Molecular Genetics Notes PDF
Document Details
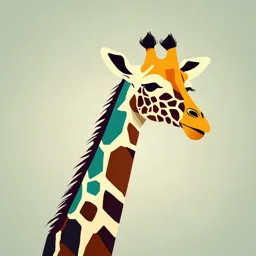
Uploaded by ComelyRisingAction
Universität St. Gallen (HSG)
Tags
Summary
These detailed notes cover the fundamental concepts of DNA and RNA structure, function, and replication. They also explore the processes of transcription and translation, essential for protein synthesis. The notes use clear explanations and diagrams to illustrate key processes.
Full Transcript
Detailed Notes: DNA and RNA 1. Introduction to DNA and RNA Genetic Material Overview: ○ DNA (Deoxyribonucleic Acid): § Primary molecule for storing and transmitting genetic information. § Located in the nucleus (eukaryotes) or nucleoid region (prokaryotes). § Structure: Doubl...
Detailed Notes: DNA and RNA 1. Introduction to DNA and RNA Genetic Material Overview: ○ DNA (Deoxyribonucleic Acid): § Primary molecule for storing and transmitting genetic information. § Located in the nucleus (eukaryotes) or nucleoid region (prokaryotes). § Structure: Double-stranded molecule twisted into a double helix. ○ RNA (Ribonucleic Acid): § Plays a major role in protein synthesis and gene regulation. § Found in both nucleus and cytoplasm. § Structure: Single-stranded molecule. Why DNA and RNA Are Important: ○ DNA stores genetic blueprints. ○ RNA acts as the intermediary, carrying instructions for protein synthesis. 2. Nucleotide Structure Nucleotide Components: ○ Basic unit of DNA and RNA. ○ Each nucleotide consists of: 1. Pentose Sugar: □ DNA: Deoxyribose (lacks one oxygen atom on 2' carbon). □ RNA: Ribose (has a hydroxyl group on 2' carbon). 2. Phosphate Group: □ Gives DNA and RNA their negative charge. □ Links nucleotides via phosphodiester bonds. 3. Nitrogenous Base: □ Divided into: ® Purines (double-ring): Adenine (A), Guanine (G). ® Pyrimidines (single-ring): Cytosine (C), Thymine (T, DNA), Uracil (U, RNA). 3. DNA Structure Double Helix Model: ○ Discovered by Watson and Crick (1953), based on Rosalind Franklin’s X-ray diffraction. ○ Two strands of DNA are antiparallel: § One runs 5' → 3', the other 3' → 5'. ○ Backbone: Alternating sugar (deoxyribose) and phosphate groups. ○ Base Pairing: § A pairs with T (2 hydrogen bonds). § G pairs with C (3 hydrogen bonds). ○ Hydrogen bonds between complementary bases stabilize the helix. Major and Minor Grooves: ○ Structural feature of the double helix. ○ Major groove: Larger space for protein interactions. ○ Minor groove: Smaller space with limited access. 4. RNA Structure Differences Between RNA and DNA: ○ RNA is single-stranded and less stable than DNA. ○ Sugar: Ribose (contains hydroxyl group at 2'). ○ Bases: Uracil (U) replaces Thymine (T). Types of RNA: 1. mRNA (Messenger RNA): § Carries genetic code from DNA in the nucleus to ribosomes in the cytoplasm. § Linear molecule. 2. tRNA (Transfer RNA): § Brings specific amino acids to ribosomes during protein synthesis. § Cloverleaf shape with anticodon region and amino acid attachment site. 3. rRNA (Ribosomal RNA): § Forms ribosome structure, which is the site of translation. § Catalyzes peptide bond formation. 5. DNA Replication Definition: ○ Process of copying DNA to ensure genetic material is passed on. Steps in DNA Replication: 1. Initiation: § Enzyme helicase unwinds and separates the DNA strands by breaking hydrogen bonds. § Topoisomerase prevents overwinding of DNA ahead of the replication fork. § Single-Strand Binding Proteins (SSBs): Stabilize single-stranded DNA. 2. Priming: § Primase synthesizes short RNA primers complementary to the DNA strand. § Primers provide a starting point for DNA polymerase. 3. Elongation: § DNA Polymerase III: Adds complementary nucleotides in the 5' → 3' direction. □ Leading strand: Synthesized continuously toward replication fork. □ Lagging strand: Synthesized discontinuously as Okazaki fragments. § DNA Ligase: Seals gaps between Okazaki fragments by forming phosphodiester bonds. 4. Termination: § Replication ends when forks meet or when replication reaches specific sequences. Semi-Conservative Replication: ○ Each new DNA molecule has one parental strand and one new strand. 6. Base Pairing Rules DNA Base Pairing: ○ Adenine (A) pairs with Thymine (T) – 2 hydrogen bonds. ○ Guanine (G) pairs with Cytosine (C) – 3 hydrogen bonds. RNA Base Pairing: ○ Adenine (A) pairs with Uracil (U). ○ Guanine (G) pairs with Cytosine (C). 7. Differences Between DNA and RNA Feature DNA RNA Sugar Deoxyribose Ribose Strands Double-stranded Single-stranded Stability More stable (no 2' hydroxyl group) Less stable (2' hydroxyl group) Nitrogenous Bases A, T, G, C A, U, G, C Function Genetic storage Protein synthesis Location Nucleus Nucleus and cytoplasm Exercises and Solutions 1. Exercise 1: Identify Differences Between DNA and RNA. ○ Refer to the comparison table above. 2. Exercise 2: Explain Complementary Base Pairing. ○ DNA: § A pairs with T (2 hydrogen bonds). § G pairs with C (3 hydrogen bonds). ○ RNA: § A pairs with U. § G pairs with C. ○ Purpose: Ensures accuracy during replication and transcription. 3. Exercise 3: Label DNA Replication Diagram. ○ Key components: § Helicase: Unwinds DNA. § Primase: Adds primers. § DNA Polymerase: Adds nucleotides. § Ligase: Joins Okazaki fragments. § Leading Strand: Continuous synthesis. § Lagging Strand: Discontinuous synthesis. 4. Exercise 4: Identify DNA and RNA Structures. ○ DNA: Double-stranded with A-T, G-C pairing. ○ RNA: Single-stranded, U replaces T. 5. Exercise 5: Functions of Different Types of RNA. ○ mRNA: Carries genetic instructions. ○ tRNA: Transfers amino acids to ribosomes. ○ rRNA: Forms ribosome and catalyzes peptide bond formation. Summary DNA: Stores genetic information. RNA: Involved in transcription and translation for protein synthesis. DNA replication is semi-conservative, ensuring genetic continuity. Base pairing rules maintain structure and accuracy. Differences between DNA and RNA allow specialization of function. Let me know if you'd like explanations or visuals on any section! Detailed Notes: DNA Replication 1. Key Concept Genetic Information in DNA Can Be Accurately Copied. 2. Cell Division and DNA Importance of Cell Division: ○ Multicellular organisms grow and repair tissues by cell division. ○ DNA must be accurately copied before cells divide to ensure each new cell gets the correct genetic material. Key Processes: 1. Mitosis (nuclear division): § Results in identical daughter cells. 2. Cytokinesis (cytoplasmic division): § Splits the cytoplasm into two. DNA Replication Role: ○ Ensures each daughter cell has the full set of genetic information. 3. Semiconservative Replication: DNA replication follows the semiconservative model: ○ Each new DNA molecule consists of: § One parental (old) strand. § One newly synthesized strand. This ensures accurate copying of genetic material. 4. Meselson and Stahl Experiment Objective: To test the hypothesis of semiconservative replication. Method: 1. Grew E. coli in a medium with heavy nitrogen (^15N) for several generations. 2. Shifted E. coli to a medium with light nitrogen (^14N). 3. Extracted DNA after each generation and analyzed its density using centrifugation. Results: ○ After one generation: DNA had an intermediate density (one old strand, one new strand). ○ After two generations: Two types of DNA appeared: § 50% intermediate density. § 50% light density. Conclusion: ○ Supported the semiconservative model of DNA replication. 5. The Process of DNA Replication Step-by-Step Process: 1. Helicase breaks hydrogen bonds between complementary bases, unzipping the DNA double helix. § This creates a replication fork with two separated strands. 2. Single-Strand Binding Proteins (SSBs): § Stabilize the separated DNA strands to prevent rejoining. 3. Primase: § Adds short RNA primers to the single-stranded DNA. § Provides a starting point for DNA polymerase. 4. DNA Polymerase: § Synthesizes new DNA strands by adding complementary nucleotides in the 5' → 3' direction. § Leading Strand: □ Synthesized continuously toward the replication fork. § Lagging Strand: □ Synthesized discontinuously in short fragments called Okazaki fragments. 5. DNA Ligase: § Seals gaps between Okazaki fragments, forming a continuous strand. 6. Proofreading: § DNA polymerase checks for and corrects errors during replication. Key Points: ○ Replication is bidirectional and occurs at multiple origins of replication in eukaryotic cells. ○ Energy for DNA synthesis is provided by nucleotides (dNTPs). 6. Exercises and Solutions 1. Task 1: When and why does DNA need to be replicated? ○ When: Before cell division (during the S phase of interphase). ○ Why: To ensure each daughter cell gets an identical copy of the genetic material. 2. Task 2: Explain why DNA replication is described as semiconservative. Include a sketch. ○ Answer: § Each new DNA molecule consists of one parental strand and one newly synthesized strand. ○ Sketch: Parent DNA: 5' ------- 3' 3' ------- 5' After Replication: New DNA 1: New DNA 2: 5' ------ 3' | 5' ------ 3' 3' ------ 5' | 3' ------ 5' 3. Task 3: Assume replication was conservative. What would Meselson and Stahl have observed in the first generation? ○ Answer: § All DNA molecules would consist of entirely heavy DNA and entirely light DNA. § There would be no intermediate density observed. 4. Task 4: Function of Key Molecules in DNA Replication: ○ Helicase: Unzips DNA by breaking hydrogen bonds. ○ SSBs: Stabilize single-stranded DNA. ○ DNA Primase: Adds RNA primers to initiate replication. ○ DNA Polymerase: Synthesizes DNA strands by adding nucleotides. ○ DNA Ligase: Joins Okazaki fragments on the lagging strand. 5. Task 5: Compare DNA Replication to a Printer (Key Analogy): ○ Template: Original DNA strand. ○ Ink: Nucleotides. ○ Printer: DNA polymerase. ○ Paper: Newly synthesized strand. 6. Task 6: Label the Diagram of DNA Replication. ○ Terms to label: § Helicase § Primase § Leading strand § Lagging strand § Okazaki fragments § Ligase § Replication fork 7. Task 7: Watch Animation on DNA Replication: ○ Link: https://www.youtube.com/watch?v=teV62zrm2P0 Summary DNA replication follows the semiconservative model, as shown by Meselson and Stahl. Key steps include unwinding the double helix, primer addition, and DNA synthesis by DNA polymerase. The leading strand is synthesized continuously, while the lagging strand is synthesized discontinuously. Accurate replication ensures genetic stability across generations. Let me know if you need further explanations or a visual breakdown of any specific section! Detailed Notes: Proteins and Amino Acids 1. Proteins: Functions and Importance Proteins are essential molecules encoded by genes and constructed from amino acids. Proteins perform a wide range of functions in living organisms and can be categorized into 9 major classes: Class Example Function 1. Enzymes Amylase Catalyze reactions (e.g., digestion of starch). 2. Structural Proteins Collagen Make up tendons and ligaments, provide structural support. 3. Regulatory Proteins Insulin Regulate processes (e.g., blood glucose levels). 4. Transport Proteins Hemoglobin Transport oxygen in the blood. 5. Receptor Proteins Rhodopsin Receive stimuli (e.g., light) and transmit signals. 6. Contractile Proteins Actin, Myosin Enable muscle contraction and movement. 7. Defense Proteins Antibodies Protect against pathogens by recognizing specific molecules. 8. Storage Proteins Ovalbumin Store amino acids for growth and development. 9. Hormones Various Chemical messengers that regulate body functions. 2. Structure of Amino Acids General Structure of an Amino Acid: ○ Amino Group (–NH₂): Positively charged at physiological pH. ○ Carboxyl Group (–COOH): Negatively charged at physiological pH. ○ Central Carbon Atom (α-carbon): Bonds to the amino group, carboxyl group, hydrogen, and R-group. ○ R-Group (Side Chain): Determines the chemical properties of the amino acid. Properties of R-Groups: ○ Polar/nonpolar, charged/uncharged, hydrophilic/hydrophobic. ○ R-groups influence how amino acids interact and fold into proteins. Example: H H O H H O | | || | | || N—C—C—OH + N—C—C—OH → Peptide Bond | | | | H R H R 3. Protein Structure Proteins are polymers of amino acids joined by peptide bonds. The structure of a protein determines its function. Proteins have 4 levels of structure: Primary Structure: Definition: Sequence of amino acids in a polypeptide chain. Determined by the genetic code (DNA). Example: Insulin has a unique amino acid sequence critical for its function. Secondary Structure: Definition: Folding of the polypeptide chain due to hydrogen bonds between the backbone (not R-groups). Two major types: ○ α-Helix: Coiled structure stabilized by hydrogen bonds. ○ β-Pleated Sheet: Folded structure where strands run parallel or antiparallel. Example: Fibrous proteins like keratin (α-helices) and silk (β-sheets). Tertiary Structure: Definition: 3D shape formed by folding secondary structures due to interactions between R-groups. Interactions include: ○ Hydrogen bonds. ○ Ionic bonds. ○ Disulfide bridges (covalent bonds between cysteine residues). ○ Hydrophobic interactions (nonpolar R-groups cluster inward). Example: Globular proteins like enzymes and transport proteins. Quaternary Structure: Definition: Association of two or more polypeptide chains into a single functional protein. Example: Hemoglobin: ○ Consists of 4 polypeptide subunits (2 α and 2 β chains). ○ Each subunit binds to heme, which carries oxygen. 4. Denaturation of Proteins Definition: Loss of protein's tertiary or quaternary structure due to disruption of bonds. Causes: ○ Heat. ○ pH changes. ○ High salt concentration. ○ Chemical agents. Denaturation destroys the protein’s 3D shape and function but does not break peptide bonds. 5. Exercises and Solutions 1. Task 1: Explain the terms primary, secondary, tertiary, and quaternary structure. ○ Primary Structure: Linear sequence of amino acids. ○ Secondary Structure: Local folding (α-helix, β-sheet) stabilized by hydrogen bonds. ○ Tertiary Structure: 3D folding due to R-group interactions. ○ Quaternary Structure: Association of multiple polypeptides into one functional unit. 2. Task 2: Distinguish between tertiary and quaternary structures (use hemoglobin). ○ Tertiary Structure: 3D folding of a single polypeptide chain. ○ Quaternary Structure: Multiple polypeptide chains combine to form a functional protein (e.g., hemoglobin has 4 subunits). 3. Task 3: What happens when a protein is exposed to excessive heat or extremes of pH? ○ Answer: The protein denatures, losing its 3D shape and functionality. 4. Task 4: Which class of protein (fibrous or globular) has mainly structural functions? ○ Answer: Fibrous proteins (e.g., collagen, keratin) provide structural support. 5. Task 5: How many different proteins (short proteins) can be built with only 5 amino acids? ○ Answer: The number of possible combinations is: 205=3,200,00020^5 = 3,200,000 (Each position can be occupied by 1 of 20 amino acids). Summary Proteins are critical molecules that carry out structural, enzymatic, transport, and regulatory roles. Amino acids are the building blocks, with the R-group determining chemical properties. Proteins have 4 structural levels that influence their shape and function: 1. Primary: Sequence of amino acids. 2. Secondary: Local folding (α-helix, β-sheet). 3. Tertiary: 3D shape due to R-group interactions. 4. Quaternary: Combination of multiple polypeptide chains. Denaturation disrupts a protein’s structure, impairing its function. Let me know if you'd like further clarification or visual aids for any section! 😊 Detailed Notes: From DNA to Protein – Protein Synthesis 1. Key Concept Genetic information in DNA can be read and used to make proteins needed by the cell. 2. The Genetic Code Definition: The genetic code is the sequence of bases in DNA that dictates the order of amino acids in a protein. DNA bases include: ○ Cytosine (C), Guanine (G), Adenine (A), and Thymine (T). Translation of genetic information involves: 1. Transcription: DNA is transcribed into mRNA. 2. Translation: mRNA is translated into amino acids by ribosomes. Steps to Protein Synthesis: 1. DNA → mRNA (Transcription): ○ DNA is transcribed into mRNA in the nucleus. ○ mRNA carries the "gene copy" to the ribosome in the cytoplasm. 2. mRNA → Protein (Translation): ○ Ribosomes "read" the genetic code and assemble amino acids in the correct order. ○ Each sequence of three bases on mRNA is a codon. 3. DNA Strands: Sense and Antisense DNA has two strands, but only one encodes the "building plan" for proteins: ○ Sense Strand (Coding Strand): § This strand matches the sequence of the mRNA (except T → U). ○ Antisense Strand (Template Strand): § Serves as the template for mRNA synthesis. mRNA synthesis always runs 5' to 3', complementary to the 3' to 5' antisense strand. 4. Codons and the Triplet Code Codon: A sequence of three nucleotides on mRNA that codes for a specific amino acid. The genetic code is: 1. Degenerate: § Multiple codons can code for the same amino acid (e.g., UUU and UUC → Phenylalanine). § There are 64 possible codons but only 20 amino acids. 2. Universal: § The same codons specify the same amino acids across nearly all organisms. 5. Start and Stop Codons Start Codon: ○ AUG (codes for Methionine) signals the beginning of protein synthesis. Stop Codons: ○ UAA, UAG, UGA: Signal the end of translation. 6. The Genetic Code Table Reading the Table: ○ First base → left column. ○ Second base → top row. ○ Third base → right column. Example: Codon AAG → Lysine. 7. Exercises and Solutions 1. Task 1: Which amino acid is represented by the mRNA codon 5'-AAG-3'? ○ Solution: § From the genetic code table: □ AAG codes for Lysine (Lys). 2. Task 2: Describe the features of the genetic code (universal, degenerate). ○ Universal: § The genetic code is the same across almost all living organisms. § Example: AUG codes for Methionine in both humans and bacteria. ○ Degenerate: § Multiple codons can code for the same amino acid. § Example: UUU and UUC both code for Phenylalanine. Summary Genetic information flows from DNA → mRNA → Protein. The genetic code is universal, triplet-based, and degenerate: ○ Codons: Triplets of nucleotides on mRNA that code for amino acids. ○ Start Codon: AUG. ○ Stop Codons: UAA, UAG, UGA. The sense strand carries the protein-encoding sequence, while the antisense strand is the template for mRNA synthesis. Let me know if you'd like additional clarifications or diagrams! 😊 Detailed Notes: Transcription 1. Key Concept Genetic information in DNA is transcribed into messenger RNA (mRNA) and leaves the nucleus. 2. Overview of Transcription Transcription is the process by which a specific section of DNA is copied into mRNA. mRNA carries the genetic blueprint to the ribosomes in the cytoplasm, where proteins are made. Two Major Steps of Protein Synthesis: 1. Transcription (nucleus): DNA → mRNA. 2. Translation (cytoplasm): mRNA → protein. 3. The Blueprint for Protein Synthesis Genes contain the instructions for making polypeptides. A gene consists of: ○ Exons: Coding sequences that code for amino acids. ○ Introns: Non-coding sequences that are removed before translation. The section of DNA that codes for a specific polypeptide is called a cistron or gene. Triplet Code: DNA bases are read in groups of three nucleotides. ○ Each triplet corresponds to an mRNA codon, which specifies one amino acid. 4. Steps of Transcription Step 1: Initiation Helicase breaks the hydrogen bonds between complementary DNA bases, unzipping the double helix. One strand of DNA (the template strand or antisense strand) serves as the template. ○ The other strand is the coding strand (sense strand) with the same sequence as mRNA (except T → U). RNA Polymerase binds to a specific promoter region on the DNA. Step 2: Elongation RNA polymerase: ○ Reads the DNA template strand 3’ to 5’ direction. ○ Synthesizes mRNA in the 5’ to 3’ direction by adding ribonucleotides complementary to the template DNA. § A pairs with U (instead of T in RNA). § T → A, C → G, G → C. mRNA grows as RNA polymerase moves along the DNA, creating a complementary RNA strand. Step 3: Termination Transcription stops when RNA polymerase reaches a termination sequence (STOP signal). The mRNA strand detaches from the DNA template and leaves the nucleus through nuclear pores. 5. Key Molecules in Transcription Helicase: Unwinds the DNA double helix by breaking hydrogen bonds. RNA Polymerase: Catalyzes the synthesis of mRNA by reading the template strand. Promoter Region: DNA sequence where RNA polymerase binds to start transcription. Terminator: DNA sequence that signals the end of transcription. mRNA: The complementary RNA strand that carries the genetic code to the ribosome. 6. Features of mRNA mRNA is complementary to the template strand and identical to the coding strand (except U replaces T). The mRNA sequence is always written 5' to 3'. mRNA leaves the nucleus through nuclear pores and enters the cytoplasm for translation. 7. Transcription Diagram Key Points Key Diagram Features: ○ DNA double helix unzipped by helicase. ○ RNA polymerase moving along the DNA strand. ○ mRNA being synthesized in the 5' to 3' direction. ○ Termination of transcription when the STOP sequence is reached. ○ Newly formed mRNA exiting the nucleus into the cytoplasm. 8. Example: DNA to mRNA Sequence Template Strand (DNA): 3’-ATCGTACT-5’ mRNA Sequence: 5’-UAGCAUGA-3’ ○ A → U, T → A, C → G, G → C. 9. Exercises and Solutions 1. Task 1: Transcribe the DNA template strand 3’-ATCGTACT-5’. ○ Solution: § Template Strand: 3’-ATCGTACT-5’ § mRNA: 5’-UAGCAUGA-3’ 2. Task 2: Identify the roles of the following molecules: ○ Helicase: Unwinds the DNA double helix. ○ RNA Polymerase: Synthesizes mRNA by adding complementary ribonucleotides. ○ mRNA: Carries genetic information from DNA to ribosomes for translation. 3. Task 3: What happens at the end of transcription? ○ Solution: § RNA polymerase reaches the termination sequence. § mRNA detaches from the DNA and exits the nucleus via nuclear pores. Summary Transcription is the process of copying DNA into mRNA. Steps of Transcription: 1. Initiation: Helicase unzips DNA; RNA polymerase binds to the promoter. 2. Elongation: RNA polymerase synthesizes mRNA (5' → 3') using the template strand. 3. Termination: Transcription stops at the terminator sequence. mRNA leaves the nucleus and carries the genetic code to the ribosome for protein synthesis. Let me know if you need further clarifications or diagrams! 😊 Detailed Notes: Transcription (Continuation or Further Insights) 1. Key Concept Genetic information in DNA is transcribed into messenger RNA (mRNA) and leaves the nucleus. 2. Recap of Transcription Transcription produces mRNA that is complementary to the template strand (antisense strand) of DNA. mRNA is a single-stranded molecule that carries the genetic message to ribosomes for translation. 3. Detailed Steps of Transcription Step 1: Initiation Helicase breaks hydrogen bonds to unwind the DNA double helix. RNA Polymerase binds to the promoter region of the gene: ○ Promoter contains a sequence (e.g., TATA box) that signals the starting point. Only one DNA strand (template strand) is transcribed. Step 2: Elongation RNA polymerase moves along the template strand 3' to 5' direction. RNA polymerase adds ribonucleotides complementary to DNA bases: ○ A → U (in RNA), T → A, C → G, G → C. The mRNA strand is synthesized in the 5' to 3' direction. Step 3: Termination RNA polymerase stops transcription when it reaches a terminator sequence: ○ Sequence signals the release of mRNA. The completed mRNA strand detaches from the DNA. 4. Post-Transcriptional Modifications (Eukaryotes) Pre-mRNA requires modifications before leaving the nucleus: 1. Capping: § A modified Guanine cap is added to the 5' end of mRNA for stability and ribosome recognition. 2. Polyadenylation: § A poly-A tail (50-250 adenine nucleotides) is added to the 3' end for protection against degradation. 3. Splicing: § Non-coding sequences (introns) are removed. § Coding sequences (exons) are joined together by spliceosomes. 5. Diagram Key Points DNA Template Strand: Source of mRNA sequence. mRNA Direction: Synthesized 5' → 3' complementary to 3' → 5' DNA template. Nucleus to Cytoplasm: mRNA exits via nuclear pores for translation. 6. Example of Transcription DNA Template Strand: 3'-ATGCCTAGT-5' mRNA Sequence: 5'-UACGGAUCA-3' 7. Exercises and Solutions 1. Task 1: Transcribe the given DNA template strand: 3’-TACGTTAGC-5’. ○ Solution: § mRNA: 5’-AUGCAAUCG-3’ 2. Task 2: Label the molecules involved in transcription. ○ Key Molecules: § DNA Template Strand: Source of mRNA code. § RNA Polymerase: Enzyme synthesizing mRNA. § mRNA: Complementary transcript of DNA. § Helicase: Unwinds DNA. § Terminator: Signals transcription stop. 3. Task 3: Describe the importance of post-transcriptional modifications. ○ Capping: Stabilizes mRNA and aids in ribosome binding. ○ Polyadenylation: Protects mRNA from enzymatic degradation. ○ Splicing: Removes introns to produce mature mRNA for translation. 8. Summary Transcription is the first step of gene expression where DNA is copied into mRNA. Key Steps: 1. Initiation: RNA polymerase binds to the promoter. 2. Elongation: RNA polymerase synthesizes mRNA complementary to the template strand. 3. Termination: Transcription ends at the terminator sequence. Post-Transcriptional Modifications: Capping, polyadenylation, and splicing ensure mature mRNA stability and functionality. mRNA leaves the nucleus for translation in the cytoplasm. Let me know if further clarifications or diagrams are needed! 😊 Detailed Notes: Regulation of Gene Expression 1. Key Concept To regulate cell metabolism, the synthesis of proteins (enzymes) must be controlled. 2. Gene Switches: The Operon Model Entire Genome: ○ A multicellular organism’s genome contains all the genetic information required to produce proteins. ○ Genes need to be expressed at the right time and in the correct amount to control cell processes. ○ Gene expression: Process of converting DNA information into proteins, regulated at transcriptional and post-transcriptional levels. Operon Model (Example: E. coli and Lactose): ○ Found in prokaryotes; operons regulate the expression of groups of genes responsible for a specific function. Key Components of the Lac Operon: 1. Structural Genes: ○ Code for enzymes needed to metabolize lactose. ○ Example: Enzymes that break down lactose into glucose and galactose. 2. Regulator Gene: ○ Produces a repressor molecule. ○ In the absence of lactose, the repressor binds to the operator and blocks RNA polymerase, preventing transcription. 3. Promoter: ○ A DNA sequence where RNA polymerase binds to initiate transcription. 4. Operator: ○ A DNA region where the repressor binds to block transcription. 5. Lactose (Inducer): ○ In the presence of lactose, lactose binds to the repressor and inactivates it. ○ This allows RNA polymerase to bind to the promoter and transcribe structural genes. Key Mechanisms: When lactose is absent → Repressor binds to operator → Transcription blocked. When lactose is present → Repressor inactivated → Transcription occurs. 3. Tryptophan Operon (Repression Mechanism) Another example of gene switches: ○ Controls genes responsible for tryptophan synthesis. When tryptophan levels are high: ○ Tryptophan (corepressor) binds to the repressor, activating it. ○ The repressor binds to the operator → Blocks RNA polymerase → Transcription stops. Tryptophan acts as a negative feedback inhibitor. 4. Comparison of Gene Regulation Mechanisms Inhibition by Product Activation by Substrate Product activates the repressor molecule. Substrate inactivates the repressor molecule. Genes transcribed only when the product is missing. Genes transcribed only when the substrate is present. Genes switch off when there is enough product. Genes switch off when no substrate is available. 5. Regulation in Eukaryotic Cells Prokaryotic vs. Eukaryotic Regulation: ○ Prokaryotic gene regulation (operons) is simpler. ○ Eukaryotic gene expression involves additional mechanisms like chromatin structure, transcription factors, and epigenetics. Mechanisms of Eukaryotic Gene Regulation: 1. Regulation by Transcription Factors: ○ Transcription factors are proteins that bind to the DNA promoter region to control the rate of transcription. ○ Activators increase transcription; repressors reduce transcription. 2. Histone Acetylation: ○ Histones tightly pack DNA into chromatin, preventing gene expression. ○ Adding acetyl groups (acetylation) to histones loosens chromatin → DNA becomes accessible for transcription. ○ Deacetylation: Tightly packs DNA → Gene expression inhibited. 3. DNA Methylation: ○ Methyl groups (-CH₃) are added to cytosine bases in DNA. ○ Effects of Methylation: § Inactivates genes (silences transcription). § Removal of methyl groups can reactivate genes. ○ Environmental Influence: § External factors (toxins, diet, trauma) can cause methylation changes, affecting gene expression. ○ Methylation patterns can be passed on to future generations (epigenetic inheritance). 6. Epigenetics Definition: ○ Changes in gene activity that do not involve changes in the DNA sequence. Mechanisms: ○ DNA methylation, histone modification, and chromatin remodeling. Impact: ○ Environmental factors (e.g., famine, trauma) can influence gene expression across generations. 7. Exercises and Solutions 1. Task 1: Use the terms to complete the diagram (Figure 2): ○ Components to Label: § mRNA, RNA Polymerase, Repressor, Promoter, Structural Genes, Operator, Regulator Gene, Enzymes, Lactose. Solution: ○ Promoter: Site where RNA polymerase binds. ○ Operator: Region where repressor binds to block transcription. ○ Structural Genes: Genes coding for enzymes. ○ Regulator Gene: Produces repressor protein. ○ Repressor: Protein that blocks RNA polymerase. ○ mRNA: Transcribed product. ○ Lactose: Inducer that inactivates the repressor. 2. Task 2: Complete the Table. Inhibition by Product Activation by Substrate Product activates the repressor molecule. Substrate inactivates the repressor molecule. Genes transcribed only when the product is missing. Genes transcribed only when the substrate is present. Genes switch off when there is enough product. Genes switch off when no substrate is available. Summary Gene Expression: Controlled to regulate cell metabolism and protein synthesis. Prokaryotic Regulation (Operons): ○ Example: Lac Operon (activation by lactose) and Tryptophan Operon (repression by product). Eukaryotic Regulation: Involves transcription factors, histone acetylation, and DNA methylation. Epigenetics: Gene expression can be influenced by external factors and passed to future generations. Let me know if further clarification or diagrams are needed! 😊 Detailed Notes: Mutations 1. Key Concept Mutations are changes in the genetic material (DNA) of a cell or organism. They can occur spontaneously or be induced by external factors (e.g., radiation, chemicals). Mutations can affect genes (small-scale changes) or chromosomes (large-scale changes). 2. Types of Mutations 1. Gene Mutations Involve changes in the nucleotide sequence of DNA. Types of Gene Mutations: 1. Substitution Mutations: § One base pair is replaced with another. § Effect Types: □ Silent Mutation: No change in the amino acid sequence (degenerate code). □ Missense Mutation: Codes for a different amino acid → may alter protein function. □ Nonsense Mutation: Introduces a STOP codon, truncating the protein. Example: § Normal DNA: TAC-GGC-CTA → mRNA: AUG-CCG-GAU → Protein: Met-Pro-Asp § Substitution: TAC-GGC-CTA → TAC-AGC-CTA → Protein: Met-Ser-Asp 2. Insertion Mutations: § One or more nucleotides are added to the DNA sequence. § Causes a frameshift mutation, altering the reading frame of codons. 3. Deletion Mutations: § Removal of one or more nucleotides. § Also causes a frameshift mutation. Mutation Type Effect Example Substitution Changes a single nucleotide. Silent, missense, or nonsense. Insertion Adds nucleotides. Frameshift mutation. Deletion Removes nucleotides. Frameshift mutation. 2. Chromosome Mutations Involve changes to large sections of chromosomes. Types of Chromosome Mutations: 1. Duplication: A segment of a chromosome is copied. 2. Deletion: Loss of a chromosome segment. 3. Inversion: A chromosome segment is reversed. 4. Translocation: A segment of one chromosome is transferred to a non-homologous chromosome. 3. Causes of Mutations Spontaneous Mutations: Occur naturally due to errors in DNA replication. Induced Mutations: Caused by external factors (mutagens): ○ Radiation: UV light, X-rays. ○ Chemicals: Cigarette smoke, pesticides. ○ Viruses: Can insert viral DNA into the host genome. 4. Effects of Mutations Neutral: No effect on protein function (silent mutations). Beneficial: Provides an advantage (e.g., mutation for malaria resistance in sickle-cell anemia). Harmful: Causes diseases or loss of function. 5. Sickle-Cell Anemia: Case Study of a Mutation Cause: ○ A substitution mutation in the gene coding for hemoglobin. ○ DNA Change: GAG → GTG ○ mRNA Change: GAG → GUG ○ Amino Acid Change: Glutamic Acid → Valine. Effect on Hemoglobin: ○ Hemoglobin molecules stick together → Distorts red blood cells into a sickle shape. Symptoms: ○ Reduced oxygen transport. ○ Anemia (low red blood cell count). ○ Pain and organ damage. Evolutionary Advantage: ○ Carriers (heterozygous individuals) have resistance to malaria. ○ Example of a beneficial mutation in certain environments. 6. Mutations and Their Impact on Evolution Mutations provide genetic variation, which is essential for evolution. Natural Selection acts on mutations: ○ Beneficial mutations are passed on to future generations. ○ Harmful mutations are removed through selection. 7. Exercises and Solutions 1. Task 1: Compare gene mutations and chromosome mutations. ○ Gene Mutations: Small-scale changes in nucleotide sequences (e.g., substitution, insertion). ○ Chromosome Mutations: Large-scale structural changes in chromosomes (e.g., duplication, translocation). 2. Task 2: Identify the type of mutation in the following example: ○ DNA Sequence: TAC-GGC-TAA → TAC-GGC-TGA ○ Solution: § Type: Substitution (Nonsense Mutation) § Explanation: TAA (normal STOP) changed to TGA, introducing a STOP codon. 3. Task 3: Explain the evolutionary advantage of sickle-cell anemia in malaria-prone regions. ○ Solution: § Heterozygous individuals (carriers of sickle-cell trait) are resistant to malaria. § Provides a survival advantage in regions with high malaria prevalence. 4. Task 4: Describe the effects of frameshift mutations. ○ Solution: § Frameshift mutations shift the reading frame of codons. § Results in entirely different amino acids downstream → Nonfunctional protein. 8. Summary Mutations are changes in DNA that can be classified into gene mutations (e.g., substitution, insertion, deletion) and chromosome mutations (e.g., duplication, inversion). Causes include spontaneous errors and mutagens (radiation, chemicals, viruses). Effects range from neutral to beneficial (evolutionary advantage) to harmful (e.g., sickle-cell anemia). Mutations are the driving force of genetic variation and evolution. Let me know if you need further clarifications or detailed explanations! 😊 Detailed Notes: Genetic Engineering 1. Key Concept Genetic engineering involves the modification of an organism’s genetic material to achieve desired traits or produce specific products. 2. Definition and Overview Genetic Engineering: The direct manipulation of an organism’s genome using biotechnology. It involves isolating, modifying, and transferring DNA between organisms. Applications include: ○ Medicine: Producing insulin, vaccines, and gene therapy. ○ Agriculture: Developing genetically modified (GM) crops. ○ Industry: Producing enzymes and biofuels. 3. Steps in Genetic Engineering Step 1: Isolating the Desired Gene The target gene (e.g., insulin gene) is identified and isolated using restriction enzymes: ○ Restriction Enzymes: Molecular scissors that cut DNA at specific sequences (recognition sites). ○ Cuts produce sticky ends or blunt ends: § Sticky Ends: Overhanging bases that can pair with complementary sequences. § Blunt Ends: Straight cuts with no overhangs. Step 2: Inserting the Gene into a Vector A vector is a DNA molecule used to carry the target gene into a host cell. Common vectors include: ○ Plasmids: Small, circular DNA found in bacteria. ○ Viruses: Engineered to deliver genes to cells. Ligation: ○ DNA ligase enzyme joins the target gene to the vector DNA. ○ The sticky ends of the gene and vector base-pair, ensuring the gene is inserted. Step 3: Transforming Host Cells Transformation: Introducing the recombinant DNA into host cells. Methods of transformation: ○ Heat Shock: Bacteria are exposed to heat to allow DNA uptake. ○ Electroporation: Electric pulses make cell membranes permeable. ○ Microinjection: Direct injection of DNA into a cell. ○ Gene Guns: Used to shoot DNA-coated particles into plant cells. Step 4: Selecting Transformed Cells Not all host cells take up the recombinant DNA. Marker Genes help identify successful transformations: ○ Antibiotic Resistance Genes: Cells with recombinant DNA survive antibiotic treatment. ○ Fluorescent Markers (e.g., GFP): Transformed cells glow under UV light. Step 5: Cloning and Expression Successfully transformed cells are cloned (replicated) to produce large populations. The target gene is expressed to produce the desired protein or trait. Example: Bacteria producing human insulin for diabetes treatment. 4. Restriction Enzymes and DNA Ligase Restriction Enzymes: ○ Cut DNA at specific sequences. ○ Produce sticky or blunt ends. DNA Ligase: ○ Joins DNA fragments by forming phosphodiester bonds between nucleotides. 5. Applications of Genetic Engineering 1. Medicine Production of Insulin: ○ Human insulin gene is inserted into bacterial plasmids. ○ Bacteria produce large quantities of insulin for diabetic patients. Gene Therapy: ○ Corrects faulty genes in patients (e.g., for cystic fibrosis). 2. Agriculture Genetically Modified (GM) Crops: ○ Crops with traits like pest resistance (e.g., Bt crops) and drought tolerance. Example: Golden Rice produces vitamin A to combat deficiency. 3. Industry Producing enzymes for detergents and food processing. Developing biofuels from genetically modified microbes. 6. Risks and Ethical Issues Risks: ○ Potential allergies or health effects from GM foods. ○ Environmental concerns, such as cross-breeding with wild species. Ethical Issues: ○ Concerns about modifying human embryos. ○ Ownership of genetic information (e.g., patents on genes). 7. Exercises and Solutions 1. Task 1: Complete the diagram for genetic engineering. ○ Steps to Include: 1. Identify and isolate the target gene. 2. Cut the DNA and vector with restriction enzymes. 3. Insert the gene into the vector (ligation). 4. Transform host cells with recombinant DNA. 5. Select transformed cells using marker genes. 6. Clone and express the target gene. 2. Task 2: Describe how restriction enzymes and DNA ligase are used in genetic engineering. ○ Restriction Enzymes: Cut DNA at specific sequences to isolate target genes. ○ DNA Ligase: Joins DNA fragments by forming bonds between nucleotides. 3. Task 3: Explain the role of marker genes in selecting transformed cells. ○ Solution: § Marker genes (e.g., antibiotic resistance or fluorescent proteins) identify cells that successfully took up the recombinant DNA. § Untransformed cells do not survive or lack the marker trait. 4. Task 4: Discuss the benefits and risks of genetically modified crops. ○ Benefits: § Increased yield, pest resistance, and improved nutrition (e.g., Golden Rice). ○ Risks: § Environmental impact, unknown health effects, and ethical concerns. 5. Task 5: Explain the process of producing human insulin using bacteria. ○ Solution: 1. Isolate the insulin gene from human DNA. 2. Insert the gene into a bacterial plasmid using restriction enzymes and ligase. 3. Transform bacteria with the recombinant plasmid. 4. Select transformed bacteria using marker genes. 5. Clone the bacteria to produce insulin. 6. Extract and purify insulin for medical use. 8. Summary Genetic engineering manipulates DNA to produce desired traits or proteins. Key steps: Isolation, vector insertion, transformation, selection, and expression. Applications include medicine (insulin, gene therapy), agriculture (GM crops), and industry (biofuels, enzymes). Tools like restriction enzymes and DNA ligase enable precise DNA manipulation. Ethical and environmental concerns must be carefully considered. Let me know if you need further explanations or visuals for any part! 😊