Notebook – Respiratory System PDF
Document Details
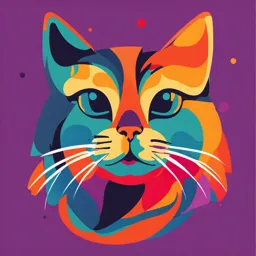
Uploaded by GreatestTucson
Tags
Summary
This document is a notebook on the respiratory system. It covers the anatomy of the respiratory system, including the nose, pharynx, larynx, trachea, bronchi, bronchioles, and alveoli. It also outlines the functions of the respiratory system, such as gas exchange, movement of air, protection from pathogens, and sound production.
Full Transcript
Notebook – Respiratory System 2024-08-29 2:45 PM Class 1: Anatomy of the Respiratory System What is the Respiratory System? All of the structures involved in breathing (pulmonary ventilation) and external respiration...
Notebook – Respiratory System 2024-08-29 2:45 PM Class 1: Anatomy of the Respiratory System What is the Respiratory System? All of the structures involved in breathing (pulmonary ventilation) and external respiration Pulmonary ventilation – airflow to/from the lungs External respiration – gas exchange between the lungs and pulmonary circulation Internal respiration Gas exchange between systemic circulation and the tissues Homeostasis requires Steady supply of O2 and a constant elimination of CO2 Disruption = oxygen starvation & waste buildup = rapid cell death 2 systems work together to make sure that our cells don’t die Respiratory system provides for gas exchange Cardiovascular system transports the respiratory gases Functions: Providing an extensive surface area for gas exchange between air and circulating blood Moving air to and from the exchange surfaces of the lungs along the respiratory passageways Protecting respiratory surfaces from dehydration, temp changes, and invasion by pathogens Producing sounds for speaking, singing, and other forms of communication Detecting odors by olfactory receptors in the superior portions of the nasal cavity Consists of: 1. Nose 2. Pharynx 3. Larynx 4. Trachea 5. Bronchi 6. Bronchioles 7. Alveoli Respiratory Tract: Respiratory tract Branching passageway that carries air to/from gas exchange surfaces of the lungs 2 divisions of respiratory tract: 1. Conducting portion Nasal cavity to larger bronchioles No gas exchange 2. Respiratory portion Smallest bronchioles: respiratory bronchioles to alveoli Where gas exchange occurs Can also divide into the upper respiratory tract and lower respiratory tract Upper Respiratory Tract: Anatomical structures Nose Nasal cavity Paranasal sinuses Pharynx Functions: Filters, warms, and humidifies incoming air Protects delicate lower tract Reabsorbs heat and water in outgoing air Lower Respiratory Tract: Anatomical structures Larynx Trachea Bronchi Bronchioles Alveoli Functions: Conducts air to and from gas exchange surfaces The Respiratory Epithelia: The specific type of epithelia varies along the respiratory tract 1. Respiratory mucosa Lines the nasal cavity and superior pharynx Also lines the superior portion of the lower respiratory tract 2. Stratified squamous epithelium Lines inferior portions of pharynx 3. Simple cuboidal or simple columnar Lines the smaller bronchioles 4. Simple squamous epithelium Forms gas exchange surfaces Distance b/w air and blood in capillaries is less the 1 um Respiratory Mucosa Respiratory mucosa lines nasal cavity through larger bronchioles Pseudostratified ciliated columnar epithelium with mucous/goblet cells Lamina propria underlying areolar tissue supports respiratory epithelium mucous glands in trachea and bronchi Mucociliary escalator Flow of mucus/trapped debris Sticky mucus produced by mucous cell and mucous glands Traps debris particles Moved by cilia Swept toward pharynx Swallowed (to acids in stomach) or coughed out Epithelial stem cells replaced damaged/old cells Upper Respiratory Tract: The Nose Nose Primary route for air entering respiratory system External nose is the portion you can see Nostrils or external nares Paired opening into nasal cavity Bony and cartilaginous structures make up the framework of the external nose Bony framework of the external nose Dorsum of nose (bridge) formed by 2 nasal bones Maxilla Frontal bones Cartilaginous framework of the external nose Nasal cartilages: small, elastic cartilages extending laterally from bridge; help keep nostrils open Septal cartilage Lateral nasal cartilage Alar cartilage The Nasal Cavity Superior border Ethmoid bone Inferior border Hard palate, made of palatine bones and palatine process of maxillae Medial border Nasal septum Lateral border Ethmoid bone Maxillae lacrimal bones palatine bones inferior nasal conchae bones Majority lined w/ respiratory mucosa Anteriorly merges with external nares Posteriorly communicates with the nasopharynx through the choanae Nasal vestibule lined with course hairs for filtering large dust particles Functional divisions of the Nasal Cavity 1. Respiratory Region Larger, inferior region of nasal cavity Lined w/ non-keratinized pseudostratified ciliated columnar epithelium with many goblet cells (respiratory mucosa) 2. Olfactory region Smaller, superior region of nasal cavity Olfactory receptors near superior nasal concha Have cilia, but no goblet cells Structures of the Nasal Cavity Nasal septum Divides R and L nasal cavities Formed by: - nasal cartilage - vomer - perpendicular plate of the ethmoid Superior, middle and inferior nasal conchae (bones) Superior, middle, and inferior nasal meatuses Passages b/w nasal conchae Swirl incoming air to trap small particles Moves odorants to olfactory receptors Warms/humidifies air Paranasal Sinuses 1. Frontal 2. Ethmoid 3. Maxillary 4. Sphenoidal sinuses Open into nasal cavity Functions Mucus secreted by sinuses moisten nasal cavity Resonate sound Lighten skull Nasal polyps Outgrowths of mucous membranes Usually found around opening to paranasal sinuses Nasolacrimal duct The lacrimal sac drains tears from the eyes The nasolacrimal duct carries tears from the lacrimal sac of the eye into the nasal cavity Lamina Propria Lamina propria (basement membrane) of nasal cavity has extensive network of vessels Release heat to warm inhaled air Water from mucus evaporates to humidify inhaled air Air moving from nasal cavity - heated to almost body temperature - nearly saturated w/ water vapor The reverse process occurs during exhalation mucosa reabsorbs heat and water; reduces heat loss an water loss to environment Mouth breathing eliminates these benefits Upper Respiratory Tract: The Pharynx Pharynx Pharynx is shared by respiratory and digestive systems Colloquially referred to as the throat 5 inch muscular tube from choana ---> cricoid Lined w/ respiratory mucosa Functions: Passage for food and air Resonating chamber for speech Lymphatic tissue (tonsil) to prevent the entry to the body 3 regions of the pharynx: 1. Nasopharynx 2. Oropharynx 3. Laryngopharynx Nasopharynx Superior part of the pharynx Lined w/ respiratory mucosa From choanae ---> soft palate Has pharyngeal openings of the auditory tubes - Eustachian tubes Contains adenoids - pharyngeal tonsils Oropharynx Middle part of the pharynx behind oral cavity Lined with non-keratinized stratified squamous epithelium From soft palate ---> base of the epiglottis Laryngopharynx Inferior part of the pharynx Lined w/ non-keratinized stratified squamous epithelium From epiglottis ---> cricoid cartilage Lower Respiratory Tract: The Larynx The Larynx Cartilaginous tube that surrounds/protects glottis ("voice box") Connects the pharynx w/ the trachea Anterior to C4 – C6 Three large hyaline cartilage make up the larynx 1. Epiglottis 2. Thyroid cartilage 3. Cricoid cartilage 1. Epiglottis Projects superior to glottis, forms lid over it During swallowing the larynx elevates, the epiglottis folds back over glottis, and blocks entry into respiratory tract 2. Thyroid Cartilage Prominent anterior surface is laryngeal prominence - adam's apple Thyrohyoid ligament attaches it to hyoid bone; other ligaments attach it to epiglottis and smaller cartilages 3. Cricoid Cartilage Forms complete ring around larynx W/ thyroid cartilage, protects glottis and larynx Provides attachment for laryngeal muscles/ligaments Landmark for tracheotomy Other Cartilaginous Structures Arytenoid Cartilages (2) Change position & tension of vocal cords via synovial joint w/ cricoid cartilage Corniculate Cartilages (2) Elastic cartilage Cuneiform Cartilages (2) Support vocal fold and lateral epiglottis Glottis Where air passes through larynx Made of Vocal folds/cords Rima glottidis (opening b/w folds) Vocal folds/vocal cords = tissue folds that contain vocal ligaments Vibrations produce sound waves Opened/closed by rotation of arytenoid cartilages Also known as the vocal cords Producing sound Bands of elastic ligaments stretched b/w laryngeal cartilages Muscle contract = cartilages move = pulls ligaments tight = stretched vocal folds out into airway – rima glottidis narrows = air passes through folds and they vibrate = sound Pitch Depends on tension of vocal folds Increase taut = rapid vibration = increase pitch Androgens – thicker & longer vocal folds = decrease pitch Volume Depends on the pressure of air Phonation Sound production from larynx Vibration of vocal cords produces sound waves Articulation Modification of sounds by tongue, teeth, and lips Amplification and resonance occur in pharynx, oral and nasal cavities, and paranasal sinuses Ventricular folds Aka false vocal cords Above true vocal cords Space b/w = rima vestibuli Useful for holding breath against thoracic cavity pressure Epithelium of the Larynx Superior to vocal folds Non-keratinized stratified squamous epithelium Inferior to vocal folds Pseudostratified ciliated columnar epithelium w/ goblet cells (respiratory mucosa) Lower Respiratory Tract: The Trachea Trachea Trachea or the "windpipe" starts at C6 and ends at T5 by branching into bronchi 5 inches long 1 inch in diameter Lies in front of the esophagus The trachea, bronchi, and bronchial branches convey air to and from lung gas exchange surfaces Has 15 – 20 C-shaped tracheal cartilages Prevent collapse and overexpansion Allow esophagus to expand slightly into tracheal space Carina ridge at the base of the trachea that separates the opening of the right and left main bronchi highly innervated mucosa very sensitive cough reflex to prevent choking End of each C-shaped tracheal cartilage connected by elastic ligament and trachealis (muscle) Contraction of trachealis narrows trachea; restricts airflow Tracheal diameter changes often, mostly controlled by sympathetic stimulation which increases airflow Tracheal cartilages are incomplete posteriorly allowing for expansion when swallowing Layers of the Trachea Mucosa Pseudostratified ciliated columnar epithelium w/ goblet cells (respiratory mucosa) Sub-mucosa Loose connective tissue with seromucous glands Fibromuscular membrane w/ smooth muscle and elastic CT (includes trachealis mm's) Allow tracheal diameter change during inhalation/exhalation Adventitia = connective tissue Binds trachea to other organs Lower Respiratory Tract: Bronchi Primary Bronchi Aka main bronchi 1st division of bronchi Right and left bronchus go into each lung Right bronchus wider, at a steeper angle, and shorter than left foreign objects in trachea often go into it Have complete cartilaginous rings Lined w/ respiratory mucosa Secondary bronchi Aka Lobar bronchi One to each lobe of the lung 3 lobar bronchi on the RIGHT 2 lobar bronchi on the LEFT Smooth muscle encircles lumen and increasingly replaces cartilage Lined w/ respiratory mucosa Tertiary Bronchi Aka Segmental bronchi Each lung has approx 10 segmental bronchi Each one supplies triangular shaped unit of lung (bronchopulmonary segment) Smooth muscle encircles lumen and increasingly replaces cartilage Lined w/ respiratory mucosa Bronchioles No cartilage; thick smooth muscle Ciliated simple columnar epithelium w/ globlet cells Ciliated simple cuboidal epithelium w/o goblet cells (club cells or clara cells) Sympathetic nervous system (NE/E) causes bronchodilation Increases airflow Parasympathetic nervous system causes bronchoconstriction Decreases airflow Histamine, asthma attack, allergies Extreme bronchoconstriction can occur during allergic reactions such as asthma Terminal bronchioles lead to pulmonary lobules (gas exchange) Respiratory bronchioles are last division Terminal Bronchioles Many terminal bronchioles Each terminal bronchiole supplies a pulmonary lobule Smooth muscle (no cartilage) = airway patency vulnerable to muscle spasms Non-ciliated simple columnar epithelium Macrophages remove debris (no cilia to move mucous) Respiratory Bronchioles Many, many respiratory bronchioles Simple cuboidal & simple squamous epithelium First place where external respiration can occur, although limited Review of the Bronchial Tree 1. Trachea – larynx to main bronchi in mediastinum 2. Main bronchi – one to each lung; cartilage rings are complete 3. Lobar bronchi – 3 in right lung, 2 in left; one per lobe 4. Segmental bronchi – branch to give rise to bronchioles 5. Bronchioles 6. Terminal bronchioles 7. Respiratory bronchioles Gross Anatomy of the Lungs: Each lung divided into lobes: Right lung (3) superior lobe, middle lobe, inferior lobe - right lung is shorter due to liver Left lung (2) superior lobe and inferior lobe - left lung is 10% smaller due to heart Each lobe has multiple bronchopulmonary segments Each lung is cone shaped and divided into lobes by deep fissures 1. Right lung (2 fissure) horizontal fissure between superior/middle lobes oblique fissure b/w middle/inferior lobes 2. Left lung (1 fissure) oblique fissure b/w superior/inferior lobes Apex (tip) extends to superior border of 1st rib Concave base rests on diaphragm Grooves on surface of lungs mark positions of great vessels Cardiac Notch left lung accommodates pericardium/heart Root of the lung Dense connective tissue fixes positions of bronchi, major nerves, blood vessels, and lymphatics Hilum Is a medial depression on each lung Allows passage of main bronchus, pulmonary vessels, nerves, lymphatics Pleurae Serous membrane sacs surrounding the lungs Visceral pleura Covers outer surfaces of lungs Parietal pleura Covers inner surface of thoracic wall; extends over diaphragm and mediastinum Pleural cavity Potential space b/w visceral and parietal layers of pleural sac Contains pleural fluid that reduces friction of the lungs against the wall Conditions involving the Pleural Cavity: Pneumothroax Pleural cavity fills w/ air Common cause is chest trauma Hemothorax Pleural cavity fills w/ blood Hydrothroax Collection of serous fluid m/c cause is cardiac failure Empyema Pus in the pleural cavity m/c cause is pneumonia ***Any may cause partial or complete lung collapse*** Microscopic Anatomy of the Lungs: Each terminal bronchiole supplies a single pulmonary lobule Pulmonary lobules contain: A terminal bronchiole Venule, arteriole, lymphatics, capillaries, multiple alveolar sacs Pulmonary lobules are wrapped in elastic CT Each terminal bronchiole branches into multiple respiratory bronchioles Respiratory bronchioles ---> alveolar ducts ---> alveolar sacs made of alveoli External respiration occurs in respiratory bronchioles alveoli Pulmonary Alveoli 150 million alveoli (singular, alveolus) per lung; give lungs an open, spongy appearance Surrounded by extensive capillary network for gas exchange Surrounded by elastic fibers – expansion/recoil aids air movement Each alveolar ducts ends in clusters of alveoli (alveolar sacs, or alveolar saccules) Alveolar Epithelium 1. Type 1 Pneumocytes Simple squamous epithelium Thin, delicate, sites of gas diffusion 2. Type 2 Pneumocytes Produce surfactant – only secretion; reduces surface tension of water in alveoli to prevent collapse 3. Roaming alveolar macrophages locate and phagocytize particles that could clog the alveoli Blood air barrier Where gas exchange occurs b/w blood and alveolar air Aka alveolar-capillary membrane or respiratory membrane Three layers: 1. Alveolar cell layer (epithelium) 2. Fused basement membranes (alveolar and capillary) 3. Capillary endothelium Minimal distance separating air and blood (average 0.5 um) allows for rapid diffusion Large total surface area (70-100 m2) also allows for a large amount of diffusion Class 2: Respiratory Physiology Respiratory Physiology: Pulmonary Ventilation Air movement in/out of lungs – BREATHING Maintains alveolar ventilation air movement in/out of alveoli Respiration Two integrated processes: 1. external respiration 2. Internal respiration External respiration Exchange of gases b/w Blood, Lungs & External environment Gas diffusion occurs across blood air barrier b/w alveolar air and alveolar capillaries Abnormalities affecting external respiration affect gas concentrations in interstitial fluids and cellular activities Hypoxia = low tissue oxygen levels - severely limits metabolic activities Anoxia = no oxygen supply - much of damage caused by heart attacks and strokes is the result of localized anoxia Internal respiration Occurs b/w blood and tissues Absorption of oxygen from blood into tissues Release of carbon dioxide by tissue cells into blood Physiology of Pulmonary Ventilation: Pressure Molecules in a gas bounce around independently When contained, collisions with container wall cause pressure More collision = higher pressure Boyle's Law More collisions occur when molecules are in smaller container Pressure is inversely related to volume ( P = 1/V ) In plain terms: - decreased volume = more collisions = higher pressure - increase volume = less collisions = lower pressure Recall diffusion – very similar with pressure Gases will move from an area of high pressure to low pressure Gases will move down their pressure gradient Direction of airflow (into or out of lungs) determined by pressure difference between: 1. Atmospheric pressure – pressure of air around us 2. Intrapulmonary pressure – pressure inside respiratory tract, usually measured at the alveoli Pulmonary ventilation involves changing volume of the thoracic cavity Movement of the diaphragm and rib cage change the volume of the thoracic cavity, which expands or compresses the lungs (changes lung volume) Change in volume = change in pressure (boyle's law) Atmospheric pressure = 760 mmHg Start of a Breath Pressures inside and outside thorax are identical; no air movement Expanding thoracic cavity expands lungs Parietal pleura attached to thoracic wall visceral pleura to lungs Pleural fluid forms bond b/w layers due to surface tension During inhalation Thoracic cavity enlarges Increased volume causes decreased pressure Pressure inside lungs drops below atmospheric pressure (P outside > P inside) Air moves into lungs from an area of high pressure to low pressure Inhalation Intrapulmonary pressure < atmospheric pressure Negative intrapulmonary pressure pulls air into lungs During exhalation Thoracic cavity decreases in volume Decreased volume causes increased pressure Pressure inside lungs increases above atmospheric pressure (P outside < P inside) Air is forced out of the lungs from an area of high pressure to low pressure Exhalation Intrapulmonary pressure > atmospheric pressure Positive intrapulmonary pressure pushes air out of lungs Respiratory Muscles and Pulmonary Function: Respiratory Muscles May be involved with inhalation (inspiratory mm) or exhalation (expiratory mm) Breathing can either be quiet breathing or forced breathing Quiet breathing – normal breathing Forced breathing – laboured breathing Quiet breathing occurs through: Active inhalation via primary inspiratory muscles Passive exhalation via elastic recoil of tissues, not by muscle action Forced breathing requires Accessory respiratory muscles Inspiratory muscles Primary inspiratory muscles – used for quiet inhalation Accessory inspiratory muscles – used for forced inhalation Primary inspiratory muscles: Diaphragm External Intercostals Diaphragm does 75% of movement – flattens floor of thoracic cavity External intercostals does 25% of movement – elevates ribs and pull out Accessory inspiratory muscles Increase speed/amount of rib movement to move more air when needed (tissue oxygen demands not met by primary inspiratory muscles) SCM Scalenes Pectoralis minor Serratus anterior Expiratory muscles There are no primary expiratory muscles Quiet exhalation is a passive process done by elastic recoil Diaphragm relaxes Dome moves superiorly External intercostals relax Ribs move down Passive elastic recoil caused by: Stretched elastic fibers Inward pull of surface tension from alveolar fluid Accessory expiratory muscles Internal intercostals transversus thoracic external oblique internal oblique rectus abdominis - abdominal muscles push diaphragm upward - decrease thoracic cavity volume quickly - allow greater pressure change and faster airflow out of lungs Internal intercostals and transversus thoracic - depress ribs Factors Affecting Pulmonary Ventilation: 1. Surface tension 2. Compliance 3. Airway resistance Surface Tension Water molecules attracted to each other more strongly than to air molecules This is why soap bubbles collapse inward and burst Thin layer of alveolar fluid coats luminal surface of alveoli Responsible for 2/3 of elastic recoil of lungs Must be overcome during inhalation Surfactant – from type 2 pneumocytes Reduces surface tension below simple water Surfactant is not produced until 24th - 28th week of development Premature infants have decreased surfactant Increased surface tension = alveoli collapse = great effort to open alveoli w/ each inhalation This leads to something called RDS – respiratory distress syndrome Treatment – intubation, ventilation, artificial surfactant Compliance Compliance – the ability of the lungs and chest wall to expand Increased compliance Lungs & chest wall expands easily Elastic stretches easily and elastic recoil is not strong making exhalation more difficult Seen with some obstructive lung diseases – emphysema Decreased compliance Lungs and chest wall difficult to expand This is restrictive lung disease - pulmonary fibrosis (asbestosis, TB) - pneumothorax - pleural effusion - chest wall injury or trauma - scoliosis - respiratory distress syndrome (RDS) - obesity and pregnancy Airway resistance Airway resistance – the resistance of the respiratory tract to airflow during inhalation and exhalation Naturally, because of pressures Bronchioles expand during inhalation = decreased resistance Bronchioles narrow during exhalation = increased resistance Obstructive lung diseases are characterized by increased airway resistance, such as: Asthma Chronic obstructive pulmonary disease (COPD) - chronic bronchitis - emphysema Lung Pathology - Restrictive vs. Obstructive Lung Disease: Restrictive Lung Diseases Include diseases that make it hard for the lungs to expand fill with air (low compliance) pulmonary fibrosis (asbestosis, TB) pneumothorax pleural effusion chest wall injury or trauma scoliosis respiratory distress syndrome (RDS) obesity and pregnancy Obstructive Lung Diseases Include disease that make it hard for people to expel air from the lungs (increased resistance) Chronic Obstructive Pulmonary Disease (COPD) - chronic bronchitis - emphysema Asthma Lung Pathology - Restrictive Lung Disease: Pulmonary fibrosis (asbestosis, TB) Pneumothorax Pleural effusion Chest wall injury or trauma Scoliosis Respiratory distress syndrome (RDS) Obesity and pregnancy Lung Pathology - Obstructive Lung Disease: Chronic Obstructive Pulmonary Disease (COPD) General term for progressive disorder of the airways that restricts airflow and reduces alveolar ventilation Two examples of COPD that frequently occur together 1. Chronic bronchitis 2. Emphysema 1. Chronic Bronchitis Long-term inflammation and swelling of bronchial lining; leads to overproduction of mucus Frequent cough, lots of sputum can clog airways, increasing resistance, reduced efficiency Cigarette smoking – most common cause Also other environmental irritants 2. Emphysema Chronic, progressive condition Alveolar walls are damaged Loss of elastic tissues increases compliance Causes alveolar sacs to collapse Loss of respiratory surface area restricts oxygen absorption (shortness of breath, intolerance of physical exertion) Strongly linked with cigarette smoking Asthma (asthmatic bronchitis) Characterized by conducting passageway that are extremely sensitive to irritation Airways respond by constricting smooth muscles along bronchial tree, edema/swelling of mucosa, increased mucus Breathing difficult; resistance markedly increased Triggers include allergies, toxins, exercise Breathing Patterns: Eupnea Normal variation in breathing rate and depth (12 – 18 breaths per minute) Apnea Temporary cessation of breath (sleep apnea) Dyspnea Painful, difficult, or laboured breathing (SOB) Tachypnea Rapid breathing rate (> 20 breaths per minute) Costal breathing Upward & outward movement of chest during contraction of intercostals Diaphragmatic breathing Abdomen moving outward when contracting diaphragm Modified Breathing Patterns: Coughing Deep inspiration, closure of rima glottidis & strong expiration blasts air out to clear respiratory passage Sneezing Muscles of expiration spasmodically contract, pushing air out of nose and mouth Hiccupping Spasmodic contraction of diaphragm & quick closure of rima glottidis produces sharp inspiratory sound Yawning Significant amount of inhaled air followed by quick exhalation Valsalva Forced expiration against closed rima glottidis (increased abdominal pressure) Sobbing Many convulsive inhalations (where rima glottidis prematurely closes, letting in only a little air) and a long exhalation Lung Volumes: Respiratory volumes are measured with a spirometer Can be shown on a spirogram Generally larger volumes in Males Taller people Younger people Lung volumes: 1. Tidal volume 2. Inspiratory reserve volume (IRV) 3. Expiratory reserve volume (ERV) 4. Residual volume 5. Minimal volume Aka TIMER 1. Tidal volume Amount of air moved in or out of lungs during a single respiratory cycle at rest - normal quiet breathing Averages 500 mL - 1/2 a liter Standard breathing 70% reaches respiratory zone for external respiration (350 mL) 35% remains in conducting airways = anatomic (respiratory) dead space (150 mL) 2. Inspiratory reserve volume (IRV) Amount of air you can breathe in beyond tidal volume Get to the top of a tidal breath, and everything you can breathe in after that 3. Expiratory reserve volume (ERV) Amount of air you can exhale beyond tidal volume (after normal exhalation) Get to the bottom of a tidal breath, and breath out everything after that 4. Residual volume Amount of air left in lungs after maximal exhalation (1200 mL) 5. Minimal volume Amount of air in the lungs if they were allowed to collapse Included in residual volume Cannot be measured in a healthy person When you die you will always have some air in the lungs – that is this volume Lung Capacities: Lung capacities cannot be measured directly but are calculated by taking sum of various respiratory volumes Lung capacities: 1. Inspiratory capacity 2. Vital capacity 3. Functional residual capacity (FRC) 4. Total lung capacity Aka FITV 1. Inspiratory capacity Tidal volume + IRV Amount of air you can inhale after normal exhalation 2. Vital capacity ERV + Vt + IRV Maximum amount of air you can move in or out of lungs per cycle 3. Functional residual capacity (FRC) ERV + residual volume Amount of air remaining in lungs after complete quiet cycle 4. Total lung capacity Vital capacity + residual volume Total volume of lungs Averages 6000 mL in adult males and 4200 mL in adult females Pulmonary Function Tests (PFTs): Pulmonary function tests (PFT) measure volume, capacities, and rates of flow Pulmonary function tests: 1. Force vital capacity (FVC) 2. Forced expiratory volume (FEV) 3. Forced expiratory flow (FEF) 4. Peak expiratory flow rate (PEFR) Aka FFFP 1. Force vital capacity (FVC) Measures how much air you can forcibly exhale after taking a deep breath 2. Forced expiratory volume (FEV) This is the amount of air expired during the first, second, and third seconds of the Force vital capacity test (FEV 1, FEV 2, FEV 3) 3. Forced expiratory flow (FEF) This is the average rate of flow during the middle half of the Force vital capacity test 4. Peak expiratory flow rate (PEFR) This is the fastest rate that you can force air out of your lungs Effects of Aging and Smoking on the Lungs: Aging All aspects of respiratory function decrease with age As elastic tissue deteriorates, vital capacity decreases Arthritis stiffens rib joints, reducing compliance and maximum respiratory minute volume Some degree of emphysema is normal for people over age 50 Extent varies widely with exposure to cigarette smoke and other irritants Respiratory function declines more with more years of smoking Lung cancer Aggressive class of malignancies Derived from epithelial cells in conducting passages, mucous glands, alveoli Signs and symptoms often not present until tumours restrict airflow or compress adjacent structures - chest pain, SOB, cough/wheeze, weight loss Causes more deaths per year than any other type of cancer 85 – 90% of lung cancer cases are direct result of cigarette smoking Smoking Effects of smoking Cigarette smoke contains several carcinogens (cancer-causing agents) Mucus and cilia in normal respiratory epithelium clean inhaled air Irritants/carcinogens in smoke cause progressive series of changes in the epithelium Dysplasia Reversible Cells damaged, and functional characteristics change Cilia damaged and paralyzed – causes local buildup of mucus Epithelium becomes less effective at protecting deeper, delicate parts of respiratory tract Metaplasia Reversible Tissue changes structure Stressed respiratory surface converts to stratified epithelium - protects underlying layers but not deeper parts of tract - may be reversed if stimulus is removed before further damage Neoplasia Irreversible growth of abnormal cells forms a cancerous tumour (neoplasm) Anaplasia Irreversible most dangerous stage, cells become malignant and metastasize to other parts of body Class 3: External and Internal Respiration Gas Laws: Principles that govern the movement and diffusion of gas molecules The atmosphere is a mixture of gases Total atmospheric pressure at sea level is 760 mm Hg Partial pressure (P) = pressure exerted by single gas in mixture Daltons law Gas mixture inside respiratory tract varies by location Inhaled air gets moistened and warmed In alveoli, it mixes with air remaining from previous breath Exhaled air mixes with air in anatomic dead space As gas mixture varies, so do the partial pressure of its component gases Boyle's Law (P1V1=P2V2) Pressure and volume have an inverse relationship Determines direction of air movement during pulmonary ventilation Decreasing volume ---> increases collisions & increases pressure Daltons Law All the partial pressures of gases added together = the total pressure exerted by the gas mixture Add up nitrogen, oxygen, carbon dioxide, and H20 it will = 760 mm Hg (atmospheric pressure) Henry's Law At a given temperature, the amount of a particular gas in solution is directly proportional to the partial pressure of that gas above the liquid Also dependent on the solubility that gas in the solution External Respiration: Blood arriving in pulmonary capillaries has lower P o2 and higher P co2 than in alveolar air Oxygen has been used in the tissues Carbon dioxide has been created in the tissues and released in the blood Diffusion between alveolar gas and pulmonary capillaries: Increase blood P o2 (oxygen enters blood) Decreases P co2 (carbon dioxide leaves blood) Internal Respiration: P o2 of blood leaving lungs in pulmonary veins drops slightly when it mixes with blood from capillaries around conducting passageways; still higher than P o2 of interstitial fluid Oxygen diffuses to interstitial fluid P co2 higher in tissues/interstitial than in blood Carbon dioxide diffuses from tissues into blood What do we do with the oxygen transferred into cells? Cellular respiration = using oxygen inside the cell to create ATP Gas Transport in the Blood: Oxygen Transport in Blood Each 100 mL of blood leaving alveoli carries 20 mL oxygen Only 0.3 mL (1.5%) is dissolved in the plasma Remaining 19.7 (98.5%) is bound to iron ions in heme units of hemoglobin (Hb) 4 molecules of oxygen per hemoglobin molecule Each hemoglobin molecule is made of 4 globular proteins, each with one heme unit Each hemoglobin molecule can reversibly bind up to four molecules of oxygen; forms oxyhemoglobin (HbO2) Carbon monoxide (CO) is dangerous because it also bind to heme units, making them unavailable for O2 transport Hemoglobin Saturation Percentage of heme units containing bound oxygen at any moment Oxygen-hemoglobin saturation curve A graph showing hemoglobin saturation at different partial pressures of oxygen Shape reflects hemoglobins increased affinity for oxygen with each oxygen molecule bound - increases steeply until it plateaus near saturation - hemoglobin is > 90% saturated with oxygen when Po2 is above 60 mm Hg Hemoglobin in blood entering systemic circuit is 97% saturated Po2 is 95 mm Hg Hemoglobin in blood leaving body tissues is 75% saturated Po2 is 40 mm Hg Hemoglobin in blood in active muscle is only 20% saturated Large amounts of oxygen being released to tissue Po2 is only 15 – 20 mm Hg Can be measured to determine respiratory function using a pulse oximeter An ideal hemoglobin saturation (SpO2) is between 96% and 99% An SpO2 of 90 – 95% requires investigation An SpO2 of 80 – 89% requires urgent medical treatment An SpO2 of < 80% is potentially fatal (don’t need to know any numbers) Shifting the Oxygen-Hemoglobin Saturation Curve: A shift in the curve represents a change in the affinity for O2 A shift to the right (red curve) Means oxygen is being more easily released from hemoglobin A shift to the Left (blue curve) Means oxygen is more tightly bound to hemoglobin Shifting the oxygen-hemoglobin saturation curve is caused by: 1. PH changes 2. Temperature changes 3. Changes in partial pressure of CO2 (Pco2) 4. Changes in concentration of 2, 3-bisphosphoglycerate (BPG) PH changes Blood pH directly affects hemoglobin saturation (Bohr effect) H+ binds to hemoglobin & alters it, decreasing the Hb affinity for O2 pH decreases (acidic) Saturation curve shifts to the right pH increases (basic) Saturation curve shifts to the left Temperature changes Higher temperature leads Hb to release oxygen more readily (right) Especially important in active tissues (generate heat) Changes in Partial Pressure of CO2 (Pco2) Increased Pco2 will shift the curve to the right High levels of CO2 will decrease the pH of the blood Decreased pH decreases the affinity Hb affinity for O2 Therefore, Increase metabolism, leads to increased CO2, leads to decreased pH, leads to more oxygen available for the tissues Changes in concentration of 2, 3-bisphosphoglycerate (BPG) A possible byproduct of glycolysis is 2, 3-bisphosphoglycerate (BPG) RBCs make BPG during glycolysis In normal oxygenation, BPG is rapidly consumed, but in low oxygen areas, BPG will build up BPG can bind deoxyhemoglobin and stop O2 from binding hemoglobin The higher the BPG, the more oxygen will be available for the tissues (right) Fetal Hemoglobin Has a slightly different structure than adult hemoglobin Has a higher affinity for O2 (a left shift) This higher affinity allow fetal RBCs to pull more oxygen from the mother as it binds it more strongly Carries up to 30% more oxygen compared to adult hemoglobin Carbon Monoxide (CO) Has a higher affinity for Hb than O2 (200x higher than O2) Therefore, even small concentrations of CO decreases O2 carrying capacity This can lead to carbon monoxide poisoning and hypoxia Symptoms: Headaches, dizziness, weakness, nausea, vomiting, chest pain, and confusion Carbon Dioxide Transport: In peripheral tissues Carbon dioxide is generated by aerobic metabolism and needs to be transported to the lungs for removal In blood stream; CO2 is transported in 3 ways: 1. Dissolved in plasma (7% limited solubility in plasma) 2. Bound to hemoglobin in RBCs (23%) Resulting compound is carbaminohemoglobin (HbCO2) 3. Converted to bicarbonate ion, HCO3 (70%) - mostly this one Conversion of CO2 to carbonic acid (H2CO3) Most carbon dioxide entering blood (70%) converts to carbonic acid Reversible reaction catalyzed by the enzyme carbonic anhydrase Carbonic acid dissociates into bicarbonate and hydrogen ions Hydrogen ion (H+) binds to Hb, forming HbH+ Hb molecules function as pH buffers Bicarbonate ions (HCO3-) exchanged (leave cell) for an extracellular chloride ion (Cl-) Process called chloride shift Haldane Effect Deoxygenation of blood increases its ability to carry CO2 Conversely, oxygenated blood has a reduced capacity to carry CO2 This helps RBC's exchange CO2 for O2 in the lungs (external respiration) and exchange O2 for CO2 in the tissues (internal respiration) Carbon Dioxide Transport in the blood: Gas Exchange at the Alveoli: Respiratory Centers and Regulation: Respiratory Center Main components of automatic respiration are located in the medulla Spontaneous rhythmic discharge of medullary neuron is modified by neurons in the pons Medullary Respiratory Center 1. Dorsal respiratory group/inspiratory center (DRG) 2. Ventral respiratory group (VRG) 3. Pre-botzinger complex Pontine Respiratory Group 1. Apenustic centers 2. Pneumotaxic centers Medullary Respiratory Center: Dorsal Respiratory Group Mainly concerned with inspiration – biggest one that matters in terms of breathing – MOST IMPORTANT Inspiratory center of Dorsal Respiratory Group controls lower motor neurons to primary inspiratory muscles (external intercostals, diaphragm) Intercostal nn to external intercostals Phrenic nn to diaphragm During normal breathing, active for 2 sec, inactive for 3 sec Not true for everyone, just general Dorsal respiratory group varies response through input from: 1. Chemoreceptors detecting O2, Co2, and pH levels in blood/CSF 2. Baroreceptors that detect pressure/stretch monitor stretch of lung wall Negative feedback system Ventral Respiratory Group (VRG) Mainly associated with forced breathing Functions only when breathing demands increase and accessory respiratory muscles are involved Activated by DRG Accessory mm of inhalation & exhalation Pre-Botzinger Complex Rhythm maker that send input to DRG Essential to all forms of breathing but poorly understood Pontine Respiratory Group: Transmit nerve impulses to the DRG Modifies the rhythm of breathing by adjusting nerve output from the medullary respiratory centers Contains two paired nuclei in the pons 1. Apeustic centers 2. Pneumotaxic centers Apneustic Centers Promote inhalation by stimulating DRG – it isnt setting the DRGs rhythm (it is adjusting the rhythm) Degree of stimulation adjusted based on sensory information from the vagus nerve about lung inflation Pneumotaxic Centers Inhibit apneustic centers Promote passive or active exhalation Increased pneumotaxic output shortens inhalation duration ( = faster respiratory rate) Decreased output slows pace and increases depth of respiration it isnt setting the DRGs rhythm (it is adjusting the rhythm) Regulation of Respiratory Centers: Input from several different locations can impact the signals coming from the respiratory centers Cerebral cortex Hypothalamus Limbic system Chemoreceptors Baroreceptors (mechanoreceptors) Proprioceptors Cerebral Cortex Voluntarily change or stop breathing To keep out gases or water But, increasing Pco2 & H+ in blood strongly stimulates DRG and sends impulses down phrenic & intercostals nerves to resume breathing If you hold your breath until you faint, you will then resume breathing Chemoreceptors Central and peripheral chemoreceptors detect chemical changes in the blood/CSF Under normal conditions, Pco2 is the most important factor influencing respiration Rise of only 10% in arterial Pco2 doubles respiratory rate Po2 levels have to drop below 60 mm Hg before triggering respiratory centers Central chemoreceptors located in the medulla oblongata of CNS (don’t need to know) Monitor Pco2 and H+ in cerebrospinal fluid Peripheral chemoreceptors are a part of PNS (don’t need to know) Monitor Pco2, Po2, & H+ in blood Aortic bodies - in wall of aortic arch close to aortic baroreceptors - part of vagus nerve (CN X) Carotid bodies - in wall of L/R common carotid arteries, close to carotid sinus baroreceptors - part of glossopharyngeal nerve (CN IX) Hypercapnia A slight increase in Pco2 (and thus H+) Most commonly caused by hypoventilation or obstructive diseases - COPD Stimulates central and peripheral chemoreceptors DRG activated, and hyperventilation occurs (rapid & deep breathing) - helps expel excess CO2 Hypocapnia A decrease in Pco2 Most commonly caused by hyperventilation (eg. anxiety) Central and peripheral chemoreceptors not stimulated No impulses sent to DRG DRG sets its own pace until CO2 accumulates and Pco2 increased to 40 mmHg Decreased arterial Pco2 Baroreceptors (mechanoreceptors) Detect lung expansion with stretch receptors (baroreceptors) in walls of bronchi & bronchioles Send inhibitory signal to DRG via vagus nerves (CN X) Activated when tidal volume >1500 ml (remember, TV = 500mL at rest) Called the Hering-Breuer reflex Proprioceptors Ventilation increases even before the need for O2 increases Proprioceptors of joints and muscles activate DRG Upper motor neurons in primary motor cortex also activates DRG Respiration in Exercise: Variations in Ventilation Pulmonary ventilation adjusts to meet body's changing oxygen needs Varies number of breaths/minute (respiratory rate) and volume moved per breath (tidal volume – VT) Respiratory rate = number of breath/minute Normal adult resting: 12 – 18 breaths/minute Average for children: 18 – 20 breaths/minute Respiratory minute volume = volume of air moved per minute Exercise Pulmonary perfusion increases as cardiac output increases O2 diffusing capacity increased 3X the rate at rest (rate that O2 diffuses from alveolar air to blood) d/t more pulmonary capillaries maximally perfused Initial abrupt breathing increase due to neural changes 1. Proprioception 2. Limbic anticipation 3. Primary motor cortex Gradual breathing increase with moderate exercise due to chemical & physical changes 1. Decreased P o2 2. Increased P co2 3. Increased temperature