Neural Impulse PDF
Document Details
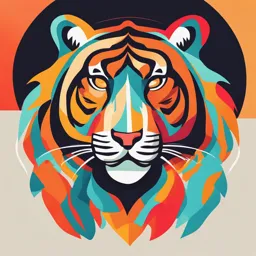
Uploaded by ReachablePoltergeist
CMR University
Dr Saranya TS
Tags
Summary
This presentation covers neural impulses, including resting and action potentials, neuron structure, and types. It also discusses the roles of different types of glial cells.
Full Transcript
Neural Impulse Dr Saranya TS Neurons Neurons are the basic information-processing structures in the CNS. They are electrically excitable cells that process and transmit information around the nervous system. Neurons transmit information either by electrical or chemical signaling. A ty...
Neural Impulse Dr Saranya TS Neurons Neurons are the basic information-processing structures in the CNS. They are electrically excitable cells that process and transmit information around the nervous system. Neurons transmit information either by electrical or chemical signaling. A typical neuron possesses a cell body (the soma), dendrites, and an axon. Dendrites are filaments that emanate from the cell body, branch numerous times, and give rise to a complex dendritic tree. An axon is like the wire in an electrical cable. It starts at the cell body at the axon hillock site and travels to the site in the nervous system where it connects with another nerve cell or a different type of cell, such as muscle. Neuron structure/ anatomy Types of neurons Efferent neurons (motor neurons): these direct information away from the brain towards muscles and glands. Afferent neurons (sensory neurons): these transmit information to the central nervous system from sensory receptors. Interneurons: found in the central nervous system, these pass information between motor neurons and sensory neurons. Mixed neurons: that mimics both motor and sensory neurons. Glial cells The glial cells are simply the so-called glue (as the name implies) by which the nervous system is held together. Neuroglial cells are the other major cell type in neural tissue; they provide structural integrity and nutrition to the nervous system and maintain homoeo stasis. Types of glial cells: A number of different types of glial cell exist. Some can be found in the central nervous system and others are essential to the functioning of the peripheral nervous system. Types of glial cells Oligodendrocytes (CNS): Oligodendrocytes surround axons in the CNS forming a myelin sheath that insulates the axon and makes it possible for electrical signals to be generated and propagated more effectively. Schwann cells (PNS): The cell in the PNS that is functionally equivalent to the oligodendrocytes in the CNS, the Schwann cell, only insulates one discrete axon. Schwann cells are specialized types of glial cells that provide myelin insulation to axons in the peripheral nervous system. Astrocytes (CNS) Astrocytes are responsible for maintaining the external chemical environment around nerve cells. Microglia (CNS) Microglia are glial cells that serve the CNS immune system and are also specialised macrophages. Microglia are important protectors of the central nervous system, and guard and support the neurons of the CNS. Neural Impulse Resting Potential: The resting potential (or resting voltage) of the cell is the membrane potential that is maintained if there are no action potentials or synaptic potentials present. The intracellular fluid of a neuron at rest is more negatively charged than the extracellular fluid, and this polarity difference is termed the resting potential. The resting membrane potential of a neuron is about -40 millivolts (mV); this means that the inside of the neuron is -40 mV less than the outside. The disparity between voltages across the cell membrane is due to the fact that there is a higher concentration of positive ions outside the cell than inside the cell. There is approximately 10 times more sodium (Na+ ) on the outside of the cell and approximately 20 times more potassium (K+ ) on the inside. This is frequently called the cell’s concentration gradient. Action Potential The membrane resting potential is present when a neuron is at rest. However, when our nervous system transmits information, an action potential or a nerve impulse occurs. An action potential is a short-term event in which the electrical membrane potential of a cell rapidly increases and falls. Positive ions flowing into the cell will reduce the negative charge and thus reduce the charge across the entire membrane; we refer to this as depolarisation. When depolarisation gets to around +55 mV (the tipping point) a neuron will give off an action potential. No action potential will fire if the neuron does not reach the threshold level. Once the threshold value is reached, an action potential of a fixed size will always fire; and for any given neuron, the size of the action potential is always the same. Often this is referred to as the all- or-nothing principle. The exchange of ions across the neuron membrane causes nerve impulses or action potentials to take place. A stimulus, for example at the touch receptors, first causes Na channels to open, and because there are many more Na ions on the outside, and the inside of the neuron is negative relative to the outside, Na enters the neuron. Thus, the neuron becomes both more positive and depolarised. Then K channels open (they take a bit more time than Na channels) and K rushes out of the cell, reversing the depolarisation. When Na enters, the membrane potential increases, and when K exits, it drops sharply back down, giving rise to the alternative name for this potential: the spike potential. Na channels then start to go back to their normal closed state, which causes the action potential to revert towards –40 mV (a repolarisation). However, the membrane potential actually moves past –40 mV to nearly –60 mV (a hyperpolarisation) due to the K channels remaining open just a bit too long. Ion concentrations will steadily return to the resting membrane, and the cell will return to –40 mV. For a short period in the middle of the action potential, the neuron is totally resistant to additional stimulation. This is known as the absolute refractory period, where the neuron cannot make another action potential. The absolute refractory period precedes another brief period, known as the relative refractory period, during which the neuron can generate another action potential but the stimulus must be of greater intensity than normal. Synaptic transmission/ neural communication Communications across the synapse are facilitated by neurotransmitters – chemicals that are synthesized by neurons and utilized to engage in communications with other cells. The presynaptic ending or synaptic terminal, also known as the synaptic knob, bouton or button, holds cell organelles like mitochondria and neurotransmitters. The neuron sending the impulse triggers the migration of vesicles which contain the neurotransmitter to the membrane of the synaptic terminal. The region of the synapse that releases neurotransmitters is called the active zone. Here, cell adhesion molecules keep the membranes of the pair of neighboring cells close to one another. The postsynaptic cell may be a muscle cell or a gland, not just another neuron. Stages of neural impulse/ Neural impulse cycle Polarization: The resting state of the neuron, with a negative charge inside and a positive charge outside the membrane. Depolarization: The stimulus that triggers the impulse, causing sodium channels to open and sodium ions to rush into the cell, making it more positive inside. Repolarization: The restoration of the resting state, with potassium channels opening and potassium ions flowing out of the cell, making it more negative inside. Hyperpolarization/ Recovery period: The overshooting of the resting state, with the membrane potential becoming more negative than the original level. Refractory period: The time during which the neuron cannot fire another impulse, until the sodium-potassium pump restores the original ion distribution. Problems that affect neural impulse Axon damage Sodium potassium deficiency Neurotransmitter deficiency Dendrite damage