells as their basic functional units **Atomic composition** ====================== Over 85% of the body is hydrogen and oxygen H=60%, O=25.7%, C=10.7, N=2.4% **Molecular composition** ========================= There are **5 principal types** of molecules in the body 1. water 2. protein 3. lipids 4. carbohydrates 5. nucleic acids **4 of these are macromolecules** 1. protein 2. lipids 3. carbohydrates 4. nucleic acids **Organic molecules** -- life supporting and containing carbon(C) 1. proteins 2. lipids 3. carbohydrates Nucleic acid ============ Rarest and most radiosensitive -- DNA, in the nucleus of a cell Water ===== About 80% of the human body H~2~O is the most abundant and simplest molecule in the body Helps deliver energy to target molecules thereby contributing to radiation effects One water molecule consists of 2 hydrogen and 1 oxygen atom (H~2~O) Exists in both a free and bound state, that is it can be dissociated or bound to other molecules When water is lost through perspiration it must be replaced to maintain **homeostasis** (the constancy of the internal environment of the human body) H~2~O and CO~2~ are the end products of **catabolism** (breaking down into smaller units) of macromolecules **Anabolism** -- production of large molecules from small ones **Metabolism** -- catabolism and anabolism combined **Proteins** About 15% of the human body Macromolecules that consist of amino acids held together by peptide bonds 22 AA's used in protein production or protein synthesis -- metabolic production of protein Provide structure and support for the body Proteins function as enzymes, hormones and antibodies Enzymes -- allow a biochemical reaction to continue Hormones -- exercise regulatory control over some body functions, such as growth and development Antibodies -- primary defense mechanism of the body to protect against infection and disease **Carbohydrates** Composed solely of C, H & O The ratio of H atoms and O atoms are 2:1, same as H~2~O, they are considered hydrated carbons Also called saccharides Function is to provide fuel for cell metabolism Glucose is the ultimate molecule that fuels the body, lipids can be transformed into glucose **Lipids** ========== Composed solely of C,H & O Composed of 2 kinds of smaller molecules -- glycerol and fatty acids One molecule of glycerol and three molecules of fatty acid Serve as thermal insulators and fuel for the body by providing energy reserves More difficult to get energy from lipids than carbohydrates **Nucleic acids** ================= Two principal ones in the body DNA and RNA **DNA** ======= In the nucleus Controls cell function and contains the hereditary information for a cell If it's a **germ cell** (sexual reproductive cell, sperm or egg), contains the hereditary information of the whole individual The most sensitive part of any human cell to ionizing radiation is the DNA molecule that forms individual chromosomes. The DNA material may be found within the nucleus of the cell. The cytoplasm is less sensitive and contains mostly water and organelles such as mitochondria, ribosomes, Golgi bodies, t-RNA (transfer-RNA), and so forth. Although these complex organelles (called macromolecules) are more radiosensitive than the surrounding water molecules, the DNA is still far more sensitive than any other cell structure. **RNA** ======= In the nucleus and cytoplasm 2 types messenger RNA (mRNA) transfer RNA (tRNA) Involved in the growth and development of a cell, controls protein synthesis 3 general factors which determine the radiosensitivity of a cell or organ 1. function of the organ 2. rate the cells mature 3. inherent sensitivity of the cell type **Cell proliferation:** 1000's of rad in vitro are required to produce a disruption of macromolecules Single ionizing events at a sensitive site of a critical target molecule is capable of disrupting cell proliferation **2 general types of cells in the body:** **Genetic cells** -- oogonium of the female and spermatogonium of the male, when they go under proliferation, or cell division, undergo **meiosis** **Somatic cells** -- when they go under proliferation, or cell division, undergo **mitosis** It is well established that sufficient quantities of ionizing radiation can cause a number of serious *somatic* and/or *genetic* effects. *Somatic effects* of radiation are those that affect the *irradiated body itself.* Somatic effects are described as being *early* or *late,* depending on the length of time between irradiation and manifestation of effects. The human reproductive organs are particularly radiosensitive. *Fertility* and *heredity* are greatly affected by the *germ cells* produced within the testes *(spermatogonia)* and ovaries *(oogonia).* Excessive radiation exposure to the gonads can cause *temporary or permanent infertility,* and/or *genetic mutations.* Infertility is somatic because it affects the exposed individual; genetic mutations affect future generations. **Response to radiation is related to cell type: 418** [Radiosensitivity] [Cell type] [Tissue] High Lymphocytes lymphoid tissue Spermatagonia gonads Erythroblasts bone marrow Intestinal crypt cells epithelial Intermediate Endothelial cells skin Osteoblasts growing bone Spermatids male germ cell Fibroblasts cornea, growing bone, GI tract Liver cells Low Muscle cells muscle Nerve cells brain Chondrocytes spinal cord Excessive radiation to the *reproductive organs* can cause genetic mutations or sterility. Excessive radiation to the *blood-forming organs* can cause leukemia or life span shortening. *Lymphocyte* cells are the most radiosensitive cells in the body. Neuroblasts and spermatogonia are stem cells, or immature cells, and therefore considered to be very sensitive to radiation. The lymphocyte is arguably *the most radiosensitive cell* in the human body, because a dose as low as 0.1 Gy (10 rad) can cause a decrease in the lymphocyte count. Erythrocytes are end cells, or mature cells, and have no nucleus, making them very resistant to radiation. Erythrocytes, like mature nerve cells, are practically immune to any damage by irradiation, and they also have a very long life span (about 4 months), as compared with several days for most other cells. suffix-blast = immature, stem cell; cyte = mature, nondividing The most radiosensitive portion of the GI tract is the small bowel. Projecting from the lining of the small bowel are villi, from the crypts of Lieberkuhn, which are responsible for the absorption of nutrients into the bloodstream. Because the cells of the villi are continually being cast off, new cells must continually arise from the crypts of Lieberkuhn. Being highly mitotic, undifferentiated stem cells, they are very radiosensitive. Thus, the small bowel is the most radiosensitive portion of the GI tract. In the adult, the CNS is the most radioresistant system and the epidermis is composed of mature, postmitotic cells-making them both radioresistant. Chapter 30 Fundamental Principles of Radiobiology ================================================= *Scattering* occurs when there is partial transfer of the photon\'s energy to matter, as in the Compton effect. *Absorption* occurs when an x-ray photon interacts with matter and disappears, as in the photoelectric effect. Our bodies contain a variety of tissues, having a variety of *tissue densities.* These tissues densities afford differing degrees of resistance to the passage of x-ray photons. Tissues having *greater density absorb more* of the x-ray beam (recall photoelectric effect). Soft tissues are fairly easily penetrated to varying degrees; that is, lung and adipose are easier to penetrate than muscle. *Bone* has much higher tissue mass density and therefore absorbs more of the x-ray beam. A*ttenuation* is the reduction in the intensity (quantity) of an x-ray beam as it passes through matter Certain cells are more radiosensitive than others There are some certain factors (physical and biological) that affect the radiosensitivity of biological tissue **Law of Bergonie and Tribondeau 421** ====================================== Bergonie and Tribondeau were French scientists who, in 1906, theorized what has now become verified law. Cells are more radiosensitive if they are *immature* (undifferentiated or stem) cells, if they are *highly mitotic* (having a high rate of proliferation), and if the irradiated tissue is young. Cells and tissues that are still undergoing development are more radiosensitive than fully developed tissues. They theorized in 1906 that all precursor cells are particularly radiosensitive (e.g., stem cells found in bone marrow). There are several types of stem cells in bone marrow, and the different types differ in degree of radiosensitivity. Of these, red blood cell precursors, or erythroblasts, are the most radiosensitive. White blood cell precursors, or myelocytes, follow. Platelet precursor cells, or megakaryocytes, are the least radiosensitive. Myocytes are mature muscle cells and are fairly radioresistant. Nondividing, differentiated cells are specialized, mature cells that *do not undergo mito*sis. Having these qualities, they are rendered radioresistant, according to the theory proposed by Bergonie and Tribondeau. The adult nervous system is composed of non-dividing, differentiated cells, and thus is the most radioresistant system in the adult. Epithelial tissue and lymphocytes contain many precursor stem cells, and hence are among the most radiosensitive cells in the body. The radiosensitivity of living tissue was a function of the metabolic rate of that tissue being irradiated Cells and tissues are usually more sensitive to radiation the more immature or highly mitotic they are. This follows the law of Bergonie and Tribondeau regarding cell sensitivity to radiation. The only exception to this law is the mature lymphocyte, which is said to be the most radiosensitive cell in the human body even though it is highly specialized and a mature end cell. The erythrocyte, osteocyte, and spermatozoa, for example, are all nondividing and mature cells that are highly specialized. The neuroblast is an embryonic cell that is immature and highly mitotic, and therefore it would be the most radiosensitive. **The law states that the radiosensitivity of living tissue varies as follows:** 1. stem cells (immature or less differentiated) are radiosensitive, the more mature a cell is the more resistant to radiation it is, non dividing, differentiated cells do not undergo mitosis, they are more radioresistant -- nerve cells 2. the younger the tissues or organs the more radiosensitive they are 3. when the metabolic activity of a cell is high, the radiosensitivity is also high 4. as the proliferation rate (multiplication rate) increases, radiosensitivity also increases In diagnostic imaging, this reminds us that a fetus is considerably more radiosensitive than a child or mature adult The Law of Bergonie and Tribondeau states that stem cells (which give rise to a specific type of cell, as in hematopoiesis) are particularly radiosensitive, as are *young cells* and tissues. It also states that *cells with a high rate of proliferation* (mitosis) are more sensitive to radiation. This law is historically important in that it was the first to recognize that some tissues have greater radiosensitivity than others (such as the fetus). Cells are frequently identified by their stage of development. *Immature* cells may be referred to as *undifferentiated* or *stem* cells. Immature cells are much more radiosensitive than mature cells. Physical factors affecting radiosensitivity =========================================== When irradiating a biologic medium, the response (tissue damage) is determined by the amount of energy deposited per unit mass -- dose in rads 1. **Linear Energy Transfer (LET)** A measure of the rate at which energy is transferred from ionizing radiation to soft tissue Measures radiation quality and determining the tissue weighting factor (W~T~) -- relative radiosensitivity of various tissues and organs Expressed in units of keV of energy transferred per micrometer of track length in soft tissue (keV/um) The ability of ionizing radiation to produce a biologic response increases as the LET of radiation increases Expresses *the rate at which particulate or photon energy is transferred* to *the absorber.* Because different kinds of radiation have different degrees of penetration in different materials, it is also a useful way of expressing the quality of the radiation. Higher LET = increase of biologic response/damage x rays = 3 keV/um LET depends on: 1\. mass high mass and charge & low speed=more damage 2. charge 3. velocity As it slows down it increases damage Alpha and protons = high LET x and gamma rays = low LET Linear energy transfer (LET) designates the amount of energy that is deposited by radiation in tissue. LET is measured in units of kiloelectron - volts per micrometer (keV/µm). Radiation that has a high LET tends to deposit all of its energy at once. This radiation is therefore not very penetrating but is highly ionizing. Types of radiation that are considered to have a high LET values are ( 1) alpha particles, which may deposit as much as 100 keV/µm; (2) slow neutrons, which may deposit 20 keV/µm; and (3) beta particles, which deposit approximately 12 keV/µm. Comparatively, x- and gamma radiation have very low LET values and are highly penetrating. They have an average LET of approximately 3 keV/µm. Alpha particles deposit all of their energy at once and have a half-value layer of 0.1 mm of human tissue. Beta particles are slightly more penetrating in tissue with a half-value layer (HVL) of about 1 to 2 cm of human tissue. X- and gamma radiation, on the other hand, have an HVL of 10 cm of tissue. In general, low LET radiation, such as x - or gamma, deposits very little energy as it travels through matter and is highly penetrating. Thus, neither, are considered as potentially damaging to biologic tissue as high LET radiation, such as alpha or neutrons. X and gamma radiation are given a quality factor of 1 when converting from rad to rem; this compares to a quality factor of 20 given to alpha radiation. Electromagnetic radiations such as x-rays and gamma rays are considered low LET- radiations because they produce fewer ionizations than the highly ionizing particulate radiations such as alpha particles. *Alpha particles* are large and heavy (two protons and two neutrons), and although they possess a great deal of kinetic energy (approximately 5 MeV), their energy is rapidly lost through multiple ionizations (approximately 40,000 atoms/ cm of air). As an *external* source, alpha particles are almost harmless, because they ionize the air very quickly and never reach the individual. As *internal sources,* however, they ionize tissues, and are potentially the most harmful. It may be stated that the alpha particle has one of the highest LET's of all ionizing radiations. X-radiation used for diagnostic purposes is of relatively *low energy.* Kilovoltages of up to 150 are used, as compared with radiations having energies of up to several million volts. LET (linear energy transfer) refers to the rate at which energy is transferred from ionizing radiation to soft tissue. Particulate radiations, such as alpha particles, have mass and charge, and therefore lose energy rapidly as they penetrate only a few centimeters of air. X- and gamma radiations, having no mass or charge, are *low-LET* radiations. Gamma rays are just like x rays in that both are electromagnetic ionizing radiation. The only difference is their origin. X rays are produced by the sudden deceleration of electrons, whereas gamma rays are emitted from the nucleus of a radioactive atom. All electromagnetic radiation has no mass and no charge; it is simply a form of energy. Because x rays and gamma rays have relatively high energy and no mass or charge, they are highly penetrating and therefore deposit very little energy as they pass through matter. They are much more likely to pass right through tissue rather than to interact at all. This property is called \"low LET". In contrast, radiation with significant mass and/or charge, such as alpha particles, is not very penetrating and therefore is more likely to deposit all of its energy at once, referred to as \"high LET". *Radiation quality* determines degree of penetration and the amount of energy transferred to the irradiated tissue (LET). Obviously, the larger the absorbed radiation dose, the greater the effect. Biologic effect is increased as the size of the irradiated area is increased. The nature of the effect is influenced by the location of irradiated tissue (bone marrow versus gonads and so on). 2. **Relative Biologic Effectiveness (RBE)** When the LET of radiation increases, the ability to produce biologic damage also increases Quantitatively describes the ability of a type of radiation (LET) to cause biologic damage LET expresses the rate at which photon or particulate energy is transferred to (absorbed by) biologic material (through ionization processes); it is dependent on the type of radiation and the characteristics of the absorber. RBE describes the degree of response or amount of biologic change one can expect of the irradiated material. *As the amount of transferred energy (LET) increases* (from interactions occurring between radiation and biologic material), *the amount of biologic effect/damage will also increase* RBE stands for \"relative biologic effectiveness\" and is a formula that is used to compare the differences in biologic effects among various types of radiation. In the formula, a 250- keV x-ray source is the constant by which all other types of radiation may be compared: [Dose of 250-keV x-ray source to produce a biologic effect ] Dose of test radiation to produce the same effect LET increases with the *ionizing* potential of the radiation, for example, alpha particles are more ionizing than x-radiation, therefore they have a higher LET. As ionizations and LET increase, there is greater possibility of an effect on living tissue; therefore, the RBE increases. The *RBE* (sometimes called QF-Quality Factor) *of diagnostic x-rays is* 1 Radiations with a LET lower than diagnostic x rays have a RBE less than one Radiations with a LET higher than diagnostic x rays have a RBE greater than one 3. **Fractionation and Protraction** A large dose of radiation delivered to the whole body all at one time will produce the greatest response If a dose of radiation is delivered over a long period of time rather than quickly, the effect of that dose is less -- OR- If the time between irradiations is lengthened, a higher dose is required to produce the same effect The effects of a quantity of radiation delivered to a body are dependent on the amount of radiation received, the size of the irradiated area, and how the radiation is delivered in time. If the radiation is delivered in portions over a period of time, it is said to be fractionated and has a less harmful effect than if it were delivered all at once, as cells have an opportunity to repair and some recovery occurs between doses. The greatest effect/response from irradiation is brought about by a *large dose of radiation to the whole body delivered all at one time.* Whole-body radiation can depress many body functions. With a fractionated dose, the effects would be less severe because the body would have an opportunity to repair between doses The lengthening of time can be accomplished in two ways: Protraction =========== When the dose is delivered continuously but at a lower rate When a dose of 600 rad is delivered in 3 minutes (200 rad/min), it is lethal for a mouse, but when 600 rad is delivered @ 1rad/hr for 600 hours, it will survive Dose protraction causes less effect because of the lower dose rate and the longer irradiation time **Fractionation** If the 600 rad dose is delivered at 200 rad/min but in twelve equal fractions of 50 rad each separated by 24 hours the mouse will survive Total dose is delivered in fractionated dose with a break in between Dose fractionation causes less effect because tissue repair and recovery occurs between doses Used with oncology (therapy) when radiating tumors Larger *quantities* increase tissue effect. The *energy* (quality, penetration) of the radiation determines whether the effects will be superficial (erythema) or deep (organ dose). *Certain tissues* (such as blood-forming organs, the lens, and the gonads) are more radiosensitive than others (such as muscle and nerve). The *length of time* over which the exposure is spread *(fractionation)* is important; the longer the period of time, the less the tissue effects. **Biologic Factors Affecting Radiosensitivity** There are certain biological conditions that alter the radiation response of biologic tissue Some of these have to do with the inherent state of the host such as age, gender, and metabolic rate There are also artificially induced modifiers of the biologic system 1. **Oxygen Effect** Tissue is most sensitive to radiation when it is *oxygenated. Anoxic* refers to tissue without oxygen; *hypoxic* refers to tissue with little oxygen. Anoxic and hypoxic tumors are typically avascular (with little or no blood supply) and therefore more radioresistant. Biologic tissue is more sensitive to radiation when it is in an oxygenated state. A characteristic of many avascular (and therefore hypoxic) tumors is their resistance to treatment with radiation. Hyperbaric (high-pressure oxygen) therapy is used in some therapy centers in an effort to increase the sensitivity of the tissues being treated. This is described numerically by the oxygen enhancement ratio (OER) OER=[Dose necessary under anoxic conditions to produce a given effect] Dose necessary under aerobic conditions to produce the same effect The OER is also LET dependent, the OER is greatest for low LET radiation, has a maximum of approximately 3; the OER decreases to about 1 for high LET radiation Low LET radiation, the presence of oxygen has a greater effect, high LET radiation, the presence of oxygen has less effect 2. **Age** Humans are most sensitive before birth, the sensitivity then decreases until adulthood when humans are most resistant to radiation induced effects, then in old age humans become more radiosensitive 3. **Gender** Males are more sensitive to radiation by 5-10% than females 4. **Recovery** Human cells are capable of recovering from radiation damage if the dose is not sufficient to kill the cell before its next division (interphase death) 5. **Chemical Agents** For these to be effective they must be present at the time of irradiation 2 types: 1. **Radiosensitizers** Agents that enhance the effects of radiation, ex.-halogenated pyrimidines become incorporated into the DNA and cause the radiation effects to be amplified Can have an effectiveness ratio up to 2 2. **Radioprotectors** They increase their resistance to radiation effects Not used on humans because to be effective they must be administered at toxic levels, the protective agent can be worse than the radiation Compounds with molecules containing the sulfhydryl group (sulfur and hydrogen bound together) Biologic damage will increase in tissues when those tissues have a higher oxygen content, when the tissues have been exposed to a higher LET radiation (radiations that deposit more energy as they penetrate matter), and when the intensity or amount of radiation increases. More energy will be deposited in tissues with a higher LET radiation. However, when using x-radiation, which is considered to be relatively low-LET radiation, absorption will increase in tissue with lower-energy ranges (low-kilovolt \[peak\] techniques) due to the increase in the photoelectric effect. This is why high-kilovolt (peak), low-milliamperage-seconds exposure factors are suggested as a form of radiation protection, but only when the image quality will not be adversely affected. For instance, it would not be wise to use 90 kilovolts (peak) when performing a urographic examination because the x rays may penetrate and \"miss\" being absorbed by a small kidney stone or be attenuated by iodinated contrast media in the ureters. **Radiation hormesis:** Suggests that low levels of radiation \< 5rad provide a protective effect by stimulating molecular repair mechanisms in the human body Radiation Dose-Response Relationships ===================================== A mathematical relationship between different radiation doses and the amount of the observed response 2 applications in radiology: 1. Used to design therapeutic treatment routines for patients with cancer 2. Provide information on the effects of low dose irradiation Every radiation dose/response relationship has two characteristics: 1. Linear or nonlinear 2. Threshold or nonthreshold **Linear (straight line)** - the response is directly related to the dose **Nonlinear-**dose will cause a response but not at a predictable linear level Can have high response up to a point, then little response above that point **Threshold-**dose intercepts the dose (X) axis at a point above 0, at that intersection the response would occur, below that point response would not occur Steeper slope equals a greater (more severe) response to increase The lines do not begin immediately at zero but appear farther to the right on the graph. This means that there must have been a certain minimum dose that had to occur before the response, or radiation-induced effect, developed. **Nonthreshold-**intersects the dose axis at or below 0, indicating that there is no safe dose, that is, *no threshold.* Even one x-ray photon can, theoretically, cause a response. Any dose regardless of size is expected to produce a response, the same effect even a 0 dose (spontaneous occurrence); ambient-natural response level Dose-response curves vary in two basic ways: (1) they are either linear, in which a proportional response is observed in relation to dose, or nonlinear, in which a proportional response is not observed in relation to dose; and \(2) they are either threshold, in which a level is reached below which no effects are observed, or nonthreshold, in which theoretically even a small dose could cause an effect. Diagnostic radiology is concerned primarily with linear, non- threshold dose-response curves because the low doses received mainly cause late effects. Linear dose response relationships ================================== **Linear / Nonthreshold** ========================= Curve A & B, figure 30-4, 0 dose shows a measurable response (ambient) Straight line, but intersects the dose axis at or below 0 Any dose or no dose is expected to produce a response Radiation-induced malignancy, leukemia, and genetic effects are late effects (or stochastic effects) of radiation exposure. These can occur years after survival of an acute radiation dose, or after exposure to low levels of radiation over a long period of time. Radiation workers need to be especially aware of the late effects of radiation because their exposure to radiation is usually low-level over a long period of time. Occupational radiation protection guidelines are therefore based on late effects of radiation according to a linear, nonthreshold dose- response curve. **Linear / Threshold** ====================== Curve C&D - Intercept the dose axis at a value greater than 0 At doses below these values, no response would be expected Any dose above these values would be expected to produce a response D is steeper than C, any increment of dose will produce a larger response on curve D If have a steeper slope, any increment of dose will produce a larger response Non-linear dose response relationships ====================================== **Non linear -- non threshold** **Curve A figure 30-5** - A large response can result from a small radiation dose At high dose levels, the radiation response will be less --an incremental dose at high levels results in less relative damage than the same incremental dose at low levels **Curve B figure 34-5** -- incremental doses in the low dose range result in very little response In the high dose range, incremental doses will produce a large response Non linear -- threshold ======================= **Curve C figure 34-5** -- at doses below D~c~, no response will be measured, as the dose is increased above D~c~, it is increasingly effective until it reaches the inflection point, above this incremental doses become less effective, called a sigmoid type relationship **Diagnostic imaging is concerned with the late effects of radiation exposure and with the** **linear - nonthreshold dose response relationships** The genetic effects of radiation and some somatic effects, like leukemia, are plotted on a *linear* dose-response curve. The linear dose-response curve has *no threshold;* that is, *there* is *no dose below which radiation is absolutely safe.* The *nonlinear/sigmoidal* dose-response curve has a *threshold* and is thought to be generally correct for most *somatic* effects-such as *skin erythema, hematologic depression,* and *radiation lethality* (death). Late or long-term effects of radiation can occur in tissues that have survived a previous irradiation months or years earlier. These late effects, such as carcinogenesis and genetic effects, are \"all-or-nothing\" effects - either the organism develops cancer or it does not. Most late effects *do not have a threshold dose;* that is, *any* dose, however small, theoretically can induce an effect. Increasing that dose will increase the likelihood of the occurrence, but will not affect its severity; these effects are termed *stochastic.* The NCRP categorizes dose limits to anatomic parts based on two types of effects: (1) stochastic and (2) non stochastic or deterministic. *Stochastic effects* are nonthreshold effects that may occur at any dose and are usually long-term late effects, such as cancer and genetic mutations. *Deterministic effects* usually occur above a certain threshold and may be either short term or long term depending on the dose. Examples of non stochastic/deterministic effects include skin erythema, alopecia, drop in the white blood cell count, and cataracts. The NCRP limits many of the very sensitive tissues of the body (bone marrow, thyroid, gonads, etc.) to low doses based on stochastic effects, which may occur at any dose (nonthreshold effects). The limits for more resistant tissues (skin, hands, and feet and lens of the eye) are slightly higher based on deterministic effects. *Nonstochastic effects* are those that will not occur below a particular threshold dose and that increase in severity as the dose increases. Biologic effects that increase in severity with the dose (that is, the effects are more severe with a higher dose than with a lower dose) are called deterministic or nonstochastic effects. Examples are (1) skin erythema that becomes progressively worse as the dose increases and (2) cataracts that have a threshold of 2 Gy (200 rad). In the latter case, the entire lens can become opacified, which may lead to complete blindness at doses of 1000 rad or more. According to the latest NCRP Report (No. 116), deterministic effects are those typically associated with a threshold dose. The old term associated with these types of effects was \"nonstochastic.\" Cataracts to the lens of the eye may be induced by a threshold dose of 2 Gy (200 rad). Genetic defects, leukemia, and thyroid cancer etc., are considered to be stochastic effects. These effects may occur at any dose. Stochastic effects are nonthreshold effects and are the basis for total body dose-equivalent limits and the ALARA philosophy. Deterministic effects = nonstochastic effects = threshold dose = cataracts = early or late effects Nondeterministic effects = stochastic effects = nonthreshold dose = may occur at any dose = genetic defects & cancer = late effects The whole-body dose limit is based on stochastic effects that may occur at very low doses, such as cancer and genetic effects. It is set at the lowest limit (50 mSv \[5 rem\]) 5rem =.05Sv = 50mSv, because whole-body doses are potentially more harmful than local tissue exposures. Some local tissues are allowed higher dose-equivalent limits based on deterministic effects. Examples are the lens of the eye, which is limited to an annual dose equivalent of 150 mSv (15 rem), and the extremities, which are allowed an equivalent of 500 mSv (50 rem). When reading dose-response curves on a graph, the horizontal axis represents the dose received, whereas the vertical axis represents the effects that may be observed. There are two broad categories of curves: (1) threshold and (2) non- threshold. There are also three distinct shapes that these curves can take: (1) linear, (2) linear quadratic, and (3) sigmoidal. A *linear curve* is one represented by a straight line. Curves A, B, and C are all linear curves because they are all straight lines. Curves A and B are both nonthreshold because the radiation effects are immediately noticeable above a dose level of zero on the graph. Curve A starts out higher on the vertical axis because some effects may occur naturally in a population prior to any radiation exposure (e.g., sterility, cancer, etc.). Curve C will begin to show an effect above a specific threshold. An example of a threshold effect would be cataracts because this effect is induced only by radiation doses exceeding 200 rads. **Constructing a Dose -- Response relationship** Its nearly impossible to measure low-dose, late effects, and that is the area of greatest concern to diagnostic imaging Scientists therefore irradiate animals with very large doses hoping to observe a significant response The principal interest in diagnostic imaging is to estimate the response at very low radiation doses The NCRP determines its dose-equivalent limits based on the linear, nonthreshold dose curve ( curve B), meaning that even a very small dose can cause certain types of biologic damage. This can't be done directly so they **extrapolate** - infer values of a variable in an unobserved interval from values within an already observed interval OR predict by projecting past experience or known data - the dose response relationship from the high dose known region to the low dose unknown region An extension of a pattern achieved through experimentation data from results achieved at a specific level Results in a linear-non threshold dose-response relationship, may not be correct because of certain conditions Linear, quadratic dose response relationship ============================================ Committee on the Biological Effects of Ionizing Radiation (BEIR) Did study on the effects of low LET radiation The effects of radiation exposure follow a linear, quadratic dose response relationship Their finding is that the linear non threshold relationship overestimates the risk associated with diagnostic radiation Scientists and physicists use the linear non threshold relationship since it does overestimate the effects