Muscle Physiology PDF - Components and Function
Document Details
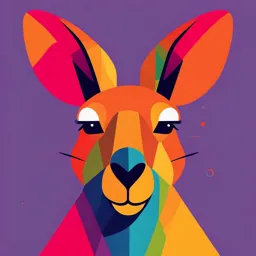
Uploaded by DeservingPoplar
University of Victoria
Tags
Summary
This document details the components of the somatic motor pathway, the structure and function of the neuromuscular junction (NMJ), and compares the three types of muscle. It describes chemical agents, muscle contraction, and the roles of different muscle proteins and contraction cycles . It also covers skeletal muscle relaxation.
Full Transcript
— Module 2 Identify the components of a somatic motor pathway The somatic motor pathway controls voluntary movements by transmitting signals from the central nervous system (CNS) to skeletal muscles 1. Upper Motor Neurons (UMNs) Location: Primary motor cortex (precentral gyrus) of the fr...
— Module 2 Identify the components of a somatic motor pathway The somatic motor pathway controls voluntary movements by transmitting signals from the central nervous system (CNS) to skeletal muscles 1. Upper Motor Neurons (UMNs) Location: Primary motor cortex (precentral gyrus) of the frontal lobe. Function: Initiate voluntary movement by sending impulses down the spinal cord. Pathways: Travel via descending tracts, mainly: ○ Corticospinal tract (controls limb and trunk muscles). ○ Corticobulbar tract (controls muscles of the face and head via cranial nerves). 2. Lower Motor Neurons (LMNs) Location: Brainstem (for cranial nerves) and anterior horn of the spinal cord (for spinal nerves). Function: Directly innervate skeletal muscles and cause contraction. Pathway: Receive input from UMNs and transmit signals through spinal nerves or cranial nerves to muscles. Describe the structural and functional operation of the neuromuscular junction and explain how chemical agents alter events at the neuromuscular junction The neuromuscular junction (NMJ) is a specialized synapse between a lower motor neuron and a skeletal muscle fiber. It is responsible for converting electrical nerve impulses into muscle contractions Structure of the NMJ - Presynaptic Terminal (Axon Terminal of LMN) ○ Contains synaptic vesicles filled with the neurotransmitter acetylcholine (ACh). ○ When an action potential reaches the terminal, voltage-gated calcium (Ca²⁺) channels open, allowing Ca²⁺ influx. ○ This triggers the release of ACh into the synaptic cleft via exocytosis. Synaptic Cleft ○ A small gap between the motor neuron and the muscle fiber. ○ ACh diffuses across this space and binds to receptors on the muscle cell membrane. Postsynaptic Membrane (Motor End Plate of Muscle Fiber) ○ Contains nicotinic acetylcholine receptors (nAChRs), which are ligand-gated ion channels. ○ When ACh binds, Na⁺ (sodium) ions enter the muscle cell, causing depolarization. ○ If the depolarization reaches the threshold, an action potential propagates along the muscle fiber, leading to contraction. Acetylcholinesterase (AChE) Enzyme ○ Located in the synaptic cleft. ○ Rapidly breaks down ACh into acetate and choline to prevent continuous stimulation. ○ Choline is reabsorbed into the presynaptic neuron to synthesize more ACh Function of the NMJ (summary) - 1. Action Potential Arrival – A nerve impulse reaches the axon terminal of the LMN. 2. Calcium Influx – Voltage-gated Ca²⁺ channels open, and Ca²⁺ enters the neuron. 3. ACh Release – Synaptic vesicles release ACh into the synaptic cleft. 4. ACh Binding – ACh binds to nicotinic receptors on the motor end plate. 5. Na⁺ Influx & Depolarization – The receptor opens, allowing Na⁺ influx, generating an end-plate potential (EPP). 6. Muscle Action Potential – If EPP reaches threshold, voltage-gated Na⁺ channels open, propagating an action potential. 7. Muscle Contraction – The action potential spreads via T-tubules, triggering calcium release from the sarcoplasmic reticulum, leading to contraction. 8. ACh Breakdown – Acetylcholinesterase degrades ACh, stopping the signal Compare the three types of muscle 1. Skeletal Muscle Structure: ○ Striated, elongated, multinucleated myofibers Function: ○ Responsible for voluntary movement ○ Controls the movement of joints Location: ○ Attached to bones 2. Cardiac Muscle Structure: ○ Striated, short, branching cells ○ Single centrally placed nucleus ○ Intercalated discs and gap junctions allow synchronized contractions Function: ○ Involuntary control ○ Autorhythmic pacemaker cells maintain a rhythmic heartbeat without nervous system input Location: ○ Found only in the heart 3. Smooth Muscle Structure: ○ Non-striated, spindle-shaped cells ○ Single central nucleus ○ No sarcomeres; actin and myosin anchored to dense bodies ○ Lacks T-tubules Function: ○ Involuntary control ○ Stretches and contracts, maintains contraction without fatigue (e.g., sphincters) ○ Controlled by the autonomic nervous system Location: ○ Found in walls of hollow organs (e.g., stomach, intestines, bladder, blood vessels) Explain the function of different muscle proteins 1. Contractile Proteins: Generate Force for Contraction 2. Regulatory Proteins: Control Contraction 3. Structural Proteins: Provide Alignment, Stability, and Elasticity Explain the significance of the sliding filament mechanism The sliding filament mechanism explains how muscles contract by the interaction between thick (myosin) and thin (actin) filaments within the sarcomere. The filaments do not shorten but instead slide past each other, leading to muscle shortening Key Principles of the Sliding Filament Mechanism - Muscle contraction occurs when the sarcomere shortens. Thick (myosin) and thin (actin) filaments overlap but do not change in length. Cross-bridge cycling pulls actin toward the center of the sarcomere. Z-discs move closer together, reducing sarcomere length. Steps of Muscle Contraction (Sliding Filament Theory) - 1. Cross-Bridge Formation ○ Myosin heads bind to actin, forming cross-bridges. 2. Power Stroke ○ Myosin pulls actin toward the M-line, shortening the sarcomere. ○ ATP is used to release and reset the myosin head. 3. Detachment & Resetting ○ New ATP binds to myosin, causing detachment from actin. ○ ATP hydrolysis resets the myosin head for the next cycle. 4. Repeat ○ The process continues as long as calcium (Ca²⁺) and ATP are available. Structure Change During Contraction Sarcomere Shortens → Z-Discs → Move closer together A-Band → Remains the same (thick filaments do not shorten) I-Band → Shortens (thin filaments slide inward) H-Zone → Narrows or disappears Significance of the Sliding Filament Mechanism - Explains how muscle fibers shorten without changing filament length Demonstrates the role of ATP in contraction and relaxation Describes the importance of calcium ions (Ca²⁺) in regulating contraction Forms the basis of all voluntary and involuntary muscle movement Outline the steps of the contraction cycle Steps of the Contraction Cycle - 1. Cross-Bridge Formation ○ Calcium (Ca²⁺) binds to troponin, causing tropomyosin to shift and expose myosin-binding sites on actin. ○ Myosin heads attach to actin, forming cross-bridges. 2. Power Stroke ○ Myosin heads pivot, pulling actin filaments toward the M-line (center of the sarcomere). ○ ADP (Adenosine Diphosphate) and Pi (inorganic phosphate) are released, generating force. 3. Cross-Bridge Detachment ○ ATP binds to myosin, causing it to detach from actin. ○ Without ATP, myosin remains attached (as seen in rigor mortis). 4. Myosin Reset (Cocking of Myosin Head) ○ ATP is hydrolyzed (split into ADP + Pi), re-energizing myosin heads. ○ Myosin returns to its pre-stroke "cocked" position, ready for another cycle Describe excitation-contraction coupling in skeletal muscle fiber Excitation-contraction coupling is the process linking an action potential in the muscle fiber to muscle contraction. This involves electrical signaling, calcium release, and the initiation of crossbridge cycling Steps of Excitation-Contraction Coupling - Neuromuscular Junction Activation (Excitation): 1. Action potential travels down the motor neuron. 2. Acetylcholine (ACh) is released from the axon terminal into the synaptic cleft. 3. ACh binds to receptors on the motor end plate. 4. Ligand-gated Na⁺ and K⁺ channels open → More Na⁺ enters than K⁺ exits, leading to depolarization. 5. End Plate Potential (EPP) forms → If threshold is reached, voltage-gated Na⁺ channels open, generating an action potential that spreads along the sarcolemma. Action Potential Propagation: 6. Action potential travels down the sarcolemma and into T-tubules. 7. DHP receptors in the T-tubules detect voltage changes and activate ryanodine receptors on the sarcoplasmic reticulum (SR). Calcium Release from the SR & Crossbridge Formation & Contraction: 8. Ryanodine receptors open, allowing Ca²⁺ to flood the cytosol from the SR. 9. Calcium-induced calcium release amplifies Ca²⁺ release via ligand-gated channels. 10.Ca²⁺ binds to troponin, causing tropomyosin to shift, exposing myosin-binding sites on actin. 11.Myosin heads bind to actin, initiating crossbridge cycling and muscle contraction. Discuss how a skeletal muscle relaxes after a period of contraction Steps of Muscle Relaxation - Nerve signal stops → No more action potentials from the motor neuron. ACh breakdown → Acetylcholinesterase (AChE) breaks down acetylcholine in the synaptic cleft, stopping muscle fiber excitation. Calcium reabsorption → Ca²⁺ is actively pumped back into the sarcoplasmic reticulum (SR) by Ca²⁺-ATPasepumps, reducing cytosolic calcium levels. Troponin releases Ca²⁺, causing tropomyosin to block myosin-binding sites on actin. Crossbridges detach → Without calcium, myosin can no longer bind to actin, stopping contraction. Muscle returns to resting length Describe the importance of a motor unit A motor unit consists of: One motor neuron All the muscle fibers it innervates The human body has ~250 million muscle fibers but only 420,000 motor neurons, meaning each neuron controls multiple fibers. A single motor neuron can innervate 1 to 150+ muscle fibers, depending on the muscle’s function. Synchronized Contraction: All fibers in a motor unit contract and relax at the same time when stimulated by the neuron Importance - 1. Control of Muscle Contraction Strength Small motor units (few fibers per neuron) → Allow precise movements (e.g., eye muscles). Large motor units (many fibers per neuron) → Generate powerful movements (e.g., leg muscles). 2. Fatigue Resistance Different motor units are recruited in rotation to delay fatigue in muscles (especially in postural muscles). 3. Motor Unit Recruitment & Force Generation More motor units are activated when more force is needed (called recruitment). This follows the size principle → Smaller units (slow-twitch, fatigue-resistant) activate first, then larger units (fast-twitch, high-power). 4. Coordinated Movements Motor units work together to ensure smooth, controlled movements instead of jerky, uncoordinated contractions. Discuss factors that determine muscle tension and force 1. Motor Unit Recruitment More motor units = Greater force production. Follows the size principle: ○ Small motor units (slow-twitch, fatigue-resistant) are recruited first. ○ Large motor units (fast-twitch, high-power) are recruited as needed. 2. Muscle Fiber Size and Type Larger fibers (fast-twitch) produce more force. Smaller fibers (slow-twitch) generate less force but have greater endurance 3. Biochemical Properties These determine the fiber’s ability to produce and use energy. Oxidative Capacity (Affects endurance & fatigue resistance) ○ Higher oxidative capacity → More ATP from aerobic metabolism → Greater endurance. ○ Determined by: Number of capillaries (More oxygen delivery). Mitochondria (More ATP production via aerobic respiration). Amount of myoglobin (Oxygen storage for sustained activity). Type of Myosin ATPase (Affects speed of ATP breakdown & contraction) ○ Fast myosin ATPase → Faster crossbridge cycling → Faster contraction. ○ Slow myosin ATPase → Slower contraction but more efficient ATP use. 4. Contractile Properties These determine how much force a muscle fiber can generate and how quickly it contracts. Maximal Force Production ○ Force is proportional to the cross-sectional area of muscle fibers. ○ Larger fibers = More myofibrils = Greater force. Speed of Contraction (Vmax) ○ Determined by myosin ATPase activity (fast vs. slow ATP breakdown). ○ Fast fibers contract more quickly than slow fibers. Muscle Fiber Efficiency ○ Efficient fibers use less ATP to generate force → Better endurance. ○ Slow-twitch (Type I) fibers are more efficient than fast-twitch (Type II) fibers Distinguish between different types of muscle contraction Isotonic Contraction (Muscle Changes Length) - The muscle generates force while changing length. Concentric Contraction (Muscle Shortens) ○ Force is greater than resistance → Muscle shortens. ○ Example: Lifting a dumbbell in a bicep curl (biceps contract concentrically). Eccentric Contraction (Muscle Lengthens) ○ Resistance is greater than force → Muscle lengthens while still contracting. ○ Example: Lowering a dumbbell in a bicep curl (biceps contract eccentrically to control movement). ***Eccentric contractions generate more force than concentric ones but cause more muscle damage (leading to muscle growth and soreness). Isometric Contraction (Muscle Does Not Change Length) - The muscle generates force without changing length. Example: Holding a plank position, gripping an object without moving it. Occurs when resistance = force, preventing movement. ***Isometric contractions help with stabilization and joint support. Isokinetic Contraction (Constant Speed, Specialized Equipment) - Muscle contracts at a constant speed with varying resistance. Requires specialized machines to maintain speed (e.g., rehabilitation settings). Describe the differences among the three types of skeletal muscle fibers Fast-Twitch Fibers (Type II) - Characteristics: Rapid energy generation for quick, powerful muscle actions High myosin ATPase activity → Faster contraction Well-developed sarcoplasmic reticulum for fast Ca²⁺ release and uptake High rate of cross-bridge turnover → More force production Performance & Function: Contract 3 to 5 times faster than slow-twitch fibers Rely on anaerobic glycolysis for ATP production (short-term energy) Suited for sprinting, jumping, and explosive movements Important in stop-and-go sports (e.g., basketball, soccer, lacrosse) Subtypes of Type II Fibers: Type IIa → Fast-Oxidative-Glycolytic (FOG); can use both aerobic & anaerobic energy Type IIx (previously IIb) → Intermediate between IIa & IIb "New" Type IIb → Fast-Glycolytic; most anaerobic, highest speed & force 2. Slow-Twitch Fibers (Type I) - Characteristics: Generate ATP through aerobic metabolism Low myosin ATPase activity → Slower contraction Slow Ca²⁺ handling & shortening speed Large and numerous mitochondria → High oxygen capacity Performance & Function: Highly fatigue-resistant, ideal for prolonged endurance activities Slow oxidative metabolism → Efficient for long-duration, low-intensity exercise Active in endurance sports like marathon running, cycling, and swimming Also recruited in postural muscles for sustained activity Describe the properties of cardiac muscle and smooth muscle 1. Smooth Muscle - Smooth muscle is found in the walls of hollow organs (e.g., intestines, blood vessels, airways) and lacks striations. It contracts involuntarily and is regulated by the autonomic nervous system and hormones. Classification of Smooth Muscle - Smooth muscle is classified into single-unit and multiunit types based on how the muscle fibers are activated. A. Single-Unit Smooth Muscle: Most common type → Found in the intestinal tract, blood vessels, and respiratory tract Muscle fibers contract together as a single unit due to gap junctions (electrical signals spread between cells) Pacemaker cells generate spontaneous depolarizations, leading to rhythmic contractions Tone → Muscle maintains a level of contraction even without stimulation Graded contractions (No recruitment; contraction strength varies with intracellular calcium levels) Stretch reflex → Relaxes in response to sudden or prolonged stretch (e.g., blood vessels adapting to pressure changes) B. Multi-Unit Smooth Muscle: Found in: Large airways, large arteries, and the eye (ciliary muscle, iris) Few, if any, gap junctions → Each fiber acts independently Each muscle fiber receives its own innervation → More precise control No tone (does not maintain baseline contraction) Recruitment occurs, allowing graded control of contraction strength 2. Cardiac Muscle - Cardiac muscle is found only in the heart and shares properties with both skeletal and smooth muscle. Similarities with Skeletal Muscle - Striated (has sarcomeres, similar to skeletal muscle) Troponin-regulated contraction (Ca²⁺ binds to troponin, allowing actin-myosin interaction) T-tubules present for action potential conduction Sarcoplasmic reticulum (SR) stores and releases calcium for contraction Similar to slow oxidative fibers: High myoglobin (stores oxygen) Many mitochondria → Relies heavily on aerobic metabolism Fatigue-resistant Similarities with Smooth Muscle - Gap junctions (found in intercalated discs) allow coordinated contraction Pacemaker cells generate spontaneous depolarizations (heartbeat is self-regulated) Autonomic nervous system innervation (modulates heart rate & force) Hormonal influence (e.g., epinephrine increases heart rate) Calcium source: Comes from both extracellular fluid and sarcoplasmic reticulum Discuss some of the components involved in reflexes and describe some of the basic motor reflexes Reflexes are involuntary, rapid responses to stimuli that help maintain posture, prevent injury, and coordinate movement. They involve sensory receptors, neural pathways, and motor responses. 1. Components of a Reflex Arc - A reflex arc is the neural pathway involved in reflex actions. It consists of: 1. Receptor – Detects the stimulus (e.g., muscle spindle for stretch, Golgi tendon organ for tension). 2. Afferent (Sensory) Neuron – Carries sensory information to the spinal cord. 3. Integration Center – Located in the spinal cord (monosynaptic or polysynaptic). 4. Efferent (Motor) Neuron – Transmits the response signal to the muscle. 5. Effector – The muscle or gland that responds to the stimulus. 2. Types of Reflexes - A. The Stretch Reflex (Knee-Jerk Reflex) - Function: Prevents overstretching of muscles by contracting them when stretched too far. Example: Patellar reflex – When the quadriceps muscle is stretched, it contracts to prevent excessive elongation. Receptor: Muscle spindle (detects muscle stretch). Mechanism: 1. Muscle spindle detects rapid stretch. 2. Afferent nerve fibers send signals to the spinal cord. 3. Alpha motor neurons trigger contraction of the same muscle. 4. Reciprocal inhibition prevents contraction of the opposing muscle. Type of Reflex Arc: Monosynaptic reflex (involves a single synapse between a sensory and motor neuron). B. The Withdrawal (Flexion) Reflex - Function: Rapidly withdraws a limb from a painful stimulus. Example: Pulling your hand away from a hot surface. Receptor: Pain receptors (nociceptors). Mechanism: 1. Pain receptors detect a harmful stimulus. 2. Afferent neurons send a signal to the spinal cord. 3. Interneurons activate motor neurons to contract the flexor muscles. 4. Reciprocal inhibition relaxes the extensor muscles. Type of Reflex Arc: Polysynaptic reflex (involves multiple synapses). C. The Flexion (Withdrawal) and Crossed-Extensor Reflexes - Function: The withdrawal reflex removes the affected limb from danger, while the crossed-extensor reflex maintains balance by extending the opposite limb. Example: If you step on a sharp object with your right foot, your left leg extends to support your weight. Mechanism: 1. Pain receptors in the injured limb send signals to the spinal cord. 2. The flexion withdrawal reflex activates flexor muscles in the affected limb. 3. The crossed-extensor reflex activates extensor muscles in the opposite limb to maintain posture. Type of Reflex Arc: Polysynaptic reflex (involves multiple synapses and both sides of the body). 3. Sensory Organs in Skeletal Muscle Reflexes - A. Muscle Spindle - Function: Detects changes in muscle length and initiates the stretch reflex. Structure: ○ Made up of intrafusal fibers, connective tissue, and sensory nerve endings. ○ Intrafusal fibers are contractile cells within the spindle. ○ Gamma motor neurons adjust spindle sensitivity. ○ Innervated by afferent nerve fibers, which detect stretch. B. Golgi Tendon Organ (GTO) - Function: Detects muscle tension and prevents excessive force by triggering the Golgi tendon reflex (muscle relaxation). Structure: ○ Located in tendons. ○ Innervated by afferent nerve fibers, which detect tension. 4. Muscle Fiber Types in Reflexes - Extrafusal Fibers: Contractile muscle cells responsible for skeletal muscle contraction. Innervated by alpha motor neurons. Intrafusal Fibers: Found within muscle spindles. Adjust sensitivity of the muscle to stretch. Innervated by gamma motor neurons.