Membrane Physiology Summary PDF
Document Details
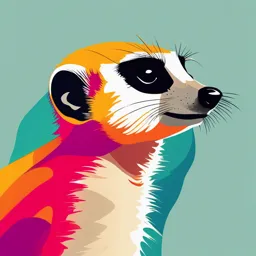
Uploaded by HandsDownStrontium7431
University of Birmingham
Tags
Summary
This document summarizes membrane physiology, covering cell membrane structure, functions, ion channels, electrochemical gradients, equilibrium potential, and resting membrane potential. It explains how these components interact to maintain membrane potential.
Full Transcript
**L02 -- Membrane Physiology** **Structure of the Cell Membrane** - The structure of the plasma membrane is a bilayer consisting of phospholipid molecules with the phosphate heads facing outwards (on the surface) and the fatty acid tails facing the inside of the bilayer. - Thi...
**L02 -- Membrane Physiology** **Structure of the Cell Membrane** - The structure of the plasma membrane is a bilayer consisting of phospholipid molecules with the phosphate heads facing outwards (on the surface) and the fatty acid tails facing the inside of the bilayer. - This makes the membrane impermeable to polar molecules. - The plasma membrane's structure also contains proteins such as ion channels (passive movement of ions) and ion pumps. Ion channels can be: - Voltage-gated (generate action potentials) - Ligand-gated - Mechanically gated - Non-gated - Leak (always open) These factors allow the plasma membrane to create an intracellular environment which is different from the external environment. **Functions of the Cell Membrane** - **Defines boundaries of the cell** -- cells have varied shapes to enable them to carry out their functions. - **Encloses the cell's organelles** -- nucleus, Nissl substance, mitochondria, etc. - **Enables the cell to create an internal environment** that promotes the normal function of the cell -- creates an internal environment that is different from the outside of the cell. - The cell membrane is able to influence the composition of the intracellular compartment but not the extracellular compartment. (Extracellular compartment aka interstitial space and intracellular environment are both largely aqueous environments). **Ion Channels** - The exposed surface of a protein can be chemically heterogeneous. - E.g., the exposed ends of channel proteins are hydrophilic, and their middle surfaces (embedded in the membrane) are hydrophobic. - Ion channels have **ion selectivity** and a **gating mechanism** -- they are essential for controlling Em (ion movement is passive). **Electrochemical Gradients** The accumulation of ions in different concentrations inside and outside cells leads to the establishment of 2 gradients: - **Chemical gradient** -- caused by concentration difference inside and outside the cell. - **Electrical gradient** -- membrane potential caused by difference in polarity (charge) inside and outside the cell. The combined effect is known as the **electrochemical gradient**. The ions involved in setting up membrane potential are mainly **Na+** and **K+**, in addition partly to **Cl-** and **Ca2+**. **Ion** **Concentration Inside (mM)** **Concentration Outside (mM)** ---------- ------------------------------- -------------------------------- **K+** 150 5.5 **Na+** 15 150 **Ca2+** 1 x 10⁻⁷ 1.8 **Cl-** 9 125 **Equilibrium Potential** The **equilibrium potential** is the membrane potential where there is no net movement of one particular ion into or out of a cell. Each ion has its own equilibrium potential, as this depends on the charge of the ion and its concentrations inside and outside the cell. **Example of K+ Equilibrium Potential:** 1. The inside of the cell is more **negative** than the outside (the inside of all cells contains a minute excess of anions leading to a negative voltage within the cell). 2. Initially, more **K+** ions are inside the cell, so a **chemical force** pushes the ions outwards through leak K+ channels. There is also a weak **electrical force** attracting K+ ions back into the cell, as the inside is more negative. 3. **Chemical force \> electrical force** at first, so K+ ions are leaving the cell. 4. As K+ ions leave, the negative charge inside increases, which increases the electrical force attracting K+ ions back. Eventually, **chemical force = electrical force**, leading to no net movement of K+. The membrane potential at which this happens is the equilibrium potential for K+ (**EK+**). If the membrane was only permeable to K+ ions, the resting membrane potential of the cell would be equal to EK+. However, the membrane is also permeable to other ions. The equilibrium potential for an ion can be calculated using the **Nernst equation** (do not need to memorize). **Interaction of Other Ions to Create Resting Membrane Potential** Following the equilibrium potential of K+, we now consider **Na+**: 1. There are more **Na+** ions outside the cell than inside, so a **chemical force** drives Na+ into the cell. 2. The inside of the cell is negative, so there is a strong **electrical force** acting inwards. 3. **Na+** enters the cell, and as this happens, the inside becomes less negative. The electrical force weakens, and the movement of K+ ions out of the cell begins. Eventually, there is equal movement of **Na+** into the cell as **K+** out of the cell. The membrane potential is now very close to the resting membrane potential. The interaction of **Cl-** must also be considered for a more accurate value. **Resting Electrical Properties** - **Resting Membrane Potential (Em)** = -60 to -70 mV - This means: - There is an excess of negative charge inside the cell. - The voltage is stable, so there is an equal movement of ions inside and outside the cell. **How is this achieved?** 1. **The permeability of the membrane to the ion**: How easily can ions cross the membrane? - The plasma membrane contains **non-gated (leak)** channels for K+ and Na+. - Therefore, the membrane has a permeability to each ion (**PK**, **PNa**). - The number of leak channels for each ion sets the permeability for that ion. 2. **Factors influencing ion movement (flux) across the membrane**: - **Chemical gradient**: There is an unequal ion distribution, so ions will flow down their concentration gradient. - K+ concentration is higher inside, so it is driven to leave the cell (efflux). - Na+ concentration is higher outside, so it is driven to enter the cell (influx). - **Electrical force**: Ions are charged (Na+/K+), thus are attracted by the voltage inside the cell (**Em**). - At negative **Em**, the drive for K+ and Na+ to move into the cell (influx) occurs. **Driving Ion Movement Across the Membrane** To move an ion across the membrane electrically: - The membrane must possess channels permeable to that ion. - There must be an electrical potential difference across the membrane. The **Goldman equation** can be used to estimate the resting membrane potential, given the concentrations of the three ions inside and outside the cell, and their relative permeability. Nernst equation can be simplified to **E~ion~ = 61mV x log~10~ (\[ion\] out / \[ion\] in)** Since the membrane is much more permeable to K+ (due to more leak channels), the resting membrane potential of neurons is closer to **EK+** than **ENa+**. The **RMP** of a neuron is approximately -70 mV. **Role of the Na/K Pump** The **Na/K pump** plays a role in maintaining the resting membrane potential. Due to the net entry of Na+ and net exit of K+, the pump neutralizes this movement by pumping: - 3 Na+ out of the cell - 2 K+ into the cell The **Na/K pump** does NOT create the resting membrane potential but only helps **maintain** it. **Electrically Excitable Biological Tissues** - A defining characteristic of electrically excitable tissues is their ability to let out a momentary discharge of the standing electrical potential between the intracellular and extracellular compartments. - All living biological tissues have a resting membrane potential. - In nervous tissue, this process is the mechanism for electrical communication between cells. - Upon discharge, the cell membrane works to restore the resting membrane potential. - The process of death is characterized by the permanent discharge of resting membrane potentials of electrically excitable tissues. **Factors That Define Whether or Not Particles Cross the Cell Membrane** - Size of particles - Electrical charge of particle - Whether particles are recognized by specialized transport systems - Solubility of molecules in water - Transport ATPases (ion pumps) - Presence of ion channels (leak channels and selective or non-selective channels) **Channel Ions** - Peripheral or trans-membrane spanning proteins span the full thickness of the cell membrane. - They facilitate the passive movement of ions across the membrane. - Some are selective in which ions cross; others are less selective in terms of which ions they conduct. - **Selectivity filter** -- filters structures for size and charge. - **Cavity** -- if it meets the selectivity filter, it enters the cavity, in hydration, molecules pick up water and reach maximum size. - **Gating** -- only certain ions can exit the gate (e.g., water and oxygen enter, but not potassium ions, as they are overhydrated). **Ion Pumps** - **Maintains RMP** -- Net entry of Na+ and net exit of K+. - The **Na/K pump** neutralizes this movement by pumping 3 Na+ out and 2 K+ into the cell. - It **doesn't create the RMP**, but only **maintains** it. - Ion pumps require **ATP** to maintain the resting membrane potential. **L03 -- Neuronal Excitability** **Initiating the Action Potential** For an action potential to be initiated, a small influx of Na+ ions must occur in the neuron, caused by a stimulus. This stimulus can be: - **Mechanical** (e.g., pressure in mechanoreceptors), - **Chemical** (e.g., neurotransmitter release at synapses), - **Electrical** (e.g., current flow between heart muscle cells). This influx of Na+ must be strong enough to depolarize the membrane potential from **-70 mV** (resting membrane potential) to approximately **-55 mV** (threshold potential). If the threshold is not reached, an action potential will not be generated. However, if the threshold is surpassed, a **positive feedback loop** will amplify the depolarization, resulting in an action potential. This process follows the **all-or-nothing principle**, meaning the action potential will always be of the same magnitude once initiated, regardless of the initial stimulus strength. Graded depolarizations occur when a stimulus causes a small change in the membrane potential, and if this depolarization reaches the threshold, an action potential is generated. **Equilibrium Potential** The **equilibrium potential** (Eₖ) refers to the membrane potential at which there is no net movement of a specific ion into or out of the cell. For **K+** ions: - The concentration of **K+** is much higher inside the cell (150 mM) than outside (5.5 mM). - The **chemical force** pushes K+ ions out of the cell via leak channels, while the **electrical force** (created by the negative charge inside the cell) pulls K+ ions back in. - Eventually, these forces balance each other, and there is no net movement of K+. This point is known as the **equilibrium potential for K+ (Eₖ)**. **Stages of the Action Potential** If the membrane reaches the threshold potential, the following stages occur: 1. **Threshold Reached:**\ Voltage-gated **Na+** channels open, causing an influx of Na+ into the cell. This makes the membrane potential more positive. 2. **Depolarization:**\ As the membrane becomes more positive, more **Na+** channels open, leading to more Na+ influx, further depolarizing the cell. This forms a **positive feedback loop**. 3. **Peak:**\ At the peak of depolarization, the membrane potential can overshoot and reach 0 mV, or even become positive in large neurons. At this point, the voltage-gated **Na+** channels close, and **K+** channels open, allowing K+ to flow out of the cell. 4. **Repolarization:**\ As K+ ions leave the cell, the membrane potential drops back toward the resting potential. The voltage-gated **K+** channels eventually close. 5. **Hyperpolarization:**\ There is a brief delay in closing the voltage-gated **K+** channels, leading to excessive K+ efflux, causing the membrane potential to dip below the resting membrane potential, known as **hyperpolarization**. The membrane potential then returns to normal. 6. **Na+/K+ Pump:**\ The **Na+/K+ pump** restores the concentration gradients of Na+ and K+, helping maintain the normal resting membrane potential. Action Potential \| Biology for Majors II **Refractory Period** The **refractory period** is the time following an action potential during which another action potential cannot be initiated. This occurs due to the prolonged inactivation of voltage-gated **Na+** channels. - **Absolute Refractory Period:**\ During this time, no new action potential can be generated, regardless of the strength of the stimulus. This is due to the inactivation of Na+ channels. - **Relative Refractory Period:**\ During this period, it is possible to generate a new action potential, but a stronger-than-normal stimulus is required because some Na+ channels are still inactivated. **Conduction of Action Potentials** The conduction speed of action potentials differs between **myelinated** and **unmyelinated** neurons: - **Unmyelinated Neurons:**\ In unmyelinated axons, Na+ ions entering the cell diffuse in both directions, causing adjacent Na+ channels to open. This sets up a local circuit that propagates the action potential in a relatively slow manner. **Conduction speed:** \~0.1 meters per second. - **Myelinated Neurons:**\ In myelinated axons, action potentials occur only at the unmyelinated **nodes of Ranvier**. This results in **saltatory conduction**, where the action potential \"jumps\" between nodes, making the process much faster and more energy-efficient. **Conduction speed:** \~100 meters per second. The larger the axon diameter, the faster the conduction speed. **L04 -- Somatic Nervous System** **What is the Somatic Nervous System & Its Importance?** The **Somatic Nervous System (SNS)** is a part of the peripheral nervous system (PNS) that controls voluntary movements and transmits sensory information. Its key functions include: - **Awareness of the external world:** It enables us to sense and respond to stimuli from the environment. - **Localization of sensations:** It helps us pinpoint the origin of sensory inputs across the body. - **Proprioception:** It provides information about the position and movement of our body in space, allowing us to maintain posture and coordinate movements. **Overview of the Nervous System** The nervous system is divided into two main parts: 1. **Central Nervous System (CNS):** Comprising the brain and spinal cord. Both encased in meninges (DAP) and suspended in CSF. 2. **Peripheral Nervous System (PNS):** This includes all nerves outside the CNS, connecting it to the body's tissues and organs. In the PNS, two types of neurons are involved: - **Afferent neurons** send sensory information from receptors in the body to the CNS. - **Efferent neurons** transmit motor commands from the CNS to muscles and glands (effectors). The **Autonomic Nervous System (ANS)** operates involuntarily, controlling functions like heart rate and digestion, and contrasts with the voluntary nature of the somatic system. **Structure of Neurons** Neurons are the fundamental units of the nervous system. Key structures include: - **Cell Body (Perikaryon):** Contains the nucleus and organelles. - **Axon:** Conducts electrical impulses away from the cell body. - **Axon Hillock:** Region where AP is generated (produces kink in neurone). - **Dendrites:** Receive signals from other neurons. - **Myelin Sheath:** Insulates axons, speeding up the transmission of nerve impulses. - **Nodal membrane:** Membrane at the node of Ranvier - **Internodal membrane:** Membrane at the regions that are myelinated **Somatic Nervous System Functionality** - **Somatic Efferent Neurons:** These transmit motor commands from the CNS to skeletal muscles, resulting in voluntary movements. The SNS plays a role in muscle contraction and motor tone. - **Somatic Afferent Neurons:** These neurons carry sensory information from the body\'s surface to the brain, enabling the sensation of touch, temperature, and pain. The SNS operates in a **bi-stable** state: either **active** (awake and responding to stimuli) or **inactive** (during sleep). During **rapid eye movement (REM) sleep**, muscle activity is largely suppressed. **Characteristics of Somatic Neurons** - **Efferent (Motor) Neurons:** - **Multipolar morphology:** The cell body is located in the CNS (e.g., ventral horn of the spinal cord) with several branching dendrites. - **Myelinated:** These neurons are typically myelinated for faster transmission, with **alpha motor neurons** (fast, responsible for powerful contractions) and **gamma motor neurons** (slow, involved in muscle tone and sensitivity to stretch). - **Afferent (Sensory) Neurons:** - **Pseudo-unipolar:** These neurons have a cell body located outside the CNS in ganglia, with a single axon splitting into two branches---one going to the sensory receptor and the other to the CNS. - **Varied Myelination:** Heavily myelinated axons (e.g., alpha and beta fibers) transmit faster, while **C fibers**, responsible for pain, are unmyelinated. **Motor Neurons & Muscle Interaction** Motor neurons innervate **skeletal muscle** (somatic effectors), which produce movement and help maintain muscle tone. These neurons form the **motor system** of the body. They can also supply smooth muscle (effectors of ANS) or cardiac muscle (myogenic). **Neuro-muscular Junction Anatomy** The **neuromuscular junction** is the site where motor neurons communicate with skeletal muscles. It is located at the **nerve entry point** of the muscle and is crucial for muscle contraction. At this site, acetylcholine (ACh) is released from the motor neuron to activate nicotinic receptors on the muscle, leading to contraction, its also the location of arterial supply and venous drainage of the muscle. **Myelination in the Somatic Nervous System** - **Myelinated Axons:** Most motor neurons and some sensory neurons are myelinated, which increases conduction speed. The thickness of the myelin sheath can vary. - **Schwann cells** myelinate axons in the PNS, while **oligodendrocytes** do so in the CNS. - Diseases like **Multiple Sclerosis**, **Guillain-Barré syndrome**, and **diabetic neuropathy** can damage the myelin, affecting nerve function. **Neuroglia in the PNS and CNS** - **PNS:** The main neuroglial cells are **Schwann cells**, which myelinate axons, and **satellite cells**, which provide structural support. Microglia have immune and inflammatory function. - **CNS:** **Oligodendrocytes** myelinate multiple axons in the CNS. **Nerve Sheaths** Nerves in both the PNS and CNS are covered by membranous sheaths: - **Epineurium:** Covers the entire nerve. - **Perineurium:** Encases nerve fascicles (bundles of axons). - **Endoneurium:** Surrounds individual axons, providing structural integrity and isolation. A diagram of the structure of the spinal cord Description automatically generated **L06 -- Synapses and Neurotransmitters** **Synapses and the Role of Neurotransmitters** A **synapse** is the site where communication occurs between two neurons or between a neuron and another cell (e.g., a muscle cell). The process involves neurotransmitters, which are chemical messengers that transmit signals across synapses. These neurotransmitters can either **excite** (increasing the likelihood of an action potential in the postsynaptic neuron) or **inhibit** (decreasing the likelihood of an action potential). **Key Concepts in Synaptic Communication** 1. **Excitatory Postsynaptic Potential (EPSP):** - When neurotransmitters bind to receptors on the postsynaptic membrane, they cause a small depolarization called an EPSP. - **Single EPSP:** A single EPSP might not be sufficient to trigger an action potential in the postsynaptic neuron. **Summation** (spatial or temporal) of multiple EPSPs is often necessary to reach the threshold for depolarization. 2. **Neuronal Specializations for Communication:** - **Dendrites:** Collect information from other neurons and help control the neuron's excitability. - **Axon Hillock:** The region where an action potential is generated. - **Axon Terminal:** Releases neurotransmitters into the synaptic cleft. **Types of Synapses** 1. **Electrical Synapse (Gap Junction):** - **Fastest and most primitive communication method** where cells are directly connected via gap junctions. - **Connexons** (protein channels) align to form a pore, allowing ions to flow between cells. This enables rapid, bidirectional communication. - Gap junctions are important in glia-neuron and glia-glia communication, and also in cardiac muscle for coordinated contraction. 2. **Chemical Synapse:** - **Uni-directional communication** where the signal travels from the presynaptic to the postsynaptic cell. - **Synaptic cleft** separates the two cells, and neurotransmitters released from vesicles in the presynaptic terminal bind to receptors on the postsynaptic membrane. **Critical Processes of Neurotransmission** 1. **Action Potential Initiation:** - The action potential (AP) begins in the cell body and propagates down the axon to the axon terminal, triggering the release of neurotransmitters. 2. **Calcium Influx:** - Depolarization at the nerve terminal opens **voltage-gated calcium (Ca²⁺) channels**, leading to calcium influx. 3. **Neurotransmitter Release:** - Vesicles containing neurotransmitters fuse with the presynaptic membrane at the **active zone** and release their contents via **exocytosis**. 4. **Neurotransmitter Binding:** - Neurotransmitters cross the synaptic cleft and bind to specific receptors on the postsynaptic membrane, leading to signal transmission. 5. **Termination of the Signal:** - The neurotransmitter must be quickly removed from the synaptic cleft to terminate the signal. This can be done via: - **Re-uptake:** The neurotransmitter is taken back into the presynaptic terminal via specific transporters. - **Enzymatic Breakdown:** Some neurotransmitters, like acetylcholine, are broken down in the synaptic cleft by enzymes. **Major Neurotransmitters** - **Acetylcholine (ACh):** Important at the neuromuscular junction (NMJ) for muscle contraction. - **Amino Acids:** - **Glutamate:** The most abundant excitatory neurotransmitter in the CNS. - **GABA (Gamma-Aminobutyric Acid):** The primary inhibitory neurotransmitter in the CNS. - **Glycine:** Another inhibitory neurotransmitter. - **Monoamines:** - **Noradrenaline, Dopamine, Serotonin (5-HT):** Involved in mood regulation, arousal, and reward systems. - **Neuroactive Peptides:** Over 100 kinds, including **opioid peptides** (endorphins, enkephalins) and **tachykinins** (e.g., Substance P). **Receptor Mechanisms** 1. **Ionotropic Receptors (Ligand-Gated Ion Channels):** - **Excitatory Synapse:** - An excitatory neurotransmitter binds to the receptor, causing a conformational change in an ion channel, allowing the influx of **Na⁺ ions** and depolarizing the postsynaptic neuron, resulting in an EPSP. - **Inhibitory Synapse:** - An inhibitory neurotransmitter causes the opening of **Cl⁻ channels**, leading to an influx of chloride ions and hyperpolarization (IPSP). 2. **Metabotropic Receptors (G-Protein Coupled Receptors):** - **Slower but longer-lasting effects** on cell excitability. - The binding of neurotransmitter activates a G-protein, which then triggers intracellular signaling pathways that can open or close ion channels or activate second messenger systems. **Synaptic Integration and Summation** - **Spatial Summation:** - Multiple presynaptic neurons stimulate different synapses on the same postsynaptic neuron. If the combined inputs reach the threshold, an action potential is generated. - Inputs closer to the **axon hillock** are more influential in reaching the threshold. - **Temporal Summation:** - A single synapse is activated repeatedly within a short period. If the EPSPs from successive activations sum up before the previous one decays, an action potential can be generated. - **Mixed Synapse:** - Inhibitory and excitatory inputs can occur simultaneously. If the excitatory and inhibitory signals are balanced, the membrane potential remains unchanged. **L08 -- Properties of Motor Units** **Overview of the Motor System** The motor system consists of motoneurons, skeletal muscles, the neuromuscular junction (NMJ), and connective tissues. These components work together to maintain posture, enable voluntary movement, stabilize joints, and generate heat through muscle contraction. - **Motoneurons** (neuronal tissue) transmit signals to muscles to initiate movement. - **Skeletal Muscles** (muscular tissue) contract to produce movement and maintain muscle tone. - **Neuromuscular Junction** is where motoneurons and muscle fibers communicate. - **Connective Tissue** (e.g., tendons, fascia) provides structural support. **Motor Unit Definition and Characteristics** A **motor unit** is the minimal functional unit of the motor system, consisting of: - A **somatic efferent (alpha-motoneuron)** and - All the **extrafusal muscle fibers** it innervates. The **innervation ratio** (number of muscle fibers innervated by a single motor neuron) varies across muscles: - **Extra-ocular muscles**: 1 motor neuron innervates \~10 fibers (precision required for eye movements). - **Quadriceps**: 1 motor neuron innervates up to 1000 fibers (strength for leg movement). The smaller the innervation ratio, the finer and more precise the control over movement. **Motor Neuron Pathways** - **Upper Motoneuron**: Originates in the brain and transmits signals to the lower motoneuron. - **Lower Motoneuron**: Located in the spinal cord (lamina IX) or brainstem, directly stimulates skeletal muscles to contract. **Muscle Structure and Function** - **Striated Muscle**: Myocytes are arranged in parallel to form muscle fibers. Striated muscles (e.g., skeletal and cardiac muscles) exhibit alternating light and dark bands due to the arrangement of **actin** (thin) and **myosin** (thick) filaments. - **Non-Striated Muscle**: Myocytes are arranged in various directions, found in smooth muscles, and are myogenic (generate their own electrical activity). **Types of Skeletal Muscles:** - **Convergent (Triangular)**: Muscle fibers converge to a tendon, allowing for strong contractions (e.g., pectoralis major). - **Circular (Sphincteric)**: Surround orifices (e.g., orbicularis oris). - **Parallel (Strap)**: Long muscles that allow large movements but offer lower strength (e.g., sternocleidomastoid). - **Fusiform**: Spindle-shaped, with a wider belly (e.g., biceps brachii). - **Pennate Muscles**: - **Unipennate**: Fibers run diagonally (e.g., lumbricals). - **Bipennate**: Fibers run in opposing diagonals (e.g., rectus femoris). - **Multipennate**: Multiple diagonal rows of fibers (e.g., deltoid). **Muscle Contraction Mechanism (Sliding Filament Model)** 1. **Neuromuscular Junction Activation**: An action potential from the motoneuron triggers the release of acetylcholine (ACh) into the synaptic cleft. 2. **Depolarization of Sarcolemma**: ACh binds to receptors on the sarcolemma, opening sodium channels and initiating depolarization. 3. **Calcium Release**: Depolarization spreads to the sarcoplasmic reticulum, releasing calcium ions. 4. **Actin-Myosin Interaction**: Calcium binds to **troponin**, which moves **tropomyosin** to expose actin-myosin binding sites. Myosin heads bind to actin, forming cross-bridges and initiating contraction. 5. **Power Stroke**: The myosin heads pivot, pulling actin filaments toward the center of the sarcomere, shortening the muscle. 6. **ATP Binding**: ATP causes myosin to detach from actin, hydrolyzing to ADP and phosphate, returning the myosin head to its original position for the next cycle. This cycle repeats rapidly, enabling sustained muscle contraction as long as calcium and ATP are available. **Motor Unit Twitch Characteristics** Muscle fibers within a motor unit share the same properties, such as contraction speed, fatigue resistance, and metabolic profile. There are three primary types of muscle fibers based on contraction characteristics: 1. **Fast Twitch (Type FF)**: High force, rapid contraction, quick fatigue (e.g., eye muscles). 2. **Intermediate Twitch (Type FR)**: Moderate speed and fatigue resistance (e.g., gastrocnemius). 3. **Slow Twitch (Type S)**: Slow contraction, high endurance, and low fatigue (e.g., soleus). **Twitch Summation**: If the frequency of stimulation increases, individual twitches blend into a **tetanus** (smooth, sustained contraction). **Motor Unit Recruitment and Properties** - Motor units can be recruited based on the force required for movement. **Smaller motor units** (with slow-twitch fibers) are recruited first, followed by **larger motor units** (with fast-twitch fibers) for greater force. - **Motor neurons** determine the type of muscle fibers they innervate. Changing the motor neuron will alter the properties of the muscle it controls, showing the importance of neuronal-muscle interaction. **Structure of Skeletal Muscles** - **Muscle Fibre (Myocyte)**: The basic unit of skeletal muscle, also known as a single muscle cell. - **Myofibrils**: The most abundant organelles within muscle fibres, responsible for contraction. - **Fascicle**: A bundle of myocytes. - **Muscle**: Formed by the collection of multiple fascicles. - **Neurovascular Bundle**: Contains blood vessels and nerves, running between and parallel to the fascicles. **Tissue Envelopes of Skeletal Fibres** - **Epimysium**: Connective tissue (CT) surrounding all fascicles, forming the outermost layer of the muscle. - **Perimysium**: CT surrounding each fascicle. - **Endomysium**: CT enveloping each individual muscle fibre (myocyte). **Importance of Tendons** - Tendons are tough bands of fibrous connective tissue. - They attach muscles to bones. - Act as points of confluence, consolidating individual myocyte contractions into a single action. - Facilitate movement, typically around joints. **L09 -- Neuromuscular Junction** **Overview of the Neuromuscular Junction (NMJ)** The neuromuscular junction is a specialized synapse between a motor neuron and a myocyte (muscle fibre), enabling muscle contraction. Several key features of the NMJ contribute to its efficient function: - **Multiple junctions** are formed along the muscle to ensure all fibers contract simultaneously. - It allows **rapid and reliable neurotransmission**, guaranteeing powerful muscle movement in response to each action potential. **Role of Calcium (Ca²⁺) in Excitation-Contraction Coupling** - Intracellular **Ca²⁺ concentration** is much higher than extracellular levels. - An **action potential** in the presynaptic neuron depolarizes the terminal, opening **voltage-gated Ca²⁺ channels**. - **Ca²⁺ influx** triggers vesicle fusion, leading to **neurotransmitter release** (e.g., acetylcholine, ACh). **Acetylcholine (ACh) and Nicotinic Receptors** - ACh is the neurotransmitter at the NMJ. - The **only receptor** on the postsynaptic membrane is the **Nicotinic Acetylcholine Receptor (nAChR)**. - This is a **ligand-gated ion channel** where two ACh molecules must bind for activation. - Once bound, **ion channels open**, allowing **Na⁺ influx** and **K⁺ efflux**. - The postsynaptic response is an **end-plate potential (EPP)**, which depolarizes the muscle membrane. **NMJ Structure** - **Presynaptic Terminal**: Contains many mitochondria and neurotransmitter vesicles, with **multiple active zones** for simultaneous neurotransmitter release. - **Postsynaptic Membrane**: Features **junctional folds** and high-density **nAChRs** and **voltage-gated Na⁺ channels**. **EPP vs. Action Potential (AP)** - **EPP**: Generated by ACh binding to **nAChRs** and causes a large depolarization of the motor end plate. It can trigger an **action potential** if it exceeds the threshold. - **Action Potential**: Once the threshold is reached, **voltage-gated Na⁺ channels** open, and an **action potential** spreads across the muscle fiber. **Fate of Acetylcholine (ACh)** - After ACh binds to **nAChRs**, it is rapidly **hydrolyzed** by **acetylcholinesterase (AChE)** into **choline** and **acetate**. - **Choline** is reabsorbed by the presynaptic neuron and used to synthesize more ACh. **Excitation-Contraction Coupling** 1. **Action potential** travels down the **T-tubules**. 2. **Dihydropyridine (DHP) receptors** in the T-tubules sense the action potential and activate **ryanodine receptors** in the **sarcoplasmic reticulum (SR)**. 3. This **opens calcium channels** in the SR, releasing **Ca²⁺** into the cytoplasm. 4. Ca²⁺ binds to **troponin**, causing the sliding filament mechanism and muscle contraction. **Muscle Contraction and Summation** - A **single action potential** in a motoneuron causes a **twitch**. - **Summation of twitches** occurs with high-frequency action potentials, leading to **tetanus**, a smooth, sustained contraction. **Myasthenia Gravis: A Disease Affecting the NMJ** - **Myasthenia Gravis** is an autoimmune disorder where the body produces antibodies against **nAChRs**, reducing their number and impairing synaptic transmission. - This results in **muscle weakness**, especially in muscles used for **ocular movements**, leading to symptoms like **drooping eyelids** and **vision problems**. - **Treatment**: **AChE inhibitors** (e.g., neostigmine) prolong the action of ACh at the NMJ, improving muscle function. **L10 -- Disorder of the Motor Unit** **Motor System of the Body** The motor system is composed of: 1. **Motoneurons (neuronal tissue)**: These neurons transmit commands from the brain to skeletal muscles. 2. **Skeletal Muscles (muscular tissue)**: These are the effectors that perform movement. 3. **Neuromuscular Junction (NMJ)**: The interface between motoneurons and muscle fibers, allowing for communication. 4. **Connective tissue**: Supports and stabilizes the structure. **Nerve-Muscle Unit** - The **nerve-muscle unit** is the simplest form of interaction between excitable tissues (nerve and muscle). - **Innervation** refers to the nerve supply to muscles. **Re-innervation** occurs when nerves regenerate to resupply muscles, although it may sometimes lead to unexpected results, like **crocodile tears syndrome** (incorrect re-innervation causes inappropriate responses such as crying while eating). - **Upper motor neurons (UMNs)** reside in the CNS, while **lower motor neurons (LMNs)** reside in the spinal cord or cranial nerve motor nuclei. **Motor Neuron Diseases (MND)** - MND is characterized by the **apoptosis** (programmed death) of motor neurons. - **Variants of MND**: - **Amyotrophic lateral sclerosis (ALS)**: Affects both upper and lower motor neurons. - **Progressive Supranuclear Palsy (PSP)**: Affects only upper motor neurons. - **Poliomyelitis**: Caused by the polio virus, which attacks LMNs, leading to paralysis. Damage to motor neurons leads to **denervation** (loss of innervation), causing muscle paralysis and atrophy. **Polio Myelitis** - Polio is caused by a viral infection that affects the **ventral horn** of the spinal cord, where lower motor neurons reside. - This damage leads to **denervation** of muscles, resulting in **paralysis**. The disease is preventable with vaccination but remains prevalent in developing countries. **Botulinum Toxin and the NMJ** - **Botulinum toxin**, often found in improperly preserved food, can cause botulism, leading to muscle paralysis. - It inhibits **acetylcholine (ACh)** release at the NMJ or can bind to **nAChRs**, preventing normal muscle activation. - **Botox** is a purified form of botulinum toxin used cosmetically to paralyze facial muscles and reduce wrinkles. **Nerve Gases and Organophosphates** - **Nerve gases** (organophosphates), used as chemical weapons, block **acetylcholinesterase**, preventing the breakdown of ACh at the NMJ, leading to muscle weakness and paralysis. - These gases also affect autonomic systems, overstimulating glands and causing excessive hormone secretion. **Myasthenia Gravis** - Myasthenia Gravis is a rare autoimmune disease where the immune system targets and destroys **nAChRs** on the postsynaptic membrane of the NMJ. - This causes **flaccid weakness** in affected muscles, such as those controlling eyelids or respiration. **Lower Motor Neuron (LMN) Signs** - **LMN disorders** result from damage to the motor neuron cell body, axons, or the NMJ. - Common signs of LMN damage include: - **Flaccid muscle weakness** (loss of motor tone) - **Hyporeflexia** or **areflexia** (diminished or absent reflexes) - **Muscle atrophy** (loss of muscle bulk) - **Fasciculations** (twitching of muscles) - **Muscle wasting** (from prolonged denervation) **Duchenne Muscular Dystrophy (DMD)** - **DMD** is a genetic disorder that affects muscle proteins, leading to progressive muscle weakness and atrophy. - Eventually, the disease causes death of muscle tissue, resulting in significant motor dysfunction. **L11 -- Uses and Actions of Local Anaethetics** **Why Use Local Anaesthetics?** - Local anaesthetics (LAs) are used to **reduce pain** in a targeted area of the body without affecting consciousness. - Common examples include pain management for dental procedures, surgery, or other minor medical interventions. **Definition of a Local Anaesthetic** - **Local Anaesthetics**: Reversibly block nerve conduction when applied to a specific area of the body to enable procedures to be carried out without loss of consciousness. - **Reversible**: The anaesthesia wears off after the procedure, unlike general anaesthesia, which affects the entire body. - **Contrast to General Anaesthesia**: Unlike general anaesthesia, which causes unconsciousness, local anaesthesia only affects the targeted area. **How Do Local Anaesthetics Prevent Pain?** - **Nociceptors**: Detect pain signals from stimuli, triggering action potentials (APs) that are transmitted to the brain. - **Action Mechanism**: LAs block the **voltage-gated sodium (Na+) channels**, preventing action potentials from propagating to the brain and thus stopping the sensation of pain. **Naming Convention of Local Anaesthetics** - All local anaesthetics end in "-caine" as a reference to the first local anaesthetic, **cocaine**. - **Cocaine**: No longer used in clinical practice due to psychoactive effects. - **Synthetic versions** like **procaine** were developed to avoid these side effects. **Chemical Nature of Local Anaesthetics** - **Common Structure**: Local anaesthetics (e.g., lidocaine, bupivacaine, procaine) share a common structure with similar functional groups. - **Aromatic ring**: Lipophilic (fat-soluble), allowing the drug to cross the cell membranes of neurons. - **Linkage**: **Amide** or **Ester**. - **Amide linkage**: Metabolized slowly, commonly used in clinical practice. - **Ester linkage**: Metabolized quickly but can cause allergic reactions (e.g., procaine). - **Amine group**: Can be neutral or positively charged, affecting solubility (charged molecules are hydrophilic and unable to cross cell membranes easily). **Mechanism of Action** - **Equilibrium**: Local anaesthetics exist in an equilibrium of ionized (charged) and non-ionized (uncharged) forms. - **Ionized form**: Hydrophilic and unable to pass through the lipid membrane. - **Unionized form**: Lipophilic and can pass through the membrane to reach the axon. - **Action**: - **Unionized form** crosses the membrane. - Inside the axon, it shifts to the ionized form. - The **ionized form** blocks the voltage-gated Na+ channels, preventing depolarization and halting action potential propagation. **Factors Affecting Effectiveness of Local Anaesthetics** - **Inflammation/Infection**: Bacteria produce acidic byproducts, leading to acidosis and lower pH in infected areas. This shifts the LA equilibrium towards the ionized form, reducing LA efficacy since only the unionized form can cross membranes. - **Antibiotics**: Often prescribed to treat infection before using LA to improve effectiveness. - **Differential Sensitivity of Neurons**: Smaller **sensory neurons** are more sensitive to LAs compared to **motor neurons**, allowing for more targeted anaesthesia. **Routes of Administration** - **Topical Anaesthesia**: Applied directly to the skin or mucous membranes, effective only on thin or accessible tissues. - **Infiltration Anaesthesia**: Injected directly into the tissue (e.g., dental procedures). It bypasses the skin barrier. - **Nerve Block Anaesthesia**: Injection near a nerve affects a large area (e.g., jaw or limb). - **Epidural Anaesthesia**: Administered in the epidural space near the spinal cord for procedures like childbirth. - **Spinal Anaesthesia**: Injected into the subarachnoid space around the spinal cord for more extensive body areas. - **Regional Anaesthesia (Bier's Block)**: Administered intravenously, using a tourniquet to isolate an extremity (e.g., arm or leg). **Side Effects** - **Non-specific reactions**: Allergic responses or redness due to preservatives in the anaesthetic. - **Specific reactions (due to Na+ blockade)**: - **High doses** or **improper administration** can lead to systemic effects, including: - **CNS effects**: Tremors, convulsions, respiratory failure. - **Cardiovascular effects**: Decreased heart contraction and blood pressure, arrhythmias. - **Cardiotoxicity**: **Bupivacaine** is particularly cardiotoxic. **Drugs Used with Local Anaesthetics** - **Vasoconstrictors**: Added to LAs to prevent dilation of blood vessels, which can spread the drug too quickly and reduce its effectiveness. - **Adrenaline/Felypressin**: Common vasoconstrictors used to prolong the anaesthetic effect and limit side effects by keeping the drug localized. - **Caution**: Careful when administering LAs in extremities (e.g., fingers or toes) since vasoconstriction can impede blood flow to these areas. **Properties of Good Local Anaesthetics** - **Reversible**: The anaesthetic effect wears off after the procedure. - **Effective for the duration of the procedure**: The appropriate choice of LA depends on how long the anaesthesia is required. - **Low toxicity**: Safe for use without causing significant side effects when administered properly. **L12 -- Degeneration and Regeneration of Peripheral Nerves** **Functional Expression of the Nervous System** - The nervous system conveys its actions through **three key permutations**: - **Neurones supply**: - **Skeletal muscles** -- via the somatic nervous system - **Smooth muscles** -- via the autonomic nervous system - **Glands** -- via the autonomic nervous system - **Sensory signals to the brain** -- via afferents originating in the periphery and special sense organs - **Descending Pathways**: These relay chains of neurons allow communication from the brain to effectors (muscles, glands) through somatic or autonomic pathways. **Causes for Nerve Degeneration** - **Genetics**: E.g., **motor neurone disease** - **Aging**: A natural process leading to nerve function decline. - **Acquired Causes**: - Trauma or injury - Toxic infections affecting nerves (e.g., **multiple sclerosis**) **Definitions of Nerve Damage** - **Disturbance to Neuron or its Environment**: Disruption can cause dysfunction, the extent of which depends on the nature and severity of the insult. - **Types of Nerve Damage** (Seddon's Classification): - **Superficial**: Damage to the **epineurium** (outermost layer of the nerve). - **Intermediate**: Damage to the **perineurium** (surrounds nerve fascicles). - **Deep**: Damage to the **endoneurium** and axons. **Types of Nerve Injury (Axonal Damage)** - **Neuropraxia**: - Temporary loss of neuron function, usually due to damage to the **myelin sheath** (e.g., compression). - **Full recovery** expected. - **Axonotmesis**: - Damage to the axon; the axon may split but the surrounding layers remain intact. - **Reinnervation** is possible if the axonal layers (endoneurium, perineurium, epineurium) remain intact. - **Neurotmesis**: - Complete transection (cut) of the axon and its surrounding layers. - **Irrecoverable** and the most severe form of nerve damage. **Reactions to Injury in Axonotmesis** - **Neuronal Response**: - **Proximal Segment**: Remains attached to the cell body and receives support. - **Distal Segment**: Detached from the cell body, loses support, and undergoes degeneration via **phagocytosis** by glial cells. - **Distal Segment Fate**: - **Immediate Response**: Stops conducting action potentials, begins swelling and seals itself. - **Within Hours**: Synaptic terminals degenerate and are cleaned up by **astrocytes**. - **Wallerian Degeneration**: The axon and myelin sheath break down in the distal segment, with phagocytes cleaning up the debris. - **Proximal Segment Fate**: - **Immediately After Injury**: Stops conducting action potentials, and leaks intracellular fluid. - **Days After Injury**: - **Chromatolysis**: Nissl bodies displaced; the cell body starts to synthesize proteins actively for repair, nucleus moves to periphery of cell body. - **Axon Regrowth**: Axon begins to grow sprouts toward the target organ. **Regeneration After Axonotmesis** - **Schwann Cells\' Role**: - **Macrophages** release mitogens that trigger Schwann cells to repopulate. - Schwann cells synthesize **laminin**, guiding regenerating axons. - **Nerve Growth Factor**: Released by Schwann cells, promoting distal axon growth. - **Guidance Structures**: The **endo-, peri-, and epineurium** provide physical and chemical guidance for nerve sprouts. **Reaction of Skeletal Muscle** - **Lower Motor Neuron Damage**: Causes **denervation** of the skeletal muscle. - **Denervation**: Muscle loses its nerve supply and becomes paralyzed. - **Reinnervation**: Axons attempt to regrow and reinnervate the muscle. Success varies. - **Muscle Type Switching**: - **Slow (S) and Fast (FF/FR) Muscles** are innervated by different motor neurons. - If a motor neuron is damaged and reinnervates a muscle of a different type, the muscle fiber switches its phenotype. - Example: A **Type S motoneuron** incorrectly innervates a **Type FF muscle fiber**, causing it to switch to **Type S** fiber. **Phases of Muscle Damage** 1. **Acute Phase** (if muscle is not reinnervated): - Muscle is **paralyzed** and **areflexic** (no reflexes). - Muscle becomes **atonic** (relaxed). - **Fasciculation** (involuntary contractions) occurs. 2. **Chronic Phase** (if muscle remains denervated): - **Fasciculations cease**. - **Denervation atrophy**: Muscle loses bulk and begins to break down. - Muscle undergoes **fibrosis**, and the muscle tissue is replaced by connective tissue (e.g., adipose tissue). **Rhabdomyolysis** - A life-threatening condition caused by the breakdown of skeletal muscle, releasing intracellular components into the bloodstream, leading to **acute renal failure**. - **Causes**: - Trauma (e.g., vehicle accidents) - **Over-exercise** or **injection of harmful substances**. - Pathological muscle damage. **L13 -- Simple Reflexes** **Simple Reflexes** Reflexes are **quick, involuntary actions** that occur as a response to a stimulus. They are essential for protecting the body, maintaining posture, and regulating physiological processes. Reflexes can be classified based on their involvement with the **somatic (voluntary)** or **autonomic (involuntary)** nervous systems. Reflexes can also be voluntary but are typically **involuntary**. **Components of a Reflex Arc** A reflex involves a specific neural pathway called the **reflex arc**, which includes five components: 1. **Sensory receptor** -- Detects the stimulus. 2. **Afferent limb (sensory neuron axons)** -- Carries the information to the CNS. 3. **Central component** -- Consists of interneurons or synapses, often in the spinal cord or brainstem (and in the gut for the enteric nervous system). 4. **Efferent limb** -- Carries the motor response to the effector organ. 5. **Effector** -- The organ that performs the response (skeletal muscle, smooth muscle, or glands). **Functions of Reflexes** Reflexes serve several critical functions: - **Protective**: E.g., limb withdrawal from painful stimuli, cough reflex, skeletal muscle reflexes to prevent injury. - **Coordinated activity**: E.g., postural control during walking or writing. - **Homeostasis**: E.g., regulation of blood pressure. **Simple Stretch Reflexes (Myotatic Reflexes)** **Stretch reflexes** are fundamental in maintaining **posture** and **muscle tone** by adjusting the degree of muscle contraction in response to changes in muscle length. - **Proprioceptors** (e.g., muscle spindles and Golgi tendon organs) detect these changes. **Muscle spindle** -- A specialized sensory receptor within muscles that detects stretch. If the sensory neuron synapses directly with the motor neuron, this is known as a **monosynaptic reflex** (single synapse). **Mechanism of the Simple Stretch Reflex (via Muscle Spindle)** 1. **Muscle Stretch**: The muscle is stretched (e.g., by a weight), which elongates the muscle spindle. 2. **Action Potential**: The stimulus generates an action potential if it reaches the threshold. 3. **Synapse**: The sensory neuron releases an excitatory neurotransmitter at the synapse. 4. **Motor Neuron Excitation**: The motor neuron becomes excited, and action potentials travel along the motor neuron to the muscle. 5. **Contraction**: The motor neuron releases **acetylcholine (ACh)** at the neuromuscular junction (NMJ), causing the muscle to contract. 6. **Prevention of Damage**: This contraction prevents damage to the muscle by resisting the stretch, for instance, when lifting heavy objects. **Excitation vs Inhibition of Motoneurons** - **Excitation**: Achieved by the release of **excitatory neurotransmitters** like ACh, leading to an action potential in the motoneuron. - **Inhibition**: Achieved by the release of **inhibitory neurotransmitters** like **GABA**, which prevents action potentials in the motoneuron. **Skeletal Muscle Reflexes** - **Stretch Reflex**: The **stretch reflex** is a motor response triggered by the stretching of a muscle, which is detected by the muscle spindles. This reflex involves: - **Contraction of the agonist muscle(s)** (via excitatory interneurons). - **Inhibition of antagonist muscle(s)** (via inhibitory interneurons). **Example**: The **knee jerk reflex**: - Striking the patellar tendon stretches the quadriceps muscle. - This is detected by the muscle spindles and sends a signal down the reflex arc. - **Quadriceps contraction** (kick) via motor neurons. - **Hamstring inhibition** (antagonistic muscle relaxation). **Mechanism of the Knee Jerk Reflex** 1. **Stimulus**: Tapping the patellar tendon stretches the quadriceps muscle. 2. **Action Potential**: The stretch activates the muscle spindle and sends an action potential to the spinal cord. 3. **Excitation**: The sensory neuron releases **ACh**, exciting the motor neuron to contract the quadriceps. 4. **Inhibition**: The sensory neuron also excites an **interneuron**, which releases an **inhibitory neurotransmitter** to prevent motor neurons from firing, allowing the hamstrings to relax. 5. **Result**: This coordinated response allows for knee extension and is a classic example of the **myotatic reflex**. **Golgi Tendon Reflex** The **Golgi tendon reflex** involves the detection of tension in the tendon and muscle. It aims to protect the muscle from excessive tension or tearing. - **Golgi tendon organs (GTOs)** detect the increased tension on the muscle. - The reflex involves: - **Inhibition of the agonist muscle** (via inhibitory interneurons). - **Excitation of the antagonist muscle** (via excitatory interneurons). **Mechanism of the Golgi Tendon Reflex** 1. **Tension**: The muscle contracts (e.g., when lifting a heavy weight). 2. **Activation**: The increased tension in the tendon activates the **Golgi tendon organ (GTO)**. 3. **Action Potential**: The GTO sends an action potential to the spinal cord. 4. **Inhibition of Agonist**: The sensory neuron releases excitatory neurotransmitters, activating an inhibitory interneuron. This interneuron inhibits motor neurons to decrease muscle activity and prevent damage. 5. **Excitation of Antagonist**: Simultaneously, the antagonist muscle is activated via excitation of its motor neurons. This is known as the **reverse myotatic reflex** -- initially, more muscle activity leads to less muscle activity to protect against injury. **Withdrawal and Crossed-Extensor Reflexes** The **withdrawal reflex** helps the body withdraw from a painful stimulus (e.g., stepping on something sharp). The **crossed-extensor reflex** compensates for this withdrawal by activating muscles on the opposite side of the body. **Mechanism of the Withdrawal Reflex** 1. **Painful Stimulus**: Nociceptors detect the painful stimulus (e.g., stepping on a sharp object). 2. **Signal Transmission**: The sensory neuron sends an action potential to the spinal cord. 3. **Excitatory Interneurons**: The sensory neuron synapses with excitatory interneurons, which activate motor neurons. 4. **Motor Response**: The motor neurons stimulate the contraction of flexor muscles, withdrawing the limb from the painful stimulus. **Mechanism of the Crossed-Extensor Reflex** 1. **Afferent Signal**: The sensory neuron for the painful stimulus crosses to the opposite side of the spinal cord. 2. **Activation of Contralateral Extensors**: Excitatory interneurons activate the motor neurons to contract the contralateral extensor muscles (e.g., quadriceps). 3. **Inhibition of Contralateral Flexors**: Inhibitory interneurons prevent contraction of the contralateral flexor muscles (e.g., hamstrings). 4. **Stabilization**: This allows the contralateral leg to support the body weight while the injured leg is withdrawn. **Summary of Reflexes** - **All of the reflexes discussed are somatic reflexes** (involving voluntary muscles). - Reflexes can involve: - **Muscle spindles** for stretch reflexes. - **Golgi tendon organs** for detecting excessive tension. - **Withdrawal reflexes** to protect the body from pain. - **Crossed-extensor reflexes** for stabilization when one limb is withdrawn. Each reflex pathway serves the crucial functions of **protecting the body**, **maintaining posture**, and ensuring the **homeostasis** of critical processes. **L14 -- Anatomy of the ANS** **1. Introduction to the Autonomic Nervous System (ANS)** The **ANS** is a critical component of the **visceral nervous system** and functions autonomously to regulate vital physiological processes. It involves **efferent** pathways (motor), **afferent** pathways (sensory), and the **enteric nervous system (ENS)**. The ANS is responsible for **involuntary control** of functions like heart rate, digestion, and glandular activity. It has a two-neuron pathway composed of **preganglionic** and **postganglionic** neurons. - **Sympathetic Division**: Typically associated with the **\"fight-or-flight\"** response. Preganglionic neurons are located in the brainstem and spinal cord. - **Parasympathetic Division**: Associated with **\"rest-and-digest\"** functions. Also uses brainstem and spinal cord as origins for preganglionic neurons. - **Enteric Nervous System (ENS)**: A more localized system that governs gastrointestinal (GI) function autonomously but communicates with the CNS. **2. Divisions of the ANS** - **Sympathetic Nervous System (SNS)**: - Prepares the body for stress or danger (e.g., increased heart rate, dilated bronchi, inhibition of digestion). - **Parasympathetic Nervous System (PNS)**: - Dominates during rest and recovery, promoting processes like digestion and relaxation (e.g., decreased heart rate, increased digestion). - **Enteric Nervous System (ENS)**: - Controls gut motility and secretion, operating mostly independently but with some communication to the CNS. **3. Structural Overview** - **Two-Neuron Pathway**: - **Preganglionic Neurons**: Originate from the CNS. - **Postganglionic Neurons**: Located in ganglia and innervate target organs. - **Neurotransmitters**: - **Sympathetic**: ACh (preganglionic), **Norepinephrine** (postganglionic, except for sweat glands which use ACh). - **Parasympathetic**: ACh for both preganglionic and postganglionic neurons. **4. Clinical Relevance** - **Horner\'s Syndrome**: Results from damage to the sympathetic chain, leading to: - **Ptosis** (drooping eyelid) - **Miosis** (constricted pupil) - **Anhidrosis** (lack of sweating) This condition can result from trauma, tumors, or lesions that affect the sympathetic pathway. **5. Sympathetic Nervous System (SNS)** - **Functions**: - **Fight-or-Flight Response**: Increases heart rate and blood pressure, dilates pupils, inhibits digestion, and stimulates adrenaline secretion. - **Anatomy**: - Preganglionic neurons arise from the **lateral horn** of the **thoracolumbar** spinal segments (**T1-L2**). - Postganglionic fibers synapse in the **sympathetic chain** or **pre-aortic ganglia**, releasing **norepinephrine**. - **Ganglia**: - Sympathetic chain ganglia, found near the vertebral column, and pre-aortic ganglia, which serve abdominal and pelvic organs. - **Clinical Note**: - **Horner\'s Syndrome** occurs due to sympathetic chain damage, causing ptosis, miosis, and anhidrosis. **6. Parasympathetic Nervous System (PNS)** - **Functions**: - **Rest-and-Digest**: Slows heart rate, promotes digestion, and facilitates activities like defecation and erection. - **Anatomy**: - Preganglionic neurons originate from **cranial nerves III, VII, IX, X** and **sacral segments S2-S4**. - Postganglionic neurons are located near or within target organs, with **acetylcholine** (ACh) as the primary neurotransmitter. - **Ganglia**: - Includes the **ciliary**, **pterygopalatine**, **submandibular**, and **otic ganglia**. **7. Enteric Nervous System (ENS)** - **Functions**: - Regulates motility and secretion within the **gastrointestinal tract (GIT)**. - **Key Plexuses**: - **Myenteric Plexus**: Controls peristalsis. - **Submucosal Plexus**: Oversees glandular secretion. - **Pathology**: - **Hirschsprung\'s Disease**: Absence of ENS neurons in the rectum, leading to constipation due to a lack of peristalsis. **8. Comparison of Sympathetic vs. Parasympathetic** **Feature** **Sympathetic** **Parasympathetic** ------------------------- ------------------------------------- --------------------------------------------- **Origin** Thoracolumbar (T1-L2) Craniosacral (CN III, VII, IX, X; S2-S4) **Preganglionic Fiber** Short Long **Ganglion Location** Close to CNS Near or within target organs **Neurotransmitter** **Norepinephrine** (postganglionic) **Acetylcholine** (pre- and postganglionic) **Function** Fight-or-Flight Rest-and-Digest **L15 -- Overview of Autonomic Function** **Types of Receptors** **Visceral Receptors** - Not consciously perceived. - Responsible for internal monitoring (e.g., blood pressure monitoring by baroreceptors). **Somatosensory Receptors** - Consciously perceived. - Responsible for external monitoring (e.g., vision, touch, hearing, proprioception). - Input from these receptors can influence both somatic and autonomic responses. **Example:** - **Cold response:** - ANS: Vasoconstriction of skin blood vessels. - Somatic: Shivering of skeletal muscles. - **Viewing nearby objects:** - ANS: Adjusts size of lens and pupils. - Somatic: Moves eyes to focus on the object. **Visceral Referred Pain** - Some visceral afferent input is not consciously perceived (e.g., baroreception), but others are. - Some visceral afferent axons from organs (e.g., heart, stomach) synapse with somatic sensory neurones in the spinal cord. - Pain is perceived in the dermatome associated with the spinal cord segment that receives the input (e.g., T1-T4 dermatome for heart). - This is referred pain, and it can present in different regions depending on the organ. **Differences Between Somatic and Autonomic Motor Neurones** **Type of Effector** - **Somatic:** Innervates only skeletal muscle. - **Autonomic:** Innervates smooth muscle, cardiac muscle, and glands. **Number of Neurones** - **Somatic:** One long axon from the CNS innervates the effector, providing precise control. - **Autonomic:** Consists of preganglionic and postganglionic neurones, with multiple postganglionic neurones for the same preganglionic neurone. This results in a widespread and synchronized response. **Exception:** - Chromaffin cells of the adrenal medulla are innervated by preganglionic sympathetic axons to release adrenaline. **Type of Neurotransmitter Used** - **Somatic:** Ach (acetylcholine) at neuromuscular junctions. - **Sympathetic:** Ach in preganglionic neurones, NA (noradrenaline) in postganglionic neurones. - **Parasympathetic:** Ach in both preganglionic and postganglionic neurones. **Note:** - **Anticholinergic drugs** target the entire autonomic nervous system (ANS). - **Anti-noradrenergic drugs** target only the sympathetic nervous system (SyNS). - Exception: Sweat glands (SNS) use Ach in both preganglionic and postganglionic neurones. **Type of Neurotransmitter Release** - **Somatic:** Discrete release of neurotransmitter at the neuromuscular junction, allowing precise muscle contraction. - **Autonomic:** Diffuse release of neurotransmitter, with axons branching and forming varicosities that release neurotransmitter across a wide area. **Integration of PNS (Parasympathetic Nervous System) and SNS (Sympathetic Nervous System)** **Antagonistic, Same-Effector Dual Innervation** - **Effector:** A single organ or tissue is innervated by both the PNS and SNS. - The balance between PNS and SNS activity determines the response. **Examples:** - **Cardiac Muscle:** - Increased PNS activity: Reduces heart rate. - Increased SNS activity: Increases heart rate. - **Smooth Muscle of the GIT:** - Increased PNS activity: Increases motility. - Increased SNS activity: Decreases motility. - **Blood Vessels (head, neck, reproductive organs):** - PNS input: Vasodilation. - SNS input: Vasoconstriction. **Antagonistic, Different-Effector Dual Innervation** - The PNS and SNS affect different muscles to produce opposite effects. **Example:** - **Pupil Diameter:** - PNS: Activates circular sphincter pupillae (constricts pupil). - SNS: Activates radial dilator pupillae (dilates pupil). **Control of Pupil Diameter:** - PNS preganglionic neurones involved in the pupillary light reflex originate from the **Edinger-Westphal nucleus** and synapse in the **ciliary ganglion**. **Single-Input Innervation** - **Sweat Glands:** Only SNS input. - **Lens Adjustment (Ciliary Muscles):** Only PNS input. - PNS causes ciliary muscle contraction to make the lens more spherical for near vision. - **Blood Vessels (Other than head, neck, reproductive organs):** Only SNS input. - SNS causes vasoconstriction, and reduced SNS activity causes vasodilation. **Non-Antagonistic Dual Innervation** - Both the PNS and SNS affect the effector in a similar manner. **Example:** - **Salivary Glands:** - SNS: Causes vasoconstriction of blood vessels to the glands, leading to thick, sticky saliva. - PNS: Causes vasodilation and promotes fluid secretion. - **Penis:** - PNS: Stimulates erection. - SNS: Stimulates ejaculation. - PNS activity must precede SNS activity for proper function. **Vasodilation of Skeletal Muscle Blood Vessels** - The SNS causes vasodilation of blood vessels supplying skeletal muscles during activity. - **Adrenaline**, released by the adrenal medulla in response to SNS activation, binds to **beta-receptors** on smooth muscle in skeletal muscle blood vessels, causing vasodilation. **Adrenergic Receptors:** - Smooth muscle in blood vessels supplying skeletal muscles has more **beta-receptors**, which dilate blood vessels in response to SNS activation. - Other blood vessels have **alpha-receptors** that, when activated by **noradrenaline (NA)**, cause vasoconstriction. **Non-Innervated Receptors:** - These adrenergic receptors are distant from nerve terminals and activated by circulating **NA** and **adrenaline**, affecting smooth muscles in the bronchioles and some blood vessels. **L16 -- The Enteric Nervous System** **Enteric Nervous System (ENS) Overview** - The ENS is an intrinsic division of the visceral nervous system. - It innervates the **GIT (gastrointestinal tract)**, **pancreas**, and **biliary system** and controls important functions. - **Intrinsic**: The ENS can function independently but also communicates with the **CNS** for certain functions. **Main Plexuses:** 1. **Myenteric Plexus**: Found between the circular and longitudinal layers of the muscularis externa, spanning the entire length of the GIT (from esophagus to anus). It coordinates **motility** (e.g., peristalsis). 2. **Submucosal Plexus**: Found in the inner layers of the stomach and small intestine. It controls **glandular secretion**. **Components of the Enteric Nervous System** - **Sensory (Afferent) Neurones**: - Associated with **mechanoreceptors** (detect physical presence of food) and **chemoreceptors** (detect chemicals released by food). - **Interneurones**: - **Excitatory** or **inhibitory** neurons that coordinate responses within the ENS. - **Secretomotor (Efferent) Neurones**: - Innervate **smooth muscles** and **secretory glands**. - Can be **excitatory** or **inhibitory** and use **ACh** or various **peptides** as neurotransmitters. **Enteric Reflexes** **1. Motility Reflex (Peristalsis)** - **Purpose**: Rhythmic movement of food along the GIT. - **Mechanism**: - **Afferent Neurones**: Detect food via mechanoreceptors (stretch) and chemoreceptors (chemical signals). - **Excitatory Interneurones**: Activate motor neurones in smooth muscle **behind** the food bolus, stimulating contraction. - **Inhibitory Interneurones**: Relax smooth muscle **ahead** of the bolus, facilitating movement. - This coordinated contraction and relaxation of GIT segments create **peristalsis**, pushing food along the digestive tract. **2. Secretory Reflex** - **Purpose**: Release of digestive enzymes and other chemicals to aid in digestion. - **Mechanism**: - **Afferent Neurones** detect food presence via mechanoreceptors and chemoreceptors. - **Interneurones** activate **secretomotor neurones**, which stimulate exocrine glands to release enzymes, acids, and mucus into the stomach and small intestine. **3. Blood Flow Reflex** - **Purpose**: Increase blood flow to the GIT to facilitate digestion. - **Mechanism**: - **Afferent Neurones** detect food presence. - **Motor Neurones** release vasoactive neurotransmitters, dilating blood vessels supplying the GIT. **Integration with the CNS** - **Autonomic Afferents**: Deliver sensory information from the ENS to the CNS, making us consciously aware of food in the stomach. - **CNS Control**: - **Sympathetic Efferents**: Inhibit peristalsis (e.g., during fight-or-flight, blood is redirected away from the GIT). - **Parasympathetic Efferents**: Support normal digestive function and motility. - **Voluntary Control**: CNS controls the **anal sphincters**, enabling conscious control of defecation. **Enteric Nervous System Disorders** **ENS-Specific Disorders:** 1. **Chaga's Disease**: - Caused by a parasite transmitted by insect bites. - The parasite produces a toxin that damages ENS neurons, impairing digestive function. 2. **Achalasia**: - Damage to ENS neurones in the mouth and esophagus, causing swallowing difficulties. - Typically occurs due to loss of inhibitory neurons in the esophagus. 3. **Hirschsprung's Disease**: - Congenital absence of ENS neurons, usually in the distal colon. - Leads to **paralysis of smooth muscle**, causing constipation, bowel obstruction, and inflammation due to faecal buildup. **General Neurological Disorders Affecting the ENS:** 1. **Parkinson's Disease**: - Pathogens may travel through the GIT to the brain via enteric neurons, causing neuron degeneration in the brain. 2. **Peripheral Neuropathies**: - Affects gut function, leading to various digestive issues. 3. **Myasthenia Gravis**: - Autoimmune disorder that can affect the ENS by producing antibodies against **ACh receptors**, disrupting smooth muscle function in the GIT. **Disorders of the Gut-Brain Axis:** 1. **IBS (Irritable Bowel Syndrome)**: - Dysfunctional interaction between the ENS and brain, often associated with anxiety. - Symptoms include abdominal pain, bloating, changes in bowel habits (e.g., diarrhea or constipation), and excessive gas. **Drugs Targeting the ENS** - **Opioids**: The ENS uses opioids as neurotransmitters, and some drugs may target opioid receptors in the GIT. - **Anti-diarrheal drugs** (e.g., **loperamide**) target opioid receptors to reduce motility. - **Pain medications** (e.g., **morphine**, **codeine**) can activate opioid receptors in the GIT, reducing gut activity and potentially causing constipation as a side effect. **L17 -- Autonomic Pharmacology** **Drugs Targeting the Entire Autonomic Nervous System** - Both the **sympathetic** and **parasympathetic** nervous systems use **nicotinic ACh receptors** within their ganglia to transmit the action potential between preganglionic and postganglionic neurons. - **N** = nicotinic receptor - **M** = muscarinic receptors Nicotinic ACh receptors are also present on **skeletal muscles**. However, the structure of the nicotinic ACh receptors of skeletal muscle is different from that in the ANS. - Generally, a nicotinic AChR is a **pentameric transmembrane ion channel** with 5 subunits. - In skeletal muscle, the AChR uses **α1** subunits. - In the ANS, nicotinic AChRs use **α2-7** subunits. Drugs can be used to specifically target the subunits of ANS receptors. - **Hexamethonium** is a non-competitive antagonist of ANS NAChRs. It blocks all the effects of autonomic stimulation (both sympathetic and parasympathetic) and has **anti-hypertensive effects**. - It is no longer used due to its many side effects, as it blocks all aspects of sympathetic and parasympathetic transmission. **Drugs Targeting the Parasympathetic Nervous System** To specifically target the **parasympathetic nervous system (PaNS)**, a drug must exploit the fact that the post-ganglionic neurons of the PaNS exert their effects on effectors via **muscarinic ACh receptors**. - **Muscarinic ACh receptors** are **G-protein coupled receptors** with 7 transmembrane domains. There are 5 types of muscarinic receptors: - **M1** -- present in the stomach and salivary glands. It is a **Gq receptor**, achieving its effect by activating **phospholipase C (PLC)**. - **M2** -- present in cardiac muscle. It is a **Gi receptor**, achieving its effect by inhibiting **adenylyl cyclase**, reducing **cAMP** levels. - **M3** -- present in smooth muscle (e.g., in eyes, bronchi) and exocrine glands. It is a **Gq receptor**, activating **PLC**. **Muscarinic Agonists (Parasympathomimetic Drugs)** These drugs activate the muscarinic ACh receptors and achieve parasympathetic effects such as: - **Decreased heart rate** - **Vasodilation** - **Smooth muscle contraction** (e.g., in the gut or respiratory tract) - **Salivation** - **Lacrimation** (tearing up) - **Bronchial mucous secretions** **Muscarine poisoning** can occur from overconsumption of muscarine-containing mushrooms, causing extreme parasympathetic effects, such as: - **Bradycardia** - **Hypotension** - **Increased gut motility and pain** - **Bronchoconstriction** - **Miosis** (abnormal constriction of pupils) **Atropine**, a competitive antagonist for muscarine, can reverse this poisoning. - Example: **Pilocarpine** is used to treat **glaucoma** by topical administration. It targets only the **M3 receptors** in the ciliary muscles, preventing unwanted side effects. **Muscarinic Antagonists** Muscarinic antagonists are used to treat various conditions, including: - **Asthma** (e.g., **ipratropium/tiotropium**) -- relax smooth muscles of bronchi to widen the airway. - **Bradycardia** (e.g., **atropine**) -- speeds up heart rate. - **Constricted pupils** (e.g., **tropicamide**) -- relaxes iris sphincter muscle to dilate the pupil. - **Urinary incontinence** (e.g., **oxybutynin/tolterodine**) -- relaxes smooth muscle of the bladder. - **Motion sickness** (e.g., **hyoscine**) - **Cyclopentolate** -- dilates the pupil for diagnostic procedures. They are also used to counteract the effect of **ACh esterase inhibitors** administered after operations to stop the paralysis induced by anesthesia, which causes ACh to build up in synaptic clefts. - **AChE inhibitors** can treat **myasthenia gravis** or be used as weapons (nerve gas), mimicking parasympathetic activation. **Atropine** is used to treat nerve gas poisoning. **Drugs Targeting the Sympathetic Nervous System** To target the **sympathetic nervous system (SyNS)**, a drug must exploit the fact that post-ganglionic neurons of the SyNS use **noradrenaline (NA)** as their neurotransmitter. These drugs can be **adrenergic agonists** or **adrenergic antagonists**, categorized by the type of adrenoceptor they act on. **Adrenoceptor Types** Noradrenaline acts on 5 types of **adrenoceptors**, which are **G-protein coupled receptors**: - **α1** -- Causes smooth muscle contraction (e.g., vasoconstriction). It is a **Gq receptor**. - **α2** -- Causes direct vasoconstriction, inhibition of NA release, and sympathetic outflow from the CNS. It is a **Gi receptor**. - **β1** -- Increases heart rate and contractility. It is a **Gs receptor**, activating **adenylyl cyclase** and increasing cellular **cAMP**. - **β2** -- Causes smooth muscle relaxation (e.g., in bronchi and blood vessels of skeletal muscle). It is a **Gs receptor**. - **β3** -- Causes smooth muscle relaxation (e.g., in the bladder) and stimulates **lipolysis**. It is a **Gs receptor**. **Uses of α-Adrenoceptor Agonists** - **Vasoconstrictors** (e.g., **adrenaline, noradrenaline**) -- Used with local anesthetics to prevent their spread and prolong action. Target **α1 receptors**. - **Nasal decongestants** (e.g., **phenylephrine**) -- Target **α1 receptors**. - **Hypertension treatment** -- Target **α2 receptors** in the CNS. - **Facial erythema treatment** -- Stimulate direct vasoconstriction via **α2 receptors**. **Uses of α-Adrenoceptor Antagonists (Alpha-Blockers)** - **Hypertension treatment** (e.g., **doxazosin**) -- Targets **α1 receptors**, stimulating vasodilation. - **Benign prostatic hyperplasia treatment** (e.g., **tamsulosin**) -- Targets **α1 receptors** in the prostate and urethra to relax them, alleviating urinary difficulties. **Uses of β-Adrenoceptor Agonists** - **Cardiogenic shock** (e.g., **adrenaline/dobutamine**) -- Targets **β1 receptors** on the heart to increase heart rate and contractility. - **Anaphylactic shock** (e.g., **epinephrine/adrenaline**) -- Targets **α** and **β1** receptors to restore blood pressure. - **Asthma** (e.g., **salbutamol**) -- Targets **β2 receptors** to relax bronchial smooth muscle. Salbutamol can also delay premature labor via intravenous injection. **Uses of β-Adrenoceptor Antagonists (Beta-Blockers)** - **Angina, cardiac arrhythmias, and heart failure**. - **Hypertension treatment**. - **Anxiety treatment**. - **Glaucoma treatment** (e.g., **timolol**). - Example: **Metoprolol** targets **β1 receptors**. **Drugs Targeting the SyNS via Catecholamine Regulation** **Regular Noradrenaline (NA) Regulation** 1. **Synthesis**: NA is synthesized from tyrosine through a multi-step mechanism involving dopamine. 2. **Storage and Release**: NA is stored in secretory vesicles and released into the synapse upon action potential. 3. **Reuptake**: NA is reabsorbed by the presynaptic bulb via the **noradrenaline transporter (NAT)**, terminating its action. 4. **Metabolism**: Some NA may be broken down by **mono-amine oxidase (MAO)** enzymes. Drugs can interfere with this regulation in the following ways: **1. NAT Inhibitors** By inhibiting **NAT**, less NA is reabsorbed, and NA remains in the synaptic cleft longer, leading to prolonged sympathetic activation. - **Examples**: - **Desipramine** (antidepressant) - **Cocaine** (local anesthetic) Side effects include **tachycardia** and **dysrhythmia**. **2. MAO Inhibitors** Inhibiting **MAO** elevates NA levels in presynaptic neurons. This also increases dopamine and serotonin levels in the CNS and PNS. - Side effects: **hypotension**, **weight gain**, **restlessness**, **insomnia**. - **Cheese reaction**: Eating cheese while on MAO inhibitors can cause a hypertensive crisis due to elevated tyramine levels. - **Examples**: - **Moclobemide** - **Phenelzine** **3. Sympathetic Amines** Sympathetic amines, structurally similar to NA, are transported into neurons by **NAT**, where they displace NA from vesicles, causing NA to leak into the synaptic cleft. - Effects include **bronchodilation**, **vasoconstriction**, and increased **blood pressure** (used in hypotension treatment). - These drugs also affect serotonin and dopamine, causing euphoric effects in the CNS, which makes them common drugs in substance abuse. - **Examples**: - **Amphetamine** - **Ephedrine** - **Tyramine** **L18 -- Motor & Sensory Pathways** **Motor Pathways** 1. **Components of Motor Pathways**: - **Upper Motor Neurons (UMN)**: - Originate in the cerebral cortex. - Synapse with Lower Motor Neurons (LMN) or interneurons. - **Lower Motor Neurons (LMN)**: - Originate in the ventral horn of the spinal cord or cranial nerve nuclei. - Directly innervate skeletal muscles. 2. **Motor Homunculus**: - Represents motor control regions in the primary motor cortex. - Illustrates the disproportionate representation of body parts based on motor precision. 3. **Role of the Cerebellum**: - Coordinates movement using: - **Motor input** from the motor cortex. - **Proprioceptive sensory input** (position, speed, movement of limbs). - Feedback via the thalamus to refine motor actions. 4. **Pyramidal Tracts**: - **Corticospinal Tract**: - Originates in the primary and secondary motor cortices. - **Pathway**: - Descends through the corona radiata, internal capsule, and medulla. - At the medulla, forms visible pyramids; decussation occurs here. - **Lateral Corticospinal Tract**: - 85-90% decussate. - Controls fine, skilled voluntary movements (e.g., fingers). - **Ventral Corticospinal Tract**: - 10-15% do not decussate. - Controls axial muscles for posture and swallowing. - **Corticobulbar Tract**: - Controls head and neck muscles by synapsing at cranial nerve nuclei. - Fibres originate similarly to corticospinal tracts but terminate at cranial nerves III-XII. 5. **Extrapyramidal Tracts**: - Originate outside the motor cortex (e.g., basal ganglia, reticular nuclei). - Regulate posture, muscle tone, and involuntary movements. - Examples: Vestibulospinal, Reticulospinal, Rubrospinal, Tectospinal. 6. **Clinical Correlation: Parkinson\'s Disease**: - Caused by dopamine deficiency in basal ganglia. - Symptoms: Akinesia, bradykinesia, rigidity, festinating gait. 7. **Lesions**: - **UMN Lesion**: - Hyperreflexia, spasticity, positive Babinski sign. - **LMN Lesion**: - Hyporeflexia, muscle atrophy, fasciculations. **Sensory Pathways** 1. **Sensory Homunculus**: - Maps body parts in the somatosensory cortex based on sensory input density. 2. **General Structure**: - Involves three orders of neurons: 1. **First-Order Neurons**: - Cell bodies in dorsal root ganglia. - Synapse in spinal cord or brainstem. 2. **Second-Order Neurons**: - Decussate and ascend to the thalamus. 3. **Third-Order Neurons**: - Project from the thalamus to the somatosensory cortex. 3. **Pathways**: - **Dorsal Column Pathway**: 1. Carries fine touch, proprioception. 2. Decussates in the medulla. - **Spinothalamic Tract**: 1. Carries pain, temperature, and crude touch. 2. Decussates at the spinal cord level. - **Spinocerebellar Tracts**: 1. Transmit unconscious proprioception to the cerebellum. 4. **Clinical Focus: Brown-Séquard Syndrome**: - Hemi-lesion of the spinal cord. - Results in: 1. **Ipsilateral loss** of proprioception. 2. **Contralateral loss** of pain and temperature. 5. **Sensory Input from the Face**: - Via trigeminothalamic pathways. - First-order neurons are cranial nerves; synapse in brainstem nuclei. **L19 -- Embryological Development of NS** **Introduction** - The embryo at Week 3 consists of a trilaminar germ disk: - **Ectoderm**: Forms the nervous system, epidermis, and sensory structures. - **Mesoderm**: Develops into connective tissues, muscles, cardiovascular and genitourinary systems, etc. - **Endoderm**: Contributes to epithelial linings of organs and glands. **Neurulation** - The **notochord**, formed in the mesoderm, induces the ectoderm to form the **neural plate** via antagonists like **noggin**, inhibiting BMP-4. - **Neural tube formation**: - Edges of the neural plate elevate (neural crests) and fuse to form the neural tube. - Neural tube closes: - Cranial neuropore: Day 25. - Caudal neuropore: Day 28. **Spinal Cord Development** 1. **Formation of Layers (Week 6)**: - **Ependymal layer**: Inner layer lining the central canal. - **Mantle layer**: Middle layer forming gray matter. - **Marginal layer**: Outer layer forming white matter. 2. **Plate Formation**: - **Alar plates** (dorsal): Sensory neurons -\> dorsal horn. - **Basal plates** (ventral): Motor neurons -\> ventral horn. - **Sulcus limitans**: Separates the alar and basal plates. 3. **Caudal End**: - Secondary neurulation forms the **conus medullaris** and **cauda equina**. - Lumbar punctures are safe at L4-L5 due to the absence of the spinal cord here. **Neural Crest Cells (NCCs)** - Origin: Neuroepithelium at neural tube edges. - Migration pathways: - Cranial, trunk, cardiac, and vagosacral regions. - Contributions: - **Peripheral Nervous System**: Sensory neurons, autonomic neurons, Schwann cells, ganglia. - **Other Structures**: Facial bones, melanocytes, cardiac septum, odontoblasts, adrenal medulla. **Brain Development** - Neural tube at the cranial end forms 3 **primary brain vesicles**: - Forebrain (**prosencephalon**) → Telencephalon, Diencephalon. - Midbrain (**mesencephalon**) → Mesencephalon. - Hindbrain (**rhombencephalon**) → Metencephalon, Myelencephalon. - **Flexures**: - Cephalic (midbrain). - Cervical (hindbrain-spinal cord). **Neural Tube Defects (NTDs)** 1. **Spina Bifida**: Failure of neural tube closure at lumbar-sacral levels. - **Occulta**: Vertebral arches fail to fuse. Minimal symptoms. - **Meningocele**: Protrusion of meninges; moderate neurological defects. - **Myelomeningocele**: Protrusion of meninges and spinal cord; severe defects like hydrocephalus. - **Myeloschisis**: Exposed neural tissue, most severe. 2. **Anencephaly**: Failure of cranial neuropore closure → incomplete brain/skull development. 3. **Chiari II Malformation**: Cerebellum and brainstem herniate → hydrocephalus. **Diagnosis and Risk Factors**: - Maternal alpha-fetoprotein levels, ultrasound (e.g., lemon sign for Chiari II). - Risk: Maternal diabetes, obesity, folic acid deficiency, certain medications (e.g., valproic acid). **Congenital Disorders from NCC Defects** - Examples: Treacher Collins syndrome, cleft palate, peripheral nervous system malformations. **L20 -- Damage & Repair in the injured CNS** **Damage and Repair in the Injured CNS** **Key Concepts** - The CNS includes the brain and spinal cord, which are highly complex but lack robust regenerative capacity. - Injury consequences depend on the **site and size** rather than the type of injury. - Major challenges arise from intrinsic cellular mechanisms and the inhibitory environment within the CNS. **Types of CNS Injury** - **Spinal Cord Injury (SCI)**: - Loss of motor, sensory, and autonomic function below the level of injury due to disruption in ascending and descending pathways. - **Traumatic Brain Injury (TBI)**: - Includes hematomas (epidural, subdural), structural damage (contusions, diffuse axonal injury), and hypoxic lesions. - **Stroke**: - Can be ischemic (energy crisis) or hemorrhagic; involves progressive neuronal death. - **Brain Cancer**: - Compression and disruption of CNS tissue by tumors. **Complexity of Nervous Tissue** - CNS injuries are challenging to repair because neurons: - Form intricate, irreplaceable networks. - Do not regenerate by cell division. - Synaptic plasticity allows functional changes but cannot replace lost neurons or networks. **Topographical Organization of the CNS** - **Brain regions** have specific functions: - **Visual cortex**: Visual processing. - **Motor cortex**: Voluntary movement control. - **Broca's and Wernicke's areas**: Language production and comprehension. - **Frontal lobe**: Executive functions. - **Cerebellum**: Coordination and balance. - Damage to specific areas results in predictable deficits (e.g., language impairment from Broca's area damage). **Energy Dependence** - Brain energy facts: - 2% of body mass but uses 15% of body energy. - No internal energy stores; relies exclusively on glucose metabolism. - Vulnerable to **energy crises** due to: - **Ischemia**: Reduced blood flow. - **Hypoglycemia**: Low blood sugar. - **Severe anemia**: Insufficient oxygen delivery. **Injury Mechanisms** **Traumatic Brain Injury** - Impact and brain movement result in: - **Hematomas**: Blood accumulation compressing the brain. - **Diffuse Axonal Injury**: Shearing of axons due to forceful movement. - **Hypoxia** results in an energy crisis, causing irreversible damage. **Hypoxic-Ischemic Injury** - **Timeline** of neuron damage: - **4-5 minutes**: Irreversible damage to highly sensitive neurons (hippocampal pyramidal cells, Purkinje cells). - **Prolonged hypoxia**: Damage to less sensitive neurons (thalamic, brainstem). - **Progression**: - **1-2 days**: Anoxic neuron swelling. - **2 weeks**: Tissue necrosis, neovascularization. - **2 months**: Glial scar formation by astrocytes and microglia. **Hemorrhages** - Types: - **Cerebral hemorrhage**: Blood leaks into ventricles. - **Lobar hemorrhage**: Confined to one region; often linked to amyloid angiopathy or trauma. - **Lacunar infarcts**: Small, fluid-filled cavities due to untreated hypertension or diabetes, leading to cognitive impairments like vascular dementia. **Consequences of CNS Injury** 1. **Primary Damage**: - Loss of neurons and synapses. - Breach of the blood-brain barrier (BBB). 2. **Secondary Damage**: - Inflammation: Microglia and astrocytes release inflammatory mediators. - Oedema: BBB breakdown causes swelling, further reducing oxygen supply. 3. **Long-Term Effects**: - Persistent deficits, seizures, dementia, or vegetative states. **CNS Repair Challenges** - Unlike the PNS, CNS repair is limited due to: - Lack of macrophage-mediated debris clearance. - Astrocytes release inhibitory ECM molecules, such as **chondroitin sulfate proteoglycans (CSPGs)**. - CNS axons have intrinsic regenerative potential, but the environment is prohibitive. **Neuroplasticity** - The brain can reorganize itself to some extent: - **Early Recovery**: Local neuronal changes. - **Late Recovery**: Structural neuroplasticity. - **Principles**: Use it or lose it, repetition matters, intensity matters, etc. - **Factors**: Younger patients and earlier interventions lead to better outcomes. **Treatment Approaches** **Medical and Surgical Interventions:** - Hematoma removal, skull fracture repair, decompression. - Medications: - **Anti-seizure** drugs: Prevent further damage. - **Diuretics**: Reduce swelling. - **Induced coma**: Allows the brain to heal. **Rehabilitation:** - Involves activities tailored to stimulate damaged neurons. - Focuses on repetition, intensity, and salience. - Aims to optimize physical, mental, and social capacity. **Experimental Treatments:** 1. **Trophic Support**: - Neurotrophic factors help sustain neuronal survival. 2. **Inhibiting Inhibitors**: - NOGO antibodies and **chondroitinase** promote regeneration. 3. **Stem Cell Therapy**: - Stem cells from the **subventricular zone** and **subgranular zone** encourage neurogenesis. - Autologous stem cells may replace lost neurons or bridge tissue gaps. **CNS Injury Prognosis** - Most improvement occurs within 6 months, but recovery can extend to 2 years or more. - Up to 5% of patients improve after 12 months. - Prognosis is generally excellent for mild to moderate stroke. **AP01 -- Structure and Ultrastructure of Nervous Tissue** **Introduction** - The nervous system comprises neurons and glial cells. - **Neurons**: - Functional units of the nervous system; number declines with age. - Cannot regenerate once damaged. - **Glial Cells**: - Supportive cells of the nervous system; can regenerate. - **CNS vs. PNS**: - CNS (Central Nervous System): Covered by meninges. - PNS (Peripheral Nervous System): Located outside the meninges. **Neuron Health and Nucleus Position** - **Central Nucleus**: Indicates a healthy neuron. - **Peripheral Nucleus**: Suggests injury; the neuron is undergoing chromatolysis. **Neuronal Anatomy** - Neurons consist of: - **Cell Body (Perikaryon)**: - Contains the nucleus and organelles. - Responsible for metabolic and protein synthesis activities. - **Axon**: - Propagates action potentials. - Single axon per neuron. - Specialized regions: - **Axon Hillock**: Site of action potential initiation. - **Axon Terminal**: End of the axon where synaptic transmission occurs. - **Nodes of Ranvier**: Gaps in the myelin sheath that enhance conduction speed. - **Internodal Regions**: Segments covered by myelin. **Types of Neurons** **Somatic Efferent (Motor) Neurons** - **Structure**: - Multipolar morphology: Cell body has multiple processes radiating in different directions. - Cell body located in the **ventral horn** of the spinal cord or **cranial nerve motor nuclei**. - Heavily myelinated axons for fast conduction. - **Types**: - **Alpha Motor Neurons**: - Innervate highly contracting muscle fibers. - Facilitate powerful contractions. - **Gamma Motor Neurons**: - Innervate slightly contracting muscle fibers. - Ensure muscle sensitivity to stretch over various lengths. - **Function**: - Transmit signals from the CNS to muscles to initiate movement. **Somatic Afferent (Sensory) Neurons** - **Structure**: - Pseudo-unipolar morphology: A single axon splits into two branches. - Cell body located in ganglia outside the CNS. - Heavily myelinated (Alpha and Beta subtypes) for faster conduction; lightly myelinated (Gamma) and unmyelinated (C fibers) for slower signals, such as pain. - **Function**: - Detect sensory stimuli and convert electromechanical signals into electrical impulses. - Signal propagation: - Peripheral branch generates and conducts impulses to the cell body. - Central branch transmits impulses to the CNS. **Bipolar Neurons** - Found in specialized sensory systems like the retina. **Neuron Function** - **Axonal Transport**: - Movement of materials along the axon. - Facilitated by motor proteins: - **Anterograde Transport**: From cell body to axon terminal via kinesins. - **Retrograde Transport**: From axon terminal to cell body via dyneins. **Myelination** - Myelin sheath provides insulation and increases conduction speed. - **Schwann Cells**: - Found in the PNS. - Myelinate one axon per cell. - **Oligodendrocytes**: - Found in the CNS. - Myelinate multiple axons simultaneously. - **Unmyelinated Axons**: - Present in sensory neurons like C fibers (responsible for pain sensation). - All motor neurons are myelinated. **Histological Features of Ne