Muscle Physiology- Pt 1.docx
Document Details
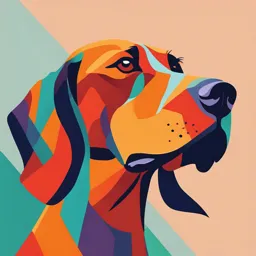
Uploaded by ashp1101
Full Transcript
Diffusion (Electrical) Potential Diffusion Potential is the charge (potential difference) generated by ions (charge molecules) moving down their concentration gradient. For example, if sodium is diffusing into the cell, the charge inside of the cell becomes more positive while the external environme...
Diffusion (Electrical) Potential Diffusion Potential is the charge (potential difference) generated by ions (charge molecules) moving down their concentration gradient. For example, if sodium is diffusing into the cell, the charge inside of the cell becomes more positive while the external environment of the cell becomes more negatively charged. Uncharged molecules use the concentration gradient for their driving force of movement. Charged molecules use the concentration gradient in combination with electrical charges for their driving force of movement. The diffusion potential is dependent on the concentration gradient and the permeability of the membrane. For example, if there is no permeability for an ion, then there would be no diffusion potential present. For example, if there is no concentration gradient, there would also ne no diffusion potential present. The diffusion potential’s ion movement does NOT cause measurable changes in concentration of ions in bulk solutions, however there can be a large change in electrical potential. Equilibrium Potential The equilibrium potential stops ion movement as it is the diffusion potential that balances the tendency of ion diffusion down its concentration gradient. Membrane Potential The membrane potential is the difference in electrical potential between the interior and exterior of a biological cell. The membrane potential across the membrane surface does NOT vary with time, with the only exception of neurons and muscle cells which undergo controlled changes to their membrane potential. Membrane potential exits across the membrane of all cells. The membrane potential is dependent on the electrical potential of different ions and the permeability of those ions. Resting Potential The resting potential is when the membrane potential goes a long period of time without changing significantly. The resting potential can also be referred to as being the membrane potential of non-excitable cells or the membrane potential of excitable cells that lack excitation. The resting potential is approximately -70millivolts but can range between -40 to -90millivolts. The -70millivolt charge refers to the inside of the cell being 70millivolts more negative than the outside of the cell. The diffusion of potassium has a larger impact on the resting potential in comparison to sodium. Resting Potential on Nerve Cells The resting potential is influenced by the sodium-potassium pump, and the diffusion of sodium and potassium through the nerve cell membrane. Potassium diffusion through the nerve cell membrane Potassium leak channels allow for potassium diffusion OUT OF the cell, resulting in an increased negative charge within the cell. The electrical driving force of the negativity within the cell will eventually cause potassium’s attraction to the opposite charge to pull it back into the cell. The potassium leak channels are 100times more selective for potassium than sodium ions, leaving the sodium anions within the cell. Sodium diffusion through the nerve cell membrane Sodium leak channels allow for the diffusion of sodium INTO the cell, causing an increased positive charge into the cell. The membrane is much more permeable to potassium than it is to sodium. Sodium-potassium pump The sodium potassium pump will transport 3 sodium ions out of the cell and will transport 2 potassium ions into the cell, overall helping to maintain the negative internal charge of the cell. The sodium-potassium pump has an ATPase function and becomes activated upon binding. Action Potential: General Info Changes in ion concentration can be due to the closing and opening of ion channels or due to the change in activity of membrane pumps. An action potential is the rapid change in membrane potential that spreads along a nerve fiber membrane until it reaches the nerve fiber’s end. Each action potential starts with a rapid change from the membrane’s normal negative resting membrane potential to a positive membrane potential. The refractory period of an already depolarized area forces a direction of propagation along the membrane of the axon until the entire membrane has become depolarized. Absolute Refractory Period vs Relative Refractory Period Absolute Refractory Period The absolute refractory period takes place throughout the entire action potential, excluding the hyperpolarization stage. The absolute refractory period also covers the amount of time needed for the voltage gated sodium channels to revert from being in an inactive state to the resting closed state. The only thing that will allow the voltage gated sodium channels to re-open is for the membrane to return to it’s resting membrane potential. During the absolute refractory period, an action potential is completely blocked from being initiated. This means that during the absolute refractory period that another action potential cannot start while another action potential is already taking place. Relative Refractory Period The relative refractory period only takes place during the hyperpolarization stage of the action potential. The relative refractory period also covers the time when the voltage gated sodium channels are closed but not inactive. During the relative refractory period, an action potential is difficult to achieve due to the negative charge of the membrane, but it is not impossible to achieve. Therefore, if a strong enough stimulus takes place, causing the membrane to reach threshold during the relative refractory period, an action potential can be initiated. Action Potential: Stages Step 1: Resting Stage During the resting stage, the membrane is at a resting membrane potential of approximately -70millivolts. The resting stage takes place prior to the start of the action potential. Step 2: Threshold The membrane reaches a membrane potential of -55millvolts, achieving threshold. The threshold is the voltage required to trigger the beginning of an action potential. Threshold can be met due to electrical, mechanical, or chemical stimuli. Step 3: Depolarization Upon threshold being met, the membrane becomes more permeable to sodium ions and the voltage gates sodium channels open (due to positive feedback), causing the influx of sodium into the cell which then results in an increased positive charge within the cell, resulting in depolarization. In the late part of depolarization, the voltage gated potassium channels open causing potassium to exit the cell, slowing down the rate of depolarization. Depolarization is defined as an influx of positive charge within the cell. Step 4: Repolarization After the membrane becomes highly permeable to sodium, the voltage gated sodium channels close, resulting in an increased negative charge within the cell, also known as repolarization. During this period, the voltage gated potassium channels remain open with potassium exiting the cell. Repolarization is when the membrane returns to it’s normal negative resting membrane potential. Step 5: Hyperpolarization (AKA: relative refractory period) Hyperpolarization is when the membrane potential goes below the resting membrane potential before eventually returning back to it. Hyperpolarization is due to there being no more positive sodium ions entering the cell and there still being positive potassium ions exiting the cell, continuing the decline in negative charge within the cell. Once the membrane potential returns to the resting membrane potential voltage of -70millivolts, the voltage gated potassium channels close. This closure of the voltage gated potassium channel results in hyperpolarization.