Muscle Bio Week 1.docx
Document Details
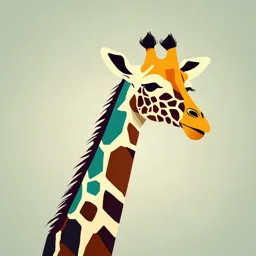
Uploaded by ComprehensiveOrangutan
Deakin University
Tags
Full Transcript
Muscle Bio Week 1 ***Week 1, Module 1: Energy Transfer and Metabolic Fuels*** - Our ability to generate energy is the result of constantly occurring chemical reactions within the body, this is known as our metabolism. - Energy is provided by ATP - In comparison to rest, ATP demand in s...
Muscle Bio Week 1 ***Week 1, Module 1: Energy Transfer and Metabolic Fuels*** - Our ability to generate energy is the result of constantly occurring chemical reactions within the body, this is known as our metabolism. - Energy is provided by ATP - In comparison to rest, ATP demand in skeletal muscle can increase by several hundred times during high intensity exercise - Little ATP is stored in muscle fibres - ATP needs to be rapidly replenished in response to exercise - Metabolic fuels that refill ATP via metabolic pathways are known as energy systems. [Overview of Muscle Structure and Muscle Contraction ] Video notes: origin of muscle research through the sliding filament theory. - All animals move through coordinated contraction of skeletal muscle and is powered by ATP - ATP wasn\'t discovered until 1929 - Skeletal muscle are attached to bones via tendons - Cardiac muscle is different to skeletal muscle. Cells in here are individual cells and are firmly attached to each other. But both have striations - The sarcomere is the contractile unit of the striated muscle cell. These are overlaps of thin and thick filaments. - Thin filaments \> actin, thick filaments \> myosin - Contraction of muscle is a result of the myofibrils and the sarcomeres within them contract, due to the sliding of the thick and thin filaments sliding over each other. - When muscles shorten, the A bands don\'t change in size, but the I bands get smaller and smaller. - Sliding filament theory: some science guys was using live muscle and studied it, watching how the bands changed.\ The I band (made up of actin) slides past the myosin thick filaments (that make up the A band) that lead to a shorter sarcomere = shorter muscle. - Myosin is in the A band and actin is in the I band. - In the overlap region, there are little cross bridges, that are the heads of the myosin molecules. They reach across and bind to actin. - In the cross section, there\'s a hexagonal array to maximally utilise ATP and give efficient muscle contraction. [Introduction to Bioenergetics and Energy Transfer ] - ATP is a stored form of energy obtained from our food - ATP contains an adenine nucleotide, and a ribose (sugar) molecule, attached to *3* *phosphate groups*. The adenine nucleotide and ribose molecule are together referred to as *adenosine*. The energy within the ATP \'battery\' is stored in the bonds linking the phosphate groups to adenosine - The phosphate groups are negatively charged and thus repel each other when close together. Lots of energy is needed to hold them together. - The phosphate molecules are added, it \'charges the battery\' - When the final phosphate group is released from the ATP molecule (called *ATP hydrolysis*), the battery\'s \'energy\' can be released, and used as the immediate energy source for cellular work such as muscle contraction. Skeletal Muscle Bioenergetics Video Notes - Energy needed to power muscle contraction comes from ATP - ATP also supplies the energy for all cellular processes, it is the energy currency for the cell - Large amounts of energy are stored in the bonds, holding each phosphate together. These bonds are broken through the adding of water, aka hydrolysis, energy is released to then power cellular processes - ATP + H2O = ADP + Pi + energy - Very little ATP is stored in muscle fibres and there\'s only enough to power muscle contraction for a few seconds. There\'s only about 4g stored in the muscle. For context, a marathon runner needs about 20kg of ATP to complete a race. - ATP needs to replenished at a rate fast enough to fuel muscle contraction. - We have 3 energy systems to replenish ATP - ATP - PC System: short term reserve, uses stored phosphocreatine for fuel. When more ATP is needed to power contraction, the creatine contributes a phosphate molecule to make ADP into ATP. Replenishes ATP rapidly, however, the creatine supply gets used very quickly. - Anaerobic glycolysis: breakdown of glucose. Metabolic pathway converting a glucose molecule into 2 lactate molecules, as well as 2 ATP and 2 NADH molecules. Glucose can be supplied from either blood glucose or stored glycogen. This system doesn\'t need oxygen. This system will only release energy for between 30 seconds - 2 minutes of max exercise. - Aerobic respiration: occurs in the mitochondria, uses oxygen. Important for muscle contraction longer than 30 seconds. Glucose is broken down to form pyruvate \> then enters the mitochondria and goes through the Kreb Cycle and the electron transport chain. This system needs oxygen as it generates more ATP per molecule of glucose, up to 38 ATP molecules. - Aerobic respiration can also breakdown fats, no other system does. When fats are broken down, they need oxygen, they produce much more ATP per molecule but takes longer to break down and uses more oxygen in the process, can yield up to 129 ATP molecules. - Differences in the amount of fat and carbs we store in our bodies and the rate at which they can be used, both have implications for how long they can support ATP production during exercise. - Carb stores can typically supply energy for up to 90 minutes of intense activity. - Fat tissue is the largest energy store in the body, can sustain energy for up to 120hrs at marathon running pace. [Structure and Properties of Metabolic Fuels: Carbs, Fats and Proteins ] - We have fuel stores in our body to give us energy instantly when food is not available. - Along with fuel stored in muscle, we also have fuel stored in other tissues (e.g., in fat stores or in the liver) that can also be 'tapped-into' when required, without having to refuel constantly. - So, to provide skeletal muscle with the energy required to power cellular processes including contraction, the muscle requires fuel that is stored within the muscle itself, but also fuel that comes from sources outside of the muscle cell (i.e., in adipose tissue and the liver). Phosphagen System - Only has enough energy for a few seconds of work - This has a buffering system to let us perform work but also buffer energy supply to demand. - The first buffer is creatine. Our muscles store phosphocreatine to buffer the recycling of ATP quickly. Sports that require rapid and intermittent work need enough creatine. t is a very osmotically active compound and so if we can optimise our muscle creatine content then we can enhance the water content of our muscles meaning fuller muscles. The downside of this of course is that this osmotic activity limits our phospho-creatine storage capacity. - The second buffer is adenylate kinase. This reaction is crucial to maintain ATP levels under energy stress conditions and also is important in producing a primary signal that ramps up glycolytic rate. Before we explain how the ADK reaction works, you should know that it is critically important to maintain ATP concentration higher than ADP concentration - the disequilibrium between these two factors is what allows useful work to be done by the hydrolysis of ATP to ADP and inorganic phosphate (Pi). Think of a water fall driving a turbine - the higher the fall (ATP concentration here represented by the height of the water) the more the turbine will turn. Conversely the lower the turbine (ADP concentration here represented by the height of the turbine from the ground) the more the turbine will turn. However, if the height of the waterfall was to equilibrate (ATP and ADP concentration become equal) with the height of the turbine then the turbine would no longer turn - we would no longer be able to do useful work with the turbine. But we can increase the effectiveness of the turbine in one of two ways, we can increase the height of the water fall, or we can lower the turbine. The same holds true for ATP and ADP, we can maintain the cell\'s energy charge somewhat by removing ADP from the system as much as we can improve the energy charge by adding ATP to the system. This is where the ADK reaction is so beneficial - the ADK reaction removes two ADP molecules from the system for every ATP molecule that it adds back to the system and in this way it maintains the all important ATP:ADP ratio. Importantly it generates a molecule of AMP. AMP is important because it activates the rate limiting step for glycolysis - the enzyme phosphofructokinase is like the tap for glycolysis. If we turn the tap (activate phosphofructokinase) we allow the glycolytic metabolites to flood through generating lactate and recycling ATP as the end product. In this way energy stress signals to glycolysis via the generation of AMP from the ADK reaction that glycolytic flux needs to be ramped up. And as glycolytic flux is ramped up a key metabolite is produced Fructose-1-6-bisphosphate. Fructose-1-6-bisphosphate activates the pyruvate dehydrogenase complex which is rate limiting for the oxidative phosphorylation of carbohydrate. Carbohydrates - Available as the simple sugar glucose or is stored as glycogen - Glycogen consists of up to 50,000 glucose molecules, that: are synthesised from circulating glucose, can be used to increase blood glucose levels when needed and function as immediate reserve of glucose for muscle cells - The amount of carbs stored in the muscle and liver is small in comparison to the amount of fat stored in adipose tissue or muscle. - They are hydrates with carbon attached. - Monosaccharides: single sugar molecules that can\'t be broken down any smaller. Glucose, fructose and galactose. Can be dissolved in water - Polysaccharides: made up of mono and disaccharides. Stored form of glucose in humans - Glycogen is stored in the liver and muscle fibre. Has linear chains of glucose, with 10-14 glucose units and can form little branches. - Muscle glycogen function as an immediate source of glucose for the muscle. - Individual glucose molecule can be removed from the chain as required - Glycogen can be stored and broken down in muscle itself and can hold up to 500g. - Liver stores up to 120g of glycogen but need to be broken down and transferred to the muscle. - Other sources of glucose can come from food. As an energy source, CHOs: - Are stored in limited amounts (\~500 grams) within muscle fibres as glycogen - Circulate within the bloodstream as exogenous (originates outside of the body) glucose derived from food, or from endogenous (originates within the body) sources such as the liver (up to 120 grams of glycogen is stored in the liver) - Produce less energy (ATP) per molecule than fats, but requires less oxygen to metabolise, and generates ATP faster than fat - Can be metabolised either *aerobically* or *anaerobically* (with or without oxygen) - Are used almost exclusively during high-intensity exercise Fats As an energy source for humans,* fat* is available from: - *Triglyceride *droplets stored within the muscle fibre (known as *intramuscular triglycerides* or *IMTGs*) - *Plasma free fatty acids (FFAs)* derived from adipose lipolysis (the breakdown of triglycerides stored in fat tissue) - Lipids are diverse molecules and include fats, oils, waxes, steroids and phospholipids - Make up a large part of structural elements of all cells - Stored at high energy density and give more energy than carbs. - Most fatty acids have between 4 -24 carbons in chain length - Fatty acid chains also differ in saturation. When the carbon chain has the max number of hydrogen atoms bonded, it is said to be saturated with hydrogen atoms. The degree of saturation is important for structure and function of the fatty acid. This will also affect the structural arrange. When there is a double bond, it can cause the chain to bend. Unsaturated fatty acids are more susceptible to bending and oxidation, whereas saturated fatty acids are the opposite - Triglycerides are the storage for fatty acids in the body - The process of fatty acids becoming triglycerides is called esterification - Adipose tissue is the largest energy store in the body. Typically there is enough stored here to produce energy indefinitely. There is up to 300g of fatty acids stored in muscles. - The major advantage of storing energy as lipid is the energy density. The caloric equivalent of fat is 9 kcals/g where as the caloric equivalent of CHO is 4 kcals/g (protein is 4 kcals/g and alcohol is 7 kcals/g). Thus, per gram fat has over double the energy density of CHO. However, when you factor in the water stored with CHO it is actually considerably more. When stored in muscle as glycogen the CHO has a caloric equivalent of \~1 kcal/g, but stored lipid (because it is hydrophobic) doesn\'t store any water and so it is an incredibly efficient method of fuel storage and this is why we invest considerable energy in converting excess CHO into fat stores through *de novo* lipogenesis. - The other advantage of fats is that they contribute to a process know as ketogenesis. Our brains have an obligatory requirement for glucose for survival. The brain needs a minimal amount of glucose but when glucose becomes limiting such as during starvation (after \~72 hours without food glycogen will be depleted) the brain can switch to an alternative fuel - ketones. Under these conditions, when insulin is very low and endogenous stores of CHO have become depleted, the liver will convert partially metabolised fatty acids into ketones which the brain can utilise. The glycerol backbone released from breaking down triglycerides can also be converted to glucose in the liver via gluconeogenesis. - During prolonged starvation or prolonged exercise our muscle protein will be broken down to provide gluconeogenic amino acids for the liver to generate glucose. - This is a great example of how the metabolisms of our body\'s tissues are integrated to support the survival of the whole organism. In a way, the muscle feeds glucose to the brain via releasing amino acids for the liver to generate glucose. This is partly coordinated by the \"FOXO\" family of transcription factors. In muscle the FOXO transcription factors coordinate the breakdown of muscle proteins into amino acids whilst in the liver the FOXO transcription factors coordinate an up regulation of the machinery required to convert amino acids into glucose As an energy source, fats: - Are stored in large amounts in adipose (fat) tissue - IMTGs are stored in relatively small amounts (up to 300 grams) in muscle fibres as small lipid droplets located near mitochondria - Have a high energy density -- they store more energy per molecule than CHO, but require more oxygen and more time to break down - Can only be metabolised *aerobically* (with oxygen) - Used predominantly during low-intensity, long duration exercise, or at rest - The capacity to metabolise fats is limited during moderate-to-high intensity exercise Protein Proteins are found in every cell in our bodies; however, skeletal muscle is the largest storage site of protein that could potentially be broken down and used as a metabolic fuel. - Proteins are commonly used as fuel sources except during extreme prolonged periods of fasting - There is no \'storage\' of proteins. - Skeletal muscle tissue is the single largest source of protein in the body and would be the first thing broken down if protein is needing to be used. - Peptide bonds come together to form a polypeptide chain to then form a protein. - There are many protein structures in the body. - Functional proteins: those that transport chemicals in or out of cells, sit on a cell membrane and recognise chemicals such as hormones. Enzymes are proteins that act as catalysts of chemical reactions, meaning they speed up the process\' - Proteins have specific shapes. - Myoglobin and haemoglobin are proteins whose physiological role is to bind oxygen. There are both similarities and differences between these 2. - Myoglobin has 1 heme group and can bind 1 oxygen and binds more tightly. Present in muscle and acts as a local storage reservoir of oxygen. - Haemoglobin has 4 heme groups, can bind 4 oxygen and binds less tightly. Present in blood and transports oxygen over long distances. - Allosteric regulation: the structure of proteins can be regulated by other molecules. The physical process of binding oxygen by haemoglobin influences its 3D structure. When oxygen is bound to the first sunbit of haemoglobin, subtle changes to the structure of the protein occur. Some important points on proteins: - *Amino acids* are the basic building blocks of proteins - Amino acids are not typically found alone within the cell, and unlike glycogen for CHO or triglycerides for fats, there is no analogous storage form of amino acids in the body - Amino acids are joined together by *peptide bonds*, forming what is known as a *polypeptide chain* - Polypeptide chains form functional proteins such as collagen (which makes up much of the connective tissue in the human body), and all proteins that perform important regulatory and structural functions in the body (e.g., enzymes, hormones, structural proteins) - Importantly, the *sequence of the amino acids* in the polypeptide chain (known as the *primary structure*) determines how the amino acids interact with one another to form bonds that cause the polypeptide chain to fold into a specific shape (known as *secondary* and *tertiary structures*) - The resulting *three-dimensional shape* of the protein determines its function **[Proteins as an energy source]** - Free amino acids can only be accessed by the body via either breaking down functional proteins, or from the diet - Used only as a fuel in extreme circumstances (such as starvation)