Neurobiology Lecture Notes PDF
Document Details
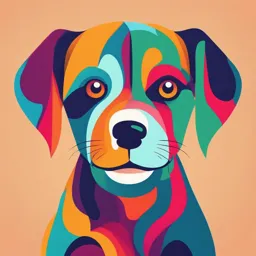
Uploaded by FruitfulSynergy8358
Tags
Summary
These notes provide an introduction to neurobiology, covering historical perspectives, different types of nerve cells and their organization, and the roles of glial cells. The text also delves into the structure and properties of cytoskeletal elements like microtubules and neurofilaments, elaborating on their functions.
Full Transcript
Introduction to Neurobiology – Lecture 1 Lesson: 28th of october Historical account - ca. 400 b. c. Aristoteles considers the heart as the organ responsible for thinking - 1504 Gregor Reisch localizes the intellectual capacities in the brain ventricles - 1543 Andreas Vesal(ius) recognizes th...
Introduction to Neurobiology – Lecture 1 Lesson: 28th of october Historical account - ca. 400 b. c. Aristoteles considers the heart as the organ responsible for thinking - 1504 Gregor Reisch localizes the intellectual capacities in the brain ventricles - 1543 Andreas Vesal(ius) recognizes the brain as the major center of mind and thinking - 1664 Thomas Willig defines the forebrain (Großhirn) as the site of memory formation - 1789 Galvani discovers the electrical nature of neuronal activity - 1891 Ramon y Cajal defines nerve cells as separate entities forming mutual contacts - 1897 Sherrington defines synapses as the sites of contact formation and communication between nerve cells - 1920 Otto Loewi identifies a chemical substance involved in signal transmission between nerve and/or muscle cells („Vagusstoff“) Levels of Neural Organization 1. Behaviour 2. Systems and pathways 3. Centers and local circuits (Circuits = Verschaltungen/ Stromkreise) 4. Neuron 5. Microciruits 6. Synapse Properties of nerve cells - Mature nerve cells are unable to divide and cannot regenerate (few exceptions) - each cell makes about 1,000 synaptic contacts, resulting in 10-100 thousand billion total contacts - 10-100 billion cells are provided at birth - natural daily loss of about 10,000 cells Properties of glial cells - mature glial cells are able to divide and can transform into tumor cells - uniform cell processes (no specialized axons or dendrites) - do not make synaptic contacts like nerve cells - usually of smaller cell size than nerve cells - four major cell types Introduction to Neurobiology – Lecture 2 Lesson: 4th of November Cytoskeletal Elements - 3 classes of cytoskeletal proteins - Present in all mammalian cells - Axonal processes can get up to one meter long - In a giraff even several meters long 1. Microtubes (blue - all over the cell) 2. Intermediate filaments (not visible in picture below) 3. Actin filaments (red – a few all over the cell (cell body, processes) but mainly at end of processes → still able to grow) Microtubules - Diameter: 20-25nm - empty in middle (hollow core), round structure - composed of globular protofilaments (units of those: heterodimer tubulins) - alpha and beta tubulins (different structures) - 13 protofilaments forming one ring/ the cylinder - Microtulules have directions in which they point; direction is defined by polarity, dynamic instability, plus end higher dynamicity, direction of transport - Microtubule associated proteins(MAPs) - ex.: kinesin, dynein, TAU-Proteins (related to Altzheimer´s Disease) - additional proteins - binding to certain parts of the filaments having certain functions: 1. helping to build fibres 2. cut fibres, provide dynamicity how fibres are build up/ destroyed 3. they have a high dynamic turnover Neurofilaments (Subtype of intermediate filaments which are present in neuronal cells) - Diameter: 10nm - different types of intermediate filaments depending on type of subunits (the basic structure of the proteins that are building up that filament) - neurofilaments in neuronal cells - solid fibers, subunits fibrous - 3 neurofilament proteins which are building up the neurofilaments - side arms Microfilaments (actin filaments) - Diameter: 5nm - Smallest of all cytoskeletal elements in diameter - Subunit: actin - composed of globular actin monomers Neurons are consuming much more energy than ANY other cell! Cellular organells - mitochondria membrane surrounded organelle long tubular structures these structures can fuse with each other containing a little bit DNA (not their function) providing energy (powerstation) evolutionary coming from bacteria (eucariotic cells) energy in the form of ATP (adenosine-tri-phosphate) (ADP+P glucose, oxygen→ ATP) energy release: ATP → ADP+P - endoplasmic reticulum structure mainly containing membranes rough ER (ribosomes): production of proteins smooth ER: production of lipids - nucleus storing most genetic material DNA (some in mitochondrium) membrenal structure surrounding nucleus transcription of genes messenger RNA (which will further be turned into proteins outside of nucleus in cytoplasma) nucleolus: ribosome assembly (important for translation) - Golgi-apparatus Contains membrane discs surrounding vesicles can fuse with membrane/ Golgi-apparatus modification of proteins from the ER (content from vesicles (mainly proteins) can undergo different post translational modification within this) sorting of vesicles secretion of proteins and mucous substances (sorting those proteins which are meant to be secreted out from the cell) Axonal Transport (2 Types) - continuous supply of substances and products from the soma, such as proteins or cell organelles, to nerve terminals - 1. Type: slow axonal transport / 1-6mm per day all proteins for nerve fiber growth/regeneration transport for bigger items like cytoskeleton proteins or small segments of microtubules - 2. Type: fast axonal transport / 100-400mm per day Anterograde: towards the nerve terminal (kinesin) – mitochondria, vesicles Retrograde: towards the soma for recycling processes of used proteins (dynein) – recycled proteins (need to be pulled back from axonal processes to be recycled) - Transport via Microtubules (kinda the roads oft he nervous system) - motor proteins (proteins which are able to exert movements) transporting goods (kinesin & dynein) kinesin always travels to plus-end of microtubules (anterograde transport) dynein travels to minus-end (retrograde transport) Molecular Structure of Kinesin - 2 Subunits = dimer - motor domain & stalk (2 domains within one subunit) stalk: responsible to connect to cargo/vesicle/mitochondria (stalk is holding the cargo – Ladung, Frachtgut) motor domain: part which can excert movement (with ATP → ADP+P process called hydrolysis) → turns energy into movement: ATP is hydrolized and a phosphate group is released Like this energy is produced which can now be used for movement (motor domain makes hydrolysis itself →this is its enzymatic function) → energy is used to change structure of one motor domain, pull it up and place infront of other (can be imagined like walking, putting oe „foot“ in front of the other) → each ATP → ADP+P provides energy for 8nm step (hand over hand walking model) Introduction to Neurobiology – week 3 Lesson: 11th of November 2022 Ultrastructure The architecture of cells and biomaterials, including the cell organs Isolating biomembranes from red blood cells - Red blood cells: final differenciation level of a certain cell type - Rbc have a short live spann, therefore have to be produced fast - most organells missing, no nucleus - Ideal cell type because these cells mostly consist out of membrane (and cytoplasm, cytosceleton) - 1. Add water (H2O), cells breaks (saugt sich voll, Membran reißt) 2. Membrane and internal cell stuff differ 3. Membrane is heavier, therefore the blood-water mix is put into the centrifuge so that the membranes set on the ground , 4. Wash 5. Finish - Membranes consist of lipid and proteins – red blood cells: 50% Lipid, 50% proteins - Inner mitochondrai membrane about 70% proteins – membrane holds a huge amount of proteins, which are nessessary for creating ATP Therefore mitrchochria have a huge inner life of folded membranes - Myelin sheath about 70% Lipid - Task of membranes: Separating and isolating Lipids of biomembranes - Isolation! - Lipids without direct contact between the solutions - Main lipid of plasma membrane is phospholipids – lecithin - hydrophilic head: choline, ethanolamine.... phosphate glycerol - hydrophobic tail: fatty acids water-attracting water-repelling Membrane Models - hydrophilic ends (head) touch water solutions - two layers with heads on the outside if there are water solutions on both sides, oft he membrane - barrier against hydrophilic molecules – seperating both water solutions! The neuronal plasma membrane acts as a diffusion barrier against hydrophilic molecules such as ions, has a selective permeability - phospholipids/amphiphilic hydrophilic headgroup (glycerol, phosphate group) hydrophobic tail (fatty acids) - proteins/hydrophilic primary structure: sequence of 20 different amino acids secondary structure tertiary structure Protein Structures Those structures can mix within a single protein: maybe starting with a random coil, then going into an alpha helix, then making a random coil again,… - primary structure: amino acid structure, backbone of peptide bond many different kinds of amino acids in one protein mix of positive and negative loads, some are repelling/ making contact if they come too close to each other - secondary structure: some amino acids which are further from each other than in the primary model three structures of 3D polypeptide chain: Alpha helix Beta sheet Random coil - The conformation depends on the amino acid loads and their composition - thats how these protein structures form - Conformations of proteins can change: That is how proteins provide funtions (E.g. as opening an ion channel) Levels of protein structure A) Primary structure: molekulare Darstellung B) Secondary structure: written above shapes C) Tertiary structure: Overstructure of one polypeptide, say one alpha helix that is twisting or coiling around itself Heme-Grups may add to these compositions, but they dont belong to this certain polypeptide D) Quartery structure: More than one polypeptide together to form one final functional protein Conformational changes of a protein The conformation of a protein can change in a precisely defined manner allowing to perform a specific task - A signaling protein binds on the conformation-changing protein E.g. Calcium binds on Calomodulin, which changes its conformation as a result - Protein changes conformation, adapts to a different conformational state to provide another function Structural Characterization of Proteins important molecular tools of nerve cells to control excitability, electrical activity and chemical signalin - molecular biology (cDNA-analysis) amino acid sequence determination (primary structure) - secondary structure prediction/determination spectroscopy Have information of primary structure while understanding DNS-Coding - tertiary structure determination crystallization and x-ray analysis Enzymes - Proteins that control biochemical reactions in cells - Recognizable by name from the suffix -ase - Enzymes are substrate-specific - Catalyzing specific reactions substrates enzymes Phospholipid phospholipase Protein Prtoease ATP ATPase - Enzyme and substrates work with the key-lock principle * * - Enzyme speciffically binding to the substrate pocket - Many proteins change their conformation after the enzymes binded onto them and did their job so the protein is able to escape the key-lock - Enzymes have a certain life span which by certain tricks can be extended. They dont get destroyed after they executed their function once - Phospholipid is a group of lipids, not a singular one Membrane proteins - Can proteins exist inside of membranes? - Amin acids can be hydrophilic, hydrophobic, basic, neutral, acidic, therefore have a huge variety -> - Hydrophobicity index: A way to measure the amount and relation of hydrophilicy and hydrophobicity inside a protein - A protein consisting out of a hydrophilic and a hydrophobic part can be a part of the membrane as an integral membrane protein - A protein consisting out of a hydrophilic, a hydrophobic and another hydrophilic part can span a membrane (e.g. a protein channel) Introduction to Neurobiology – week 4 Lesson: 18th of November 2022 The resting menbrane potential: who are his players and how is it formed? Molecules not able to cross the plasma membrane Cations Anions (positively charged molecules) (negatively charged molecules) - sodium Na⁺ - chloride Cl⁻ - potassium K⁺ - phosphate PO4³⁻ - calcium Ca²⁺ The charge of the molecule attracts water molecule to surround the Ion. This is called hydration; a water shell. Therefore they cannot pass through the hydrophobic region oft he plasma membrane. That the ions cannot move freely is the basis of the bioelectricity: Bioelectricity - ions cannot freely move across plasma membranes - membrane potentials are generated by an asymmetric distribution of anions and cations on both sides of the membrane - membrane can function as capacitor if membrane surface outside and inside cell is differently charged (stores energy on the two sides of plasma membrane → membrane potential) capacitor: passive two terminal electrical component that stores potential energy in an electric field - How can this potential be measured? Researchers in the early 19hunderts used a sqid, which hast he animal with the thickest axons (up to 1mm). Following concentrations could be read from the sqid axon: within neuronal cell we have special mechanism which needs to have very specific permability to certain ions resulting in completely different concentration than blood/ sea water Calcium very low; much more important for other cellular functions even if small amounts of calcium would go across membrane, it could induce several single transduction mechanisms Potassium and Sodium competely different distribution Structure of the Na -K -ATPase The obviously contrary charge of sodium (Na+) and potassium (K+) is artificially created by a certain enzyme, the ATP-ase. Due to ist function it is also called the Sodium-Potassium-pump. To shuffle the ions against their concentration gradient, it needs some sort of energy source. The ATPase is able to break ATP into ADP and a phosphate group, which creates enegry that can now be used to transport the sodium and potassium across the membrane against their concentration gradient. The high need for energy created by the sodium-potassium-pump causes an insanely high ATP/energy consumption oft he neuronal cell in comparison to other cell types. The anatomy oft he ATPase consists mainly out of alpha-helixes and beta-shields. To pass the membrane in ordert o execute its function, the ATPase needs to be embedded in the plasma membrane and therefore has transmembrane domains. Speaking in plural because the enzyme has to be able to form a channel within the membrane to move the ions. These transmembrane domains are 10 alpha-helices which are spanning the membrane to form this tunnel. The energy-producing part which breakst he ATP lies in the intracellular part because the ATP is present intercellularly, since it is mainly made by the mitrochondria. A single breaking of ATP can transport three sodium ions out and two potassium-ions in. This makes this enzyme outstanding in energy efficiency. Step by step: the functional process of the ATPase 1. In the intracellular surface ATPase is binding ATP and the sodium ions ATPase breaks ATP into ADP and a phosphate group The phosphate group now does not disappear somewhere in the intercellullar room but instead binds an aminoacid of ATPase and phosphorylates the enzyme 2. ADP is released which leads to a conformation change oft he enzyme: The sodum ions willl be occluded within the proteine 3. The second conformational change is initiated and the sodium is released into the extracellular room 4. This new conformation allows potassium ions from the extracellular space to bind onto the proteine 5. This potassium uptake initiates that the phosphor group is released (dephosphorylation). Hereby the potassium ions are occluded within the ATPase. 6. A new ATP comes to bind onto the enzyme which leads tot he final conformational change and a release oft he potassium into the intercellular room. 7. The circle is now starting over again. Resting membrane potential - Higher concentration of Potassium Ions inside makes them escape to outside, even though overall potential might be neutral; this leads to an increase in positive potential outside, negative inside - leads to membrane potential (resting membrane potential) - average neuron usually at -71,2 mV Excitability of Nerve Cells - Na+ /K+ -ATPase makes electrochemical gradient for Na+ and K+ - potassium leakage via ion channel because potassium level is increased due to Na+ /K+ - ATPase leads to rather negative inner membrane surface and rather positive outer membrane surface - leakage funtion process of the ion channel: Ion channel embedded in membrane More potassium inside than outside of the neuronal cell If one of the ion channels is in an open state, the potassium will simply flow out because oft he concentration gradient (Brown´sche Molekularbewegung) K+ concentration gradient drives K+ out of cell at the same time electical potential difference drives K+ into cell, because the more potassium leaves the cell, the more negative the gradient becomes – driving potassium back in Negative charged molecules are not able to follow the potassium, because they cant pass the membrane or travel through the channel (selective permeability) no additional energy source, gradient is enough Sodium leakage current smaller than K+ channels letting Na+ into cell because of negative charge inside cell caused by extracellular K+ leakage also less sodium ion channels neertheless sodium ions are also relevant for building up the resting membrane potential electrochemical balance but electrical potential difference Calculation oft he membrane potential calculation of membrane potential in voltage: Numbers from the squid table: Nernst equation The Goldman-Hodgkin-Katz equation (modified version) Summary of Information - Basis how neuronal cells are working are differences in charges of both sides of the cell (asymmetric distribution of positive and negative charges) - resting membrane potential generated - potential changes when cell is functioning in gradient fashion or in an all- or non fashion (actionpotentials) - Phospholipid bilayer working as isolation / Ion regulation system - Ions always covered in water shell (hydrated coverage) → can‘t pass through membrane due to hydrophobic nature of membrane - Proteins regulating distribution like Sodium-Potassium ATPase (working constantly) - highly energy consuming ATPasemaking own energy by breaking down ATP - Leakage (Ion) channels, most times specific to certain Ion - They usually open according to signal ex: Ion concentration or gradient - electrical gradient pulling Potassium into the cell and chemical gradient pulling it outside - Equilibrium via electro-chemical gradient reached if resting membrane potential is achieved - Also Ion channels for sodium and chloride - rather negative membrane potential inside cell and positive outside Introduction to Neurobiology – week 5 Lesson: 25th of November 2022 Experimental Manipulation of Membrane Potential Injection of different ions into the axonal process More negative: Membrane potential changes into more negative state (down to -90mv) Membrane potential changing into negative: „Hyperpolarization“ More positive: membrane potential changes into more positiove values, reducing of polarity „Depolarization“ Action potential First researches by electrophysiological recordings. If cell stays too long in a positive value, the cell will lose the ability to communicate and die; it has to return to the resting membrane potential before too long. Experimental procedure: Small, sharp stimulus lasting 2 miliseconds -> leads to hyperpolarization of the resting membrane potential, RMP builts fastly back Next experimental procedure: Change towards depolarization, RMP again builts fastly back Small stimulus -> small response, bigger stimulus -> bigger response Once the stimulus is high enough to bring the RMP up to -40 mV 40mV ist he threshhold (Schwellenwert) – if this is overstepped, the answer is enormeous! After more than 40mV an overshoot takes place and the action potential happens. From this point, the strentgh oft he stimulus doesnt matter anymore, no matter how strong it is, once the threshhold has been overstepped, the actionpotential always happens with the same strength (amplitude). This action potential can now be sent all over the axonal process without fading and losing its strength – it does continue being produced all over the axon no matter the length of the processes. Frequency modulation Since the action potentials amplitude never changes, there is another way of communicating the strength oft he incoming stimulus. This can now be accorded in the emodulation of the frequency of following action potentials. Providing information for the adressed neurons: „This stimulus was insanely strong/ quite weak“ The refractory period The regeneration process after the action potential After the depolarization and the weakening of it and its return tot he RMP a hyperpolarization follows which leads to struggles with newly arriving stimuli – during the refractory period, an action potential cannot be initiated 78 The sodium hypothesis Alan Hodgkin and Andrew Huxley worked with squid Axons. Hypothesis: electrical stimulation causes a sudden increase of the membrane‘s sodium permeability. This would mean that many ion channels open due to electrical stimulation, which results in a fast sodium influx that depolarises the membrane → depolarisation of membrane. In their experiment: reduced external sodium concentration (exchange solution which axonal process was bathing in). Result: axonal process decreased in size. The voltage-clamp technique - measuring ion currents through membranes of exitable cells - while holding certain membrane voltage at set level (clamping voltage at certain level) - modifying voltage (via command voltage), then understand whats the required compensatory current for cell to reach voltage which had been set (via feedback amplifier and current passing amplifier) - measure voltage of plasma membrane to see changes (voltage Measuring Amplifier) to go to level we were setting at command voltage Channels Sodium inwart current (current – Einstrom) is stopped at a certain porint – if it went further on, the whole information transpassing process would take very long. SO there hast o be a mechanism that closes the sodium channels Different kinds of ion channels: - Voltage-gated: Once the electrical mambrane voltage changes, they open and close when it changes to a certain level - Leaky channels (always open) - Molecule-binding ion channels (that open or closes when a molecule binds) Pharmacology of ionic currents Introduction to Neurobiology – week 6 Lesson: Second of December 2022 Sodium: English for Natrium (exactly the same element) Potassium: English for Kalium (again exactly the same element) Voltage gated channels need something to sense the voltage in ordert o be able to open and close the channels once the voltage reaches the threshhold – an enzyme? Isolation oft he sodium channel protein - Model for that: Electric ale (Aal) - Using the gel-electrophoresis to understand the ales proteins - Gel-electrophoresis is sorting the proteins by their size - Loading samples into „pockets“ that are filled with a gel - This gel has a certain poor-size - Upper-and lower-bufferreservoiar - Upper: negatively chargen, lower: positively charged - Applying electrical force, this leads to a travel oft he proteins - The small proteins migrate faster, the big ones slower - This seperates the proteines very well - Proteines are being stained so that they are visible - In the gel a certain proteine cannot be targeted, but on the surface of a membrane a certain protein can be targeted and stained – nowadays with the use of antibodies - This antobody for sodium is saxitoxin: Consists of beta1 and beta2 subunits (each 30 kilodalton) as well as alpha subunit (260 kilodalton – more than 2000 aminoacids) Voltage gated Ion-channels - hydrophobicity plot (if we know amino acid sequence of a protein) - if enough hydrophobic amino acids → able to span membrane - between transmembrane elements there can be a hairpin loop (selectivity filter) structure hairpin loop: providing selectivity for respective ion (sodium ion) between S5 and S6 (channel pore) Heterologous expression systems - frog oocytes were used becase the are pretty big - nowadays an injection is not required anymore so the frog is usually not neccessary anymore - after isolation and cloning of ion channels: invitro transcription - varify that electrophysiological properties are same as electrophysiological properties of native sodium channels - invitro transcription: cDNA (cellular DNA) converted into mRNA invitro (in chemical tube) - invitro transcribed mRNA can be modified with tools (check for changes in electrophysiological properties of protein) - mRNA injected into unfertalized eggs of frogs (Frog Oocytes of african claw frog cause they‘re big) - mRNA translated and voltage gated sodium channels placed into plasma membrane of Frog Oocyte Two-microelectrical voltage clamp Inserting microelectrodes into plasma membrane Setting certain voltage, reading out current that is needed to compensate the set voltage Recognizing „whole cell current“ (all oft he ion channels in the plasma membrane indtead of individual ion channels) Voltage-dependent gating of ion channels - S4-segmants are positively charged - Once the positively charged inner surface is appearing, the segments rise up and ions can flow through - Positive repells positive - These channels have to be able to inactivate their gates - This is done with the effect of pronase - using digestion via proteases (enzymes which are cleaving (pronase in this case)) proteases: digesting proteins cause they‘re cleaving peptide bonds between amino acids pronase (partial cleaving) changes sodium current very fast → prolonged opening of sodium ion channels - inactivation particle (gate) situated in intracellular part between 3rd and 4th domain of protein at high depolarised state of plasma membrane, particle changes position and blocks channel - Accessory Beta-Subunit (2x30kilodalton)!! beta 1 binding to 4th domain of alpha-subunit both changing kinetics of ion channels not required for basic function Voltage sensor Sodium Functionl elements: - Voltage sensor – S4 - Selectivity filter – hairpin loop between S5 and S6 (channel pore) - Inactivation gate - intracellular loop between S3 and S4 Sum Up on sodium channels Three states of soduim channels - Closed when resting membrane potential (negative inner membrane surface) - Opened when depolarization is reaching the threshhold - Inactivated when depolarization is even higher but no more sodium is supposed to cross therefore inactivation particles change position Beta-subunits - Beta1 binding to 4th domain of alpha subunit - Beta2 binding to 1st domain - Changing kinetics of ion channel – beta nott neccesarily required fort he function but definetely engaging and supporting it Voltage gated potassium channels - Model organism: fruit fly (good isolation of genes and proteins responsible for a change in behaviour) -> positional cloning Flys shaking when undergoing anasthesis (shaker mutants) -> pointing towards neurological problem Check up with neurobiologists Shaker mutant: elongation of action potential because potasium ions much slower going out of cell Potassium current reduction Voltage clamp experiments Problem in voltage gated potassium channel gene - Structural characterization oft he potassium channel: 6 transmembrane segments 4th segment: enriched in positively charged aminoacids; serving as a sensor for changing in the voltage Hairpin loop in between 5th and 6th segment providing selectivity filter Comprising just one domain; much smaller proteine than for sodium channel. How can this proteine possibly form a pore? - Electronmicroscopical 3D-reconstruction - For this researchers needed purified channels - cDNA expressed in a certain cell model channels are forming functional structure in the membrane, from there these proteins are purified Once a channel proteine is purified and placed on a surface to make highresolution electromecroscopical images a lot of information can be drawn from there images Channels are not comprising of one domain but from four proteines which are forming a tetramer Why four proteines? - Possibility to exchange subunits - Means that four genes have to be encoded and expressed - Nine different types of sodium channels in different cells in the mammalian system - Even higher variation possible due to differentiating combination of subunits > increased variation of channels due to different combinations - hydrophobic aminoacids within the inner pore – charged ions have to lose the hydration that they carry for energetic stability if they want to cross - on the two ends hydrophilic aminoacids close to the surface Potassium channel selectivity - ions are of different size e.g. potassium is bigger than a sodium ion can a sodium ion pass through a potassium channel? Size is not the only relevant trait a molecule can have - channels have inner and outer vestibute cell inside and outside are hydrophilic aminoacids there ions are in hydrated state ion needs to lose water molecules when reaching selectivity filters – but for stability of it´s energetic state the molecule has to connect to something, so when losiung the hydration, it instead connects to oxygen atoms which are standing alongside the selecticity filter on the other side (after passing) the ion loses the oxygen and again connects to water molecules the sodium ion may have the right size to cross the channel but smaller charged ions have a bigger hydrated surface around them – aequathic coverage is larger fort he sodium than for a larger ion sodium can bin to one oft he oxygen but not on the other side (oxygen needs tob e added on two sides) but it ist o small and therefore cannot lose all ist hydration – because it is to small and cannot lose al ist water molecules, it cannot reach through the hydrophobic inner part oft he potassium channels Types of voltage-gated potassium channels - Shaker (Kv 1.1 – Kv 1.3) (conductivity at: -40mV) - Shab (Kv 2.1 – Kv 2.3) (conductivity at: -40mV) - Shaw (Kv 3.1 – Kv 3.3) (conductivity at: -20mV) - Shal (Kv 4.1 – Kv 4.3) (conductivity at: -60mV) (acting completely different to others) Modifications oft he patch-clamp technique - Invented to analyze the function mechanism and activity of individual, single ion channels - Not usig a sharp microelectrode to penetrate into the membrane but an smooth one that is placed gently onto the surface to seal potentially just one ion channel and record the current which is coming through that explicit ion channel - Pulling small surface of cell membrane and measuring on that - Several modifications: Stronger suction whith opening the membrane: complete crossover between micro- pipette and inner part oft he cell (measuring the whole cell mode) Single recordings: pulling small surface and hoping to contain just a single ion channel Inside out: Inside of cell is outside (from on-cell) Right-side out: right-side is outside (from whole-cell) punctuating the cell in advance, then pinching off a small part (membrane will close again due to phosphor-lipids) Voltage-gated calcium channels - Present at presynapse (axon terminal), very important function - Sensing voltages - Structure: resemble mainly to sodium- channels - Calcium levels extra- and intracellular are very low - Initiating many different signalization reactions - Toxic in too hiugh concentration (initiating toxic reactions) - Long polypeptide chain Alpha-subunit Respective four domains Voltage sensing transmembrane segment Many more accessory subunits: alpha2, beta, delta, gamma subunits Introduction to Neurobiology – week 7 Lesson: 9th of December 2022 Cable properties Researchers conducted several experiments to find out about cable properties - Inserted microelectrode into axonal process Initiating positive current, leading to deporalization voltages Clamping Measurements of voltage on both side oft he electrode alongside axonal process Membrane depolarization is not happening locally but affects both sides oft he electrode But weakening out the further the distance tot he microelectrode Membrane resistence (Rm) – how much membrane is resisting to leak ions out (how many leaky channels are in the membrane?) High mmbrane resistence means many leaky channels, low membrane resistence means low amount of leaky channels Length (internal) resistance (Ri) – how much ions can spread alongside inner axonal membrane - λ = length constant (distance over which magnitude of depolarisation falls to volaue of 1/e of initial depolarisation) - a = axon diameter, bigger diameter, bigger membrane surface, more ion channels to leak ions smaller Rm and Ri Ri decreasing faster than Rm → longer λ value - there needs to be a mechanism within axon to constantly reinforce depolarisation for long distance travelling (signal propagation) - This signal is weakinging along the process and therefore lost if it is not being reinforced Action potential Signal propagation - passive electrotonical spread → continuous impulse conduction - action potential only propagates towards axon terminal from cell body sodium channels afterwards are in closed state so can be opened channels before are still in inactivated state (refractory period) - Impulse conduction (Velocity): more complex tasks requires more neurons and higher speed myelin sheath: isolation around axon in periodic manner axon potentials only generated between myelin sheaths (nodes of Ranvier) called saltatory impulse conduction diameter makes difference in velocity for unmyelinated fibers! (WHY?) Impulse conduction systems - Continuous impulse conduction Traveling continuously across the axon The thicker the axon the faster the impulse speed - Saltatory input conduction faster conduction due to myelin sheath Periodic isolation around the axon increase of membrane restistance reduces leakage currents lower leakage → faster, longer enhances conduction of action potentials even in thinner nerve fibers/ axonal processes reduction of capacitance (C = F/d) accelerates passive electrotonical spread focused localisation of ion transport processes at nodes of Ranvier saves energy → process much faster action potential jumping from node of ranvier to node of ranvier (ranvier´sche Schnürringe) Nodes of Ranvier - double labeling immunoflourescence - not all oft he humane processes are saltatoric, many are but not all need the speed - every myelin sheath consists out of just one glial cell membrane sheath Anchoring of sodium channels (why channels clustered) - anchoring oft he sodium channels by proteins (ankyrin G) which themselves are connected to cytoskeletal elements of the cell via spectrin (actin filaments) Cells forming myelin sheath - Glial Cell Types: Oligodendrocyte (~25%) and Schwann Cell - rougly equal proportions of glial cells and neurons in body - Schwann Cells present in peripheral nervous system one Schwann Cell is myelinating/ insulating one segment of an axonal process - Oligodendrocytes present in central nervous system one oligodendrocyte is able to insulate several segments of axonal processes - myelin sheath: many layers of plasma membranes - major dense line: inner membrane surfaces of Schwann cell - intraperiod line: outer surfaces usually facing surrounding of cell Myelin proteins (sticking together the wrapped membranes and providing isolation) - CNS: Proteolipidprotein (PLP) and myelin basic protein (MBP) - PNS: myelin basic protein (MBP) and Po Protein - intraperiod line: PLP and Po - major dense line: MBP - Function of myelin basic protein: without MBP, layers not tight together → uncoordinated movement (Shiverer) Multiple Sclerosis – Cell Pathologie - Autoimmune disease - Immunesystem produces Auto-antibodies against myelin proteins (MBP) - Patients might have had Measle virus infection before molecular mimikry: molecule belonging to virus similar to MBP, misjudged structure antibodies need to go through blood brain barrier - Therapy: Immunesuppession, Trials for cell transplantation (not successful yet) - Diagnosis: NMR images of plaques, angiocentric demyelination Glial Cell Types of the CNS Astrocytes - connect to neurons and can provide metabolic support of neuronal cells - connect to nodes of ranvier - connect to blood vessels and take nutrients out of them - wrap around synapses and influence the processes there Microglial cells - smallest type of glial cells - function: reacting to neuronal damage (clearing out damaged cells) in central nervous system (CNS), kinda immune system oft he cns - neuronal damage → cell loses integrity → leaking molecules like ATP (signaling molecule) - microglial cells have ATP-receptors → activated microglial cell undergoes proliferation (increase in number) - synthesise and secrete cytokine (signaling molecules of immune system) to damaged cell - depending on type and amount of cytokine and state of neuronal cell it either provides neuroprotection or is cytotoxic (kills cell) - also removal of damaged cells - partially responsible for scar formation Summary of Functions of glial cells Ependymal cells - CNS - Glial cell - Cannot migrate - No processes - Function: isolating surfaces - Cubiodal shape - On the side of the ependyma that is exposed to the CSF, ependymal cells have several motile, hair-like projections called cilia. Function: to move the CSF through the ventricles of the brain and the central column of the spinal cord. - Ependymal cells also have a number of microvilli. Function: these are non-motile structures that increase the surface area of the cell and assist with the reabsorption of CSF. - Responsoible fort he production oft he ceretospinal fluid - Main function of ependymal cells: to produce the CSF that fills the cavities of the brain and spinal cord. Introduction to Neurobiology – week 8 Lesson: 16th of December 2022 Synapse between motor neuron and muscle fiber (neuromuscular junction) - When implementing a microelectrode into the muscle fiber, after a stimulus a potential can be measured - This potential looks very similar to the classic action potential but not exactly the same -> it’s a muscle action potential - Muscle reacts with contraction - Contraction in different degrees/ strengths depending on the frequency of the stimulus - Axon terminal of the motorneuron is called the motor end plate (basically the presynapse) - When measuring the action potential closer to the motor end plate, it looks different - Curare: Poison used by south American tribes to paralyze the prey - When injected, even after stimulus, no action potential can be measured, therefore no contraction - When measuring very close to entplate: Very small response could be measured -> the endplate potential The endplate potential and the muscle potential work differently At neuromuscular junction occurs a membrane potential change that involves a mechanism, which provides a graded change in the membrane potential If membrane potential is strong enough, then sodium channels open and AP is conducted Ultrastructure of neuromuscular junction - neuronal endterminal full of membrane coated vesicles (neurotransmitter vesicles) - membrane of muscle fibre folded to hold a lot of receptive molecules able to bind specific neurotransmitters Neuromuscular junction (NMJ) - Neurotransmitter membrane surrounded synaptic vesicles merge with plasma membrane and release transmitter into synaptic cleft - On post-synapse: all receptors able to bind specific neurotransmitters - endplate = pre-synaptic terminal - Receptor: Acetylcholine-receptor AChR (Ion Channel at the same time) - Transmitter Acetylcholine cleaved within synaptic cleft - Acetylcholienesterase in Synaptic cleft to stop reaction/ enzyme cleaves leftover Ach NTM in cleft to stop the further opening of channels Synaptic transmission - neurotransmitter vesicles release content when calcium enters into pre-synapse through voltage gated calcium-channels (situated in membrane of nerve terminal) - → action potential travels through axonal process → reaches nerve terminal → initiates membrane potential change → depolarization leads to an opening of calcium channels → higher calcium ion concentration outside, Calcium enters terminal (follow their concentration gradient) → Calcium initiates fusion of synaptic vesicles with pre-synaptic membrane → realease of neurotransmitte content which cross the synaptic cleft → 2 Neurotransmitters bind to 1 acetylcholine-receptor to open receptor (ligand-gated ion channel – chemically gated) → cations (mainly sodium) will enter into and depolarize post-synaptic target cell through ion channel → change in membrane potential of muscle fibre (depolarisation representing size of neurotransmitter release: The more NTM released, the more channels open, the higher deporalization) - Enzyme protein Acetylcholinesterase cleaving acetylcholine so molecule cannot bind to receptors any longer → no further signalisation - Exocytosis: NTM release at presynaptic nerve terminal (Fusion of the vesicles with the membrane and efflux of NTM in cleft) Regulation of Exocytosis I - synaptic vesicles are connected to cytoskeletal elements: microfilaments Microfilaments are Actinfilaments - synonymes - microfilaments densely present in nerve-terminal responsible to hold neurotransmitter- packed synaptic vesicles in proper position in terminal synapsin protein binds vesicles to microfilaments Calcium enters nerve terminal, initiates change in synapsin protein, which now releases the vesicle - synaptic vesicles need to attach to pre- synaptic membrane synaptobrevin protein attached to vesicle draws to syntaxin protein in pre-synaptic membrane and they connect – vesicle is not attatched to memrane - Synaptotagmin protein fuses the vesicle with the pre-synaptic membrane Exocytosis II Docking of Vesicle membrane and Plasma membrane: Proteins of the snare family and their structures - v-snares (vesicle snares): synaptobrevin alpha-helix passes through vesicle-membrane bulky-globular unit with many secondary-beta-sheet structures beta-sheets structure with units able to form specific connections to eg Syntaxin synaptotagmin long alpha-helical structure coiling together with syntaxin, forming “coiled coil” syntaxin and synaptotagmin are pulling the vesicle and the membrane together - t-snairs (terminal snares): syntaxin long alpha-helical structure - Fusion requiring presence of increased Calcium concentration! - Protein believed to sense increased Calcium is synaptotagmin - Effect of tetanus toxin: violent spastic paralysis it cleaves synaptobrevin → blocking neurotransmitters within inhibitory interneurons (can‘t properly regulate function of motor neurons) → inhibit motor neurons which initiate contraction of muscle -> no proper regulation of motorneurons, leads to spasts Pharmacology/ Subtypes of the AChR An antagonist is a type of molecule that is blocking the physiological reaction induced by another molecule (even if the actual NTM is present) Agonist – drugs that are mimicking a molecule to induce the same physiological response - AChR (ion channels) present in skeletal muscle and heart muscle (found by researches by using different drugs) - antagonist (curare/ atropine) → drugs which block physiological response of receptor curare in skeletal muscle atropine in heart muscle - agonist (nicotine/ muscarine) → drugs which mimic physiological response (bind to receptor and initiate same conformational change of the receptive molecule that is initiating the signal transduction) nicotine in skeletal muscle muscarine in heart muscle - receptor subtypes in skeletal muscle cell membranes they‘re called nicotinic AChR (chemically gated cation channels) in heart muscle cell membranes they‘re called muscarinic AChR (G-protein coupled receptors) Isolation of the nAChR protein (nicotinic type) - source: electric organ of electric ray - drug used to identify receptors: alpha-Bungarotoxin (from taiwaneese cobra) - seperating proteins on gel and specifically labelled (with alpha-Bungarotoxin) - recognise 4 different sub-units (biochemical analysis revealed Pentamer) 2 alpha units, 1 beta, 1 gamma, 1 delta Structure of nAChR - within alpha-subunit: M1-segment has extracellular bulky part with tyrosine amino acid at specific position which is responsible to bind Acetylcholine - 2 Acetylcholine each binds to M1 segment of either alpha and gamma subunit or alpha and delta subunit - M2 is important to build the round shape of the tunnel – facing the inner pore, forming 3-dimensional structure Gating – opening AChR - Ach binds to alpha-subunits and causes a twisting movement within M2 segments which opens the ionic pathway through membrane Structure of ATP and GTP ATP - 3 main structures - nitrogenous base (Adenosine) - sugar Ribose (providing energy) - 3 phosphate groups connected to sugar Ribose GTP - 3 main structures - nitrogenous base (Guanosine) - sugar Ribose - 3 phosphate groups connected to sugar Ribose - cutting off 1 phosphate group: GDP - not providing energy like ATP - changing size of melucule - losing some of negative charge (phosphate is negatively charged) → might initiate conformational change Muscarinic type of Ach-Receptor (in heart muscle) - poisonous part of Muscarin from Fliegenpilz - action on heart muscle cells - only 1 subunit → no pore, no ionic transfer - subunit has 7 transmembrane segments - G-protein coupled receptor on intracellular membrane surface it‘s binding to G-protein complex (3 sub-units) alpha & gamma subunit: losely associating to inner membrane surface dissociation during signal transduction: into an alpha-subunit and a beta-gamma-subunit both of these dissociated sub-units able to lateraly move across inner surface of membrane to find other targets to initiate signal transduction mechanisms - G-protein complex binds GDP (guanosine-diphosphate) GDP signaling molecule when 3 sub-units together, alpha-subunit binds GDP - Neurotransmitter ACh binds to receptor through signal of GDP Signal Transduction I - on extracellular surface, we have the ligand ACh - 1 ACh bound to receptor to initiate conformational changes - conformational change in receptor leads to conformational change in intracellular G- protein-complex - alpha-subunit releases GDP and instead binds GDP → further initiates dissociation of the complex - New signaling molecule: beta-gamma-subunit reaches K (Potassium) nearby Channel ⁺ binds to channel initiates its opening Potassium outflow because there‘s higher K concentration inside cell ⁺ losing more positive charge from inside cell → even more negative inner membrane- surface (hyperpolarisation) creating ipsp: inhibitory post-synaptic potential epsp: excitatory post-synaptic potential Introduction to Neurobiology – week 9 Lesson: 23rd of December 2022 Neurotransmitters - Several families of NTMs exist, as being introduced in the following Amino acid NTM Family Amino acids usually are the building bricks for molecules and proteins, but they might also work in the function of communication as NTM (only some AA) - Glutamate: has two kinds of receptors o Ionotropic receptors (receptor molecule itself is an ion channel → opens when binding glutamate) Receptor activated via: NMDA-receptors with agonist: N-methyl-D-Asparate non-NMDA-receptors these channels also have hairpin-loop structures o Metabotropic receptors (G-protein coupled receptors) Receptor activated via: mGluR1 /-R2….R8 most (but not all) are functioning inhibitory - γ-amino butyric acid ((GABA) only amino acid functioning as transmitter but not as part of a protein) Again two kinds of receptors: o Ionotropic receptors Receptor activated via: GABAA -Receptors Chloride-channel (letting through negatively charged ion Chloride) → inducing inhibitory post-synaptic potential o Metabotropic receptors Receptor activated via: GABAB -Receptors Gβγ-activates K -channels ⁺ K flowing out of the cell o Neurotransmitter only inducing ipsp - glycine (functioning as an inhibitory neurotransmitter → ipsp) o Ionotropic receptors resembling (ähneln) GABAA-receptors → chloride channels Function of Ionotropic Glutamate Receptos - Differences between NMDA-Type and non-NMDA-Type: - non-NMDA-receptors bind Glutamate → conformational change and pore opens → allowing positively charged ions in and out (more Sodium goes in than Potassium going out) o is therefore not selective and not one-directional → Depolarisation o activated by neurotransmitter o because of strong sodium influx - NMDA-receptors bind Glutamate o Mg² hanging in middle of channel not letting through ions Even if glutamate binds to receptor This is the one strong difference between (Non-)NMDA o Depolarisation of membrane causes release of Mg²⁺ → pore opens → Sodium, Potassium AND Ca² can pass through! o voltage gated + chemically gated ion channel - NMDA-receptors: no EPSP → silent synapse - non-NMDA-receptors: EPSP If there were only NMDA receptors, they wouldn´t open simply because of glutamate since they need the depolarisation as well Therefore non-NMDA is necessary as well Properties of GABA-receptors GABA only functions as NTM, no protein building block GABA is exclusively inhibitory - Ionotropic: GABAA receptors o Chloride channel → Cl is negative -> hyperpolarisation → IPSP o Molecular Structure: Pentamer (5 Segments) each with 4TM subunits o M2 (2nd TM) facing inner side of pore allowing ions through channel o extracellular part of one segment responsible for binding one GABA o Pharmacology antagonist: bicuculline (plant toxin) binds to same position as GABA -> fighting for binding pocket if higher concentration than GABA → blocking function of ion channel → inducing severe convulsion agonist: muscimol binds to receptor and activating it Multiple allosteric modulators binding somewhere else to the channel other than the binding pocket → modulates its function Mix of antagonist and allosteric modulator: picrotoxin binds within ion channel → channel blocker Mix of agonist and allosteric modulator (allosteric agonist): benzodiazepine („valium“): binds somewhere to channel, enhancing its function Barbiturates: anesthetic drug -> enhancing effect of GABA (inhibition) - Metabotonic: GABAB receptors o Have to have 7TM Helices (general rule) o receptor hetero-dimer (2 Proteins: GABA B1 /B2) o extracellular domain also called „venus flytrap“ domain (open and closed conformation) o closed conformation is stabilised by binding of GABA o active form of receptor → activation causes activation of G-Protein (alpha- subunit dissociates from beta- and gamma-subunit) o The beta-gamma-subunit activates a potassium channel → potassium rushing out of cell -> IPSP Biogenic Amines (modified amino-acids with bilogical function) - Tyrosine + OH group → Dopamine + OH → Noradrenaline - Dopamine o metabotropic receptors: (D1 -D5) -G-protein receptors o two populations of dopaminalgic neurons present in midbrain (Nuclei) o 1st population/ Nucleus: ventral tegmental area (Ventral tegmentum) → involved in processing of rewarding stimuli → involved in mental illnesses, psychoactive drugs o 2nd population/ Nucleus: substantia nigra → directly directed to Parkinson‘s disease: Neurodegeneration in substantia nigra - Noradrenaline o metabotropic receptors (α, β -receptors) o inactivation by monoamine oxidase (MAO) → neurotransmitter signaling needs to be stopped, otherwise elongated or even continuous AP o Locus coeruleus in brainstem (blue nucleus) → main source for noradrenaline in brain o functions: multimodal alertness and motivation & dynamic reorganisation of activity in neuronal circuits o “norapenaphrine” is the US-term important for neuropeptide transmission - Release is not into cleft but somewhere around -> diffusing around target cell and also influencing other synaptic connections that are close by - Dense core vesicles examples: Vasopressin, Dynorphin, Substance P, Hypocretin, NPY, MCH, POMC Chemical synapse vs. Electrical synapse - chemical: all synapses we‘ve talked about so far o monodirectional delayed response o delay because of all the processes that need to happen before the signal is transmitted, such as vesicle contraction, fusion, receptor docking, … - electrical: o no synaptic vesicles o no synaptic cleft → membranes bridged together → Gap junctions: densely packed channels (one Connexus formed by 6 Subunits of protein connexins, each having 4TM segments) o no pre/ post synapse → signals can go on in both directions o bidirectional (signal can go in both directions), no delay → ions flowing into the other neuron freely o neurons functioning in networks with synchronized activity Introduction to Neurobiology – week 10 Lesson: 13th of January 2023 Signal processing From a graphic we could read the nessessary depolarization in different neuronal cell body parts to initiate an action potential Apparently, at the last segment before the axon, significantly less cations are needed inside the cell Why is that? - voltage-gated sodium channels distributed all over membranes of soma and dendritic processes - BUT highly concentrated at axon initial segment - firing threshold much lower at initial segment → much easier to initiate an action potential - incoming signal from previous cell → change of membrane potential → traveling down to axon initial segment in passive way (voltage changes/ decreases) → if epsp not strong enough, it doesn‘t reach threshold so no action potential fired to next cell - backpropagating action potentials: influences membrane potential and state of synapses along dendritic processes and membrane of soma of neuronal cell → depending on how active cell is, it‘s influencing state of own synaptic contacts as well Summation of EPSPs - temporal summation o two synaptic inputs on same neuron right after the other → strong enough change on post- synaptic membrane → firing of cell - spatial summation o two incoming signals from two different positions of cell (possibility for synaptic plasticity Synaptic Integration - epsp These cells are generating synaptic contacts often on dendritic processes and even on each other (axoaxonal contact) Leads to deporalization - ipsp Inhibitory cells are generating synaptic contacts usually directly on soma and close to axonal process Leads to hyperpolarization inhibitory and excitatory signals to accumulate/ erase each other: one inhibiting signal may wipe out the depolarization of an excitatory one Memory trace in a Network - Mayor cell type here is the pyramidal neuron - 1 neuron providing strong synaptic input on the following cell (basically innervates it) → follow up cell has a connection to 2 further cells which it innervates - reinforcement needed: one synaptic input is too weak to elicit an action potential from another set of neurons → leads to a strong biochemical change in last neuron (which maintains information that there were 2 lines of neuronal contact providing the information) → changed neuron will react in a slightly different manner next time the incoming information arrives/ reacts slightly different this is called synaptic plasticity Synaptic plasticity - This different reaction is namely that a larger/ smaller input is given by the respective innervating axon to our neuron - Not the amplitude of the action potential changes, but the reaction of the innervated cell 1. Homosynaptic modulation - rapidly repeated stimulation increases the size of epsp, effect lasts only for 100ms (depends on time between two stimuli) - only temporary increased strength in answer - tetanic: High-frequency - Cause of this connected to calcium – better look this up - posttetanic potentiation o high-frequency stimulation („tetanic“) increases the size of epsps, effect lasts for minutes - synaptic depression o repeated weak stimulation gradually decreases the size of the epsp: habituation 2. Heterosynaptic modulation - a model for simple associative learning – change in synaptic strength as a result of experience and training. (sea hare Aplysia used as a model organism by Eric Kandel‘s group – actually a snare but the large ears make it look a little bit rabbit-ly) o the sea hare was used because its easy to hold in a laboratory and the cells are big and easy to acess o and simple network connections o The gills are very high sensory (for protection purposes) Touching them leads to a withdrawal reflex o tests of touching animal´s gills and observing withdrawal reaction decreasing with time (Habituation) if touching gently, each time the withdrawal will occur weaker, until it will stop -> learning process o another strong input on ex. other part of animal can sensitize network connection → gills will be withdrawn again (Sensitization), even if the strong touch is not on the gills themselves Sensitization - interneuron functioning with serotonin (biogenic amino neurotransmitter) - serotonin released → signal through serotonin receptors on sensory neuron at nerve terminal - → leads to potassium current reduction (activity of sensory neuron is modulated via the interneuron) o Recap: Action potential: Sodium flowing into cell → repolarisation via potassium current - repolarisation longer because the pump needs longer to return to the resting membrane potential when the potassium channels are modulated and working slowlier due to Proteinkinase A → elongated action potential → voltage change stays for longer period - voltage gated calcium channels open due to an action potential will be open for longer → more calcium → stronger release of neurotransmitter Serotonin Receptors - TM (Transmembrane Segment) G-protein coupled – Receptor - binds Seratonin as Neurotransmitter → conformational change → induces activation of G-protein (alpha subunit binds GTP) → alpha subunit + GTP is active form in this case, activating the effector: adenylyl cyclase → adenylyl cyclase induces production of cyclic AMP (cAMP) → cAMP binds to another enzyme (proteinkinase A) → activated proteinkinase A starts to phospholate target molecule (potassium channel) o Kineases usually always phospholate stuff → Phosphorylation of channel → less Potassium ions can get through → current reduction Formation of cyclic AMP - adenylyl-cyclase cleaves 2 phosphate groups off ATP and binds the 1 left Phosphate group to other part of adenosine molecule → cyclic adenosine-monophosphate (cAMP) - cAMP functions as a second messenger in cell → transducing signals further on Summary - serotonin binds to its receptor - cAMP level increases - proteinkinase A becomes activated - K -channel reduces conductivity ⁺ - AP is prolonged - Ca² -current increases ⁺ - More transmitter is released at the sensory nerve terminal Studies with mutant flies - easy mutation - easy screening of the genes and the structural and behaviouraöl changes - good tracking back to which genes are responsible learning of an odor discrimination task studies on two types of flies that were considered to be very bad at learning (“Stupid flies”) - „rutabaga“ and „dunce“ mutant flies (bad at learning odor discrimination) - change in level of cAMP (mutation affecting genes responsible for proper level of cAMP) - rutabaga: mutation in AC (adenylyl-cyclase) – preventing proper levels of cAMP - dunce: mutation in PDE – responsible for cleavage of cAMP (downregulation of cAMP- level) - change in cAMP level → change in function of ion channels affecting learning process - proper training → flies were able to maintain odor discrimination for long time → longterm memory - persistent amount of cAMP → activation of transcription factors (CREB) - CREB proteins in cytoplasm entering cell nucleus and connecting to promotor section of respective gene → modulating expression of gene (blocking/initiating) Longterm learning and memory formation Grow new connections or strenghten existing connections by changing structural aspects and the amount of molecules that are present at these synapses Which are the key properties of NMDA-receptors relevant for the induction of LTP? The extended depolarization of the postsynaptic dendritic tree that NMDA receptors require to become receptive to glutamate is achieved by a series of action potentials. →Mg2+ block is released →Activation of NMDA receptors (therefore, a strong and frequent stimulus is needed to activate these receptors). - Calcium ion permeability, which act as a "second messenger" causing various metabolic effects in the target cell. → additional activation of enzyme producing nitric oxid (gaseous neurotransmitter) by Calcium → activation of CaM-kinase II → phosphorylates itself → long-term effect →induces insertion of additional non-NMDA receptors into the postsynaptic membrane → increase in epsp Introduction to Neurobiology – week 11 Lesson: 27th of January 2023 LTP in the Hippocampus - principle cell types for that particular region: o pyramidle (neuronal) cells (cornu ammonis - named after Egyptian god – ammons horn, neocortex) o granule cells (dentate gyrus) - Experiments: o isolate and slice hippocampus into ½ mm tissue slice o all cells within slice are intact except for those at the sliced edge o cells similar orientation as slice o explore with electrophysiological means - three-synaptic curcuit: o first synaptic connection from entorhinal cortex into hippocampus to granule cell (dentate gyrus region) – Tractus perforans o axonal processes of dentate gyrus cells construct mossy fibre o mossy fibre axonal processes construct 2nd synaptic connection to CA3 pyramidle neurons o one branch of axonal processes from CA3 towards CA1 pyramidle cells o post-synaptic memory formation o maintaining level of potentiation for long time – two hours or even much longer o memories in hippocampus only for short term memory o long term memory in associative cortex areas - Hippocampus brain region known for memory formation and retrieva o Three-layered structure Associative long term potentiation - why is neuronal cell suddenly answering with much higher epsp although receiving same stimulus strength → associative long term potential - excitatory inputs (axonal terminals from differnt neurons) making synapse into neuron - weak stimulation of axonal process = weak epsp - training cell with high frequency stimulation (many stimulations within short intervall) = high depolarisation in part of dendritic tree = increased epsp after weak stimulation - Response due to NMDA type Glutamate receptors Role of NMDA-receptors for LTP - can be ionotropic and metabotropic (ionotropic in this case → ion channels) - subclass of ionotropic receptors o non-NMDA receptors only need to bind glutamate → conformational change o NMDA receptors have Mg² blockage ⁺ within channel → need depolarisation to remove Magnesium from pore → voltage & ligant gated channels (also lets through Calcium) - Calcium entering into cell functioning as a 2nd messenger initiating several cellular processes - mainly modifying strength of synapse (can occur pre- and postsynaptically) - presynaptic effects: o production of nitric oxide (NO), NO can freely diffuse out from postsynapse and into presynapse (retrograde messenger) o enhanced exocytosis of synaptic vesicles - postsynaptic effects: o activation of CaM-kinase II (binds calcium which activates enzyme which places phosphate groups onto target proteins) o one of the target proteins is the enzyme itself → autophosphorylating itself → alongating activity of itself → persistent activation o phosphorylation of AMPA-receptors (another target protein) o recruitment of more AMPA-receptors leading to increasing strength of synapse Recruitment of non-NMDA receptors - if we only have NMDA-receptors in post- synaptic membrane, synapse is silent - LTP formation: o post-synaptic membrane had been depolarised → Magnesium-blockage removal o Glutamate opens NMDA receptors o Calcium can enter into post-synaptic cell, initiating cellular processes recruiting more non-NMDA receptors into postsynaptic cell o next time with same stimuli there are more non-NMDA receptors present → increased epsp Dendritic spines - different structures (big head) depending on size and strength of synapse - spines receive vast majority of excitatory input - head size proportional to postsynaptic surface - about 1 spine per micrometer on dentrites (density) - dendritic processes can change due to ex. Aging, experience, loss of memory, etc - dynamic changes according to novel information the cell is receiving o if stronger signals, dendritic spines will enlarge in structure (building in more AMPA type structure receptors) o less signal → structure decrease - receptors need to be renewed all the time - introduce protein of AMPA receptor locally → increased dendritic spine density - transecting root from cell body to dendrite – losing AMPA receptors → synapses will not function → withdrawal of dendritic spine - transection + AMPA → presenting receptors locally again → dendritic spines increase Function of dendritic spines - compartmentalisation - incoming information to dendritic spine with big head, small neck o stiumation of pre-synapse → calcium influx o calcium freely diffusing into dendritic shaft but limited diffusion due to small neck o can diffuse into other dendritic spines and initiate their signalisation LTP shortly explained High frequency stimulus leads not only to an opening of non-NMDA, which lead to a Sodium influx, but also to an opening of NMDA due to the non-NMDA depolarization. Calcuim enters through the NMDA-channels, which activates three enzymes: - NO-synthase: leads to a flow of NO (Stickoxid) into the presynapse – higher release of vesicles -> more transmitters (happens every time synapse is activated) - CaMKII: builds more non-NMDA receptors into the postsynapse – leads to a higher depolarization following a stimulus (happens every time synapse is activated) - Adenylylcyclase: Transforms ATP into cAMP – activates protein synthesis to build proteins, which can build new synapses or activate resting ones (synapse has to be activated over very long period of time to to this, but outcome is very long-lasting) Introduction to Neurobiology – week 12 Lesson: 3rd of February 2023 Spinal cord - segmental organisation o neck (8) o breats (12) o lumbal (5) o caral (5) - segments within spinal cord corresponding with segments of vertebrae - However, not corresponding to size! → mismatch o Spinal cord ends at about the first lumbar vertebre - spinal nerves o pairs, entering and leaving cord o motoric branch coming from frontal part of spinal cord o dorsal part where axonal/ sensory processes are entering - 2 enlargements: Cervical and Lumbar (surface for getting sensory information and motoric outputs) - inner part: grey matter o neuronal cell bodies - outer part: white matter o ascending and descending axonal processes o most of these axonal processes are myelinated (responsible for white colour) - different proportion of grey and white matter o last segments: little white matter, mostly neurons: hardly any information collected/ released o upper segments: lower grey matter, population of axons is increasing, more input/ output on cord - dorsal horns : through which sensory information will reach cord o information gathered by specific neurons (dorsal root ganglia (pseudo-unipolar cells)) bulky region! o peripheral and central axonal process - ventral horn: motoric processes from motor neurons leaving cord targeting other cell types - reflex arc: small circuit only involving 1 – 2 synapses (monosynaptic) - pseudounipolar cells in DRG – looking like they had just one process, but it splits into central and periphal axon Knee jerk (patellar reflex – monosynaptic reflex) - sensory information collected via pseudo-unipolar cells (dorsal root ganglion) - ending of unipolar neuron within muscle spindle (understands tension and tension differences) - sensory input reaching spinal cord and provides motor output via motor neurons in ventral horn - axonal processes leave spinal cord through ventral root - terminate in form of neuromuscular junction within muscles - very fast because it is a monosynaptic reflex more detailed: Afferent collateral inhibition - sensory fiber bringing sensory information into spinal cord giving collaterals (branching) - one branch will have direct synaptic connection to motoneurons (to extensor muscle) - other branches to interneuron which is synapsing to motoneuron (to flexor muscle) - extensor muscle to actively relax the muscle – inhibition the flexor muscle Summary components of a reflex arc - sensor (e.g. muscle spindle) - afferent fiber (sensory fiber) o eventually interneurons - efferent fibers (motor fibers) - effector (muscle) Central Control Pathways - information going up to cortex (to contralateral side) through ascending pathways o sensory information like temperature, pain - touch: ipsilateral side - input from cortex through descending pathways (cortex might want to modify some output , e.g. for voluntary movement), on ipsilateral side Brown -Sequard Syndrome - half-sided transection - worst case: paralysis → loss of types of sensation o loss of touch in paralysed leg, loss of temperature and pain in other leg General overview central system Functions of the spinal cord - fast automatic reactions o reflexes: motosynaptic, polysynaptic - control of basic motor functions o routine movements like walking, running, rhythmic activity, servo function - → relief of higher brain centers from detailed movement control Spinal pattern generators - ale swimming: spiking patterns recorded from two opposing spinal roots o contraction of one muscle group while relaxation of opposing muscle group → rhythmic firing - Half-center model o driver (neuron getting activated) o two axonal branches going to interneurons, providing excitation/ inhibition towards flexor/ extensor o inhibitory interneuron connections providing reciprocal inhibition Ion channels as pattern generators - driver cell initiates excitatory signalisation onto neuron - Ca² - Channels open and Calcium ions flow into cell → depolarisation - Ca² - activated K -Channels → Potassium runs out of cell → self termination - repolarisation via Potassium flowing out closes Calcium channels - Removal of Ca² → closure of Potassium Channels ⁺ - → opens Calcium channels again … - → Rhythmic possibility to change membrane polarity based on Ca² - oscillations Regulation of muscle force - motor unit: motorneuron and all target muscle fibers this particular cell is innervating - emanating axon will leave spinal cord through ventral horn - travel dependent where target muscles are - axon terminal at end, reaching several muscle fibers providing neuromuscular junction (skeletal muscle fibers, not cells!) Ways to modulate muscle force: - frequency modulation (ex. Stronger synaptic input) → induce contraction of fibres in shorter or elongated way - bigger motorneurons have more branches providing innervation to more muscle fibers o higher force o fast and fatigating o coarse control (bigger units contracted at the same time) o strong signal needed because membrane surface in proportion to neuronal size is small o big axon - speedy - normal motorneurons o fast and fatigue resistant o somewhat stronger signal needed - smaller motorneurons o low force o small axon, slow o delicate control o even with lower signal we can recruit/ activate cell because membrane surface in proportion to neuronal size is big Parts of the Nervous system - CNS (brain, spinal cord) - PNS (peripheral nervous system) o sensory nerves and motor nerves o somatic reflex arc - ANS (autonomic nervous system) o responsible for modulation and organisation of mainly unconscious function of organs o sensory information from sensory nerves o parasympathetic/ sympathetic nerves used for modulation of functions - opposite modulations towards organs o autonomic reflex arc ANS (Autonomic Nervous System) - Sympathetic branch o always Ganglion (close to spinal cord) in between target and neuron which is originating in spinal cord o preganglionic (in intermediate part of grey matter) o and postganglionic (long axonal process) neuronal cell to targeted organ ex. Heart o acetylcholine as neurotransmitter - Parasympathetic branch o Ganglion much closer to target organ o acetylcholine as neurotransmitter o postganglionic cell functioning with muscarinic type of acetylcholine receptors on target cells - ENS (Enteric nervous system) o Considered as the third division of the ANS, defined as sympathetic, parasympathetic and enteric o ENS is large – human ENS contains more than 100mio neurons -, complex and uniquely able to orchestrate gastrointestinal behaviour independently of the central nervous system o locally able to modulate function of our digestive system o most important parts: myenteric (Auerbach´s) plexus submucosal (Meissner´s) plexus - heart rhythm/ rate regulated by specialised heart muscle cells (self-inducing contraction) - Convergence: one post-ganglionic cell receiving multiple input signals → security of transmission - Divergence: from same level of spinal cord, preganglionic cell provides axonal branches/ synaptic connections to ceveral postganglionic neurons → spreading signal by activating more postganglionic neurons Summary ANS - sympathetic branch: sympathetic chain in the breast and lumbal region o „fight or flight“ - parasympathetic branch: 10th cranial nerve (nervus vagus), sacral region o „rest and digest“ Sympathicus: postganglionic via Norepiphrine, exciting the heart muscle Parasympathicus: postganglionic via mAChR, inhibitimg the heart muscle