Multi-omics Approaches to Human Disease PDF
Document Details
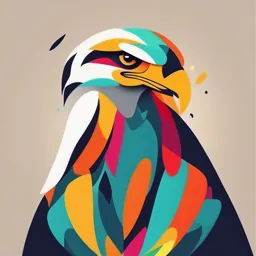
Uploaded by KindlyHawthorn
Dr Rachelle Irwin
Tags
Related
- Multi-Compartment Models PDF
- SNFO 11 Multi-Casualty Incident PDF
- Southern Nevada Fire Operations Multi-Casualty Incident (MCI) Procedures PDF
- Cours 2 Simulation Multi Agent - TChouaki PDF
- LAFD Multi-Casualty Incident Operation Manual PDF
- Pharmacogenomics: A Key Component of Personalized Therapy - 2012 PDF
Summary
This document details an introduction to multi-omics approaches to human disease. The presentation discusses the central dogma, multi-omic approaches, and various 'omics' fields such as genomics, epigenomics, transcriptomics, proteomics, metabolomics, and microbiomics. Applications in diagnostics and research are also covered.
Full Transcript
Multi-omics Approaches to Human Disease Dr Rachelle Irwin In this lecture, we will discuss: The central dogma and its complexities Multi-omic approaches as a concept with application to human disease in terms of diagnostics and research Genomics, Epigenomics, Transcriptomics, P...
Multi-omics Approaches to Human Disease Dr Rachelle Irwin In this lecture, we will discuss: The central dogma and its complexities Multi-omic approaches as a concept with application to human disease in terms of diagnostics and research Genomics, Epigenomics, Transcriptomics, Proteomics, Metabolomics and Microbiomics. Integration of these concepts. Central Dogma basics vs the real picture Beyond the human genome: What is multi-omics? The addition of “omics” to a molecular term implies a comprehensive, or global, assessment of a set of molecules. Each type of omics data, on its own, provides a list of differences associated with the disease The omics field has been driven largely by technological advances that have made possible cost-efficient, high-throughput analysis of biological molecules. Why is the multi-omic approach important:? Compared to single omics interrogations, multi-omics can provide researchers with a greater understanding of the flow of information, from the original cause of disease (genetic, environmental, or developmental) to the functional consequences or relevant interactions. multi-omics also provides a dynamic view over time across different cell and tissue types, as opposed to the largely static snapshot that the genome alone Determines markers of the disease process and the biological pathways between disease and control groups Corner-stone for precision medicine Multi-omics Most mature of omics fields Focuses on identifying genetic variants Genomics associated with disease Investigates all microorganisms of a Focuses on genome-wide given community Microbiomics Epigenomics characterisation of reversible including skin, mucosa, DNA modifications or gut microorganisms associated DNA-related (bacteria/viruses/fungi) proteins DNA methylation Phenotype Histone modifications Simultaneously Metabolomics Transcriptomics Examines genome-wide quantifies multiple small molecules RNA levels – which (fatty acids/amino transcripts are expressed acids/etc) and how much of each is Proteomics expressed Quantifies peptide abundance, modification and interaction Genomics Microbiomics Epigenomics Phenotype Metabolomics Transcriptomics Proteomics 1. Genomics The first omics discipline to appear, genomics, focused on the study of entire genomes as opposed to “genetics” that interrogated individual variants or single genes. Genomics focuses on identifying genetic variants associated with disease, response to treatment, or future patient prognosis. Involves use of genomic information about an individual as part of their clinical decisions and consideration of implications for treatment Identifies an individual’s genetic material – i.e. sequences or mutations specific to that person Natural genetic variation exists in individuals and between individuals. For example, one individual could have normal variation, such as eye colour alongside other genetic differences that affect their diet, response to medication, influence disease and as a result of an inherited genetic condition. Genomic ‘medicine’ Genomic medicine is an emerging medical discipline that involves using genomic information about an individual as part of their clinical care (e.g. for diagnostic or therapeutic decision-making) and the health outcomes and policy implications of that clinical use. Number of citations of the terms "Multiomics" and "Multi-omics" in PubMed Genomic ‘medicine’ throughout the life cycle There are many modalities for genomics to have an impact on clinical care, with entry points for application that span the human life cycle from conception to death. Genetic variation can exist in the germline or somatically, and be associated with Mendelian disorders, age of onset, and more. Advances in sequencing technologies have enabled detection of multiple variants in the genome at one. Genomics technology Applications of Genomics The FDA has cleared >45 human genetic tests and over 100 nucleic acid-based microbial pathogen tests. DNA sequencing is being used to investigate infectious disease outbreaks including: Ebola virus Resistant strains of S. aureus E coli Diagnosing bacterial meningoencephalitis Targeted gene therapy for cystic fibrosis Whole genome sequencing for rare disease cases Pharmacogenomics – how a person will respond to a drug – over 100 FDA-approved drugs with this info on their labels (anti-cancer therapies, anti-viral, analgesics, etc). Summary of Genomics Applications Examples of large genomics initiatives globally Genomics Microbiomics Epigenomics Phenotype Metabolomics Transcriptomics Proteomics 2. Epigenomics Study of reversible epigenetic modifications that affect gene expression without change in the DNA sequence. Reversible processes include: DNA methylation: methyl groups added to DNA sequences Histone modifications: acetyl groups and methyl groups added to histone proteins MicroRNAs: post-transcriptional short non-coding RNAs Chromatin modifiers: alter composition of nucleosomes. Epigenomics and environmental influence Environmental influence can affect epigenetic changes which are inherited by daughter cells Individual differences in epigenetic states, arising from genotype of maladaptive responses to environmental influences (e.g. smoking) can lead to disease Harnessed to provide adjuvant treatment and novel treatment strategies to genomic approaches. Epigenetic disease examples and interplay Changes in genetics can underpin epigenetic changes. Disease examples include: Acute myeloid leukaemia – de novo mutations in DNMT3A DNMT3a overgrowth syndrome (Autosomal dominant inheritance) Prader-Willi syndrome: Deletion of an imprinting control region, which regulates several genes with allele-specific gene inheritance patterns. Epigenetic changes alone can result in disease. Examples include: Hypermethylation of BRCA1 promoter in breast cancer/APC1 gene in lung cancer PTEN activation in prostate cancer via H3K9 methylation and deacetylation miR-18a upregulation in liver cancer Loss of methylation on maternal SNRPN allele assoc. with Prader-Willi syndrome Epigenomics technology Applications of Epigenomics Cancer biomarker Promising biomarker for depression, autism, Alzheimer’s etc. Epigenetic drugs – dynamic and reversible state of interest in drug development such as DNMTi – DNA methyltransferase inhibitors (Vidaza) HMTi/HACi/HDACi – histone methylation/acetylation/deacetylation inhibitors (Vorinostat) Genomics Microbiomics Epigenomics Phenotype Metabolomics Transcriptomics Proteomics 3. Transcriptomics Examines all RNA molecules in the cell, known as the transcriptome Although 3% of the genome encodes proteins, up to 80% is transcribed. Comparison of cell types, or healthy vs diseased cells/in response to treatments, allows for identification of differentially expressed genes. Main approaches for analysis are: Microarrays- quantify a set of predetermined sequences RNA-sequencing – captures all sequences Transcriptomics technology RNA-seq transcriptome analysis of COVID-patien Applications of Transcriptomics Diagnostic and disease profiling by identification of RNA SNPs, allele-specific expression, contributing to disease causal variants RNA sequencing is being used to identify novel virulence factors and infectious disease outbreaks, predict host-pathogen immune reactions/resistance including: Coronavirus (COVID-19) Staph aureus methicillin resistance Dysregulation of non-coding RNAs important for endocrine function, neurodevelopment etc. Implications include diabetes, cancer. Whole genome sequencing for rare disease cases Pharmacogenomics – how a person will respond to a drug – over 100 FDA-approved drugs with this info on their labels (anti-cancer therapies, anti-viral, analgesics, etc). Genomics Microbiomics Epigenomics Phenotype Metabolomics Transcriptomics Proteomics 4. Proteomics The proteome is the overall protein content of a cell. Proteomics involves application of technologies for the identification and quantification of overall protein content. ELISA, Western Blotting, IHC staining are used in proteomics with revolutionalisation by MS-based methods adapted for high-throughput analyses for cells or bodily fluids Affinity purification methods, in which one molecule is isolated using an antibody or a genetic tag, can also be used. MS is then used to identify any associated proteins. Such affinity methods, sometimes coupled with chemical crosslinking, have been adapted to examine global interactions between proteins and nucleic acids (e.g., ChIP-Seq). Proteomic technologies Applications of Proteomics Diagnostics from human urine sample for acute renal failure and chronic kidney disease (LEFT) Biomarker monitoring (E.g. of metabolites) C-reactive protein PSA in prostate cancer Diagnosis and identification of pathogens from biological samples: E. coli C. Difficile H. pylori Genomics Microbiomics Epigenomics Phenotype Metabolomics Transcriptomics Proteomics 5. Metabolomics and applications The metabolome is the complete set of metabolites within a cell or tissue/biological sample at a given time point – these are continuously synthesised, absorbed, degraded interacting with other biological molecules. Metabolomics simultaneously quantifies amino acids, fatty acids, carbohydrates and other cellular products of metabolic reactions. Levels of metabolites affect metabolic functions, out of range metabolites are indicative of disease [urine/blood/seminal fluid] contain highly informative metabolites. Metabolomic technologies Down’s syndrome in maternal serum testing Genomics Microbiomics Epigenomics Phenotype Metabolomics Transcriptomics Proteomics 6. Microbiomics Human bodies consist of different microbial symbionts, from 10 to 100 trillion within a single human. Microbiomics is an emerging discipline, and considers the microorganisms that live on and in humans, and their association with health and disease. Host genetics shape gut microbiome in concert with environmental factors diet and lifestyle. Major factors influencing the composition of the gut microbiome. Host genetic variants influence gut microbiome composition by affecting aspects such as architecture, BMI, diet and immune system activity. GWAS Twin studies have provided great insight into human genetic variation and the microbiome. Dysbiosis in the gut has been associated with multiple diseases including inflammatory bowel disease (IBD) and T2DM. Microbiome technologies mGWAS Microbiome omic data is regressed with host genomic data. Results from mGWAS and hGWAS indicate host- microbiome associations, effect of host-microbiome associations on the phenotype, and provide insight into biological system by giving a better view of the interaction networks that underlie expression of host phenotypes. Applications of Microbiomics Variants in single genes such as LCT, MUC2, NOD2 and FUT2 affect gut microbiome composition. Microbiome genome-wide association studies hold promise for the identification of additional host genetic variants that affect disease progression by perturbing the composition of the microbiome. Development of pre/probiotics and microbiome therapeutics New treatments such as faecal transplants for recurrent C. difficile infection. Monitor drug effects on the microbiome – the relationship between microbiome community composition as a causal or consequential factor of disease. Multi-omics approaches to disease High-throughput multi-omics approaches. Arrows represent interactions between omics layers (e.g. red transcript can be correlated with multiple proteins.