Molecular Pathology - Marino PDF
Document Details
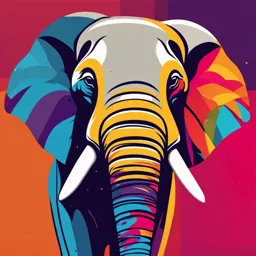
Uploaded by WiseChalcedony7058
Tags
Summary
This document provides a detailed overview of molecular pathology, focusing on cell injury and cell death mechanisms, with examples of various causes and resulting effects.
Full Transcript
MOLECULAR PATHOLOGY – MARINO Cell’s damage can be a consequence of: Oxygen deprivation Physical agents...
MOLECULAR PATHOLOGY – MARINO Cell’s damage can be a consequence of: Oxygen deprivation Physical agents Chemical agents and drugs Infectious agents Immunologic reactions Genetic abnormalities Nutritional imbalance STEPS LEADING TO CELL DEATH 1) Reversible cell injury: early functional and structural alterations; correctable if the dangerous stimulus is removed. a. Generalized swelling of the cell: caused by the influx of water caused by the failure of ATP-dependent Na-K pump (due to the depletion of ATP from oxygen deficiency, or mitochondrial damage by radiations or toxins) b. Fatty changes: in organs involved in lipid metabolism (liver) the injury disrupts metabolic pathways with accumulation of triglycerides. As well known, liver cells are involved in all the metabolic pathways and their damage can lead to multiple diseases such as cirrhosis (late-stage liver disease characterized by scarring (fibrosis) of the liver), mostly caused by ethanol toxicity. Presence of myelin figure à phospholipids accumulation derived from damaged cellular membranes. 1 2) Point of no-return: dependent on the adaption capability of the cell, the type and the duration of damage applied on those cells, as well as on the organelles that get involved during the injury. For example, fibroblasts are much more resistant than neurons or myocardial cells; however, over a certain extent, their power of regeneration fails. 3) Irreversible stage: membranes, cytoskeleton and DNA are the next sites of cellular damage. The misfolding of proteins is a sign of advanced damage à unpaired function NOTE FOR THE FOLLOWING TOPICS: ROS (reactive oxygen species; oxygen free radicals, hydrogen peroxide) are the waste products of the oxidative metabolism (taking place in the mitochondria) in physiological conditions are produced at low-level and can be discarded, avoiding any potential damage. However, if a cell is injured and we have a mitochondrial injury (due to hypoxia, toxic injury, or mitochondrial aging) we will have oxidative stress, leading to an increase of intracellular ROS The electron transport chain is not necessarily couples to ATP generation; indeed thermogenin/uncoupling protein-1 (UCP-1), a hydrogen ion transporter, can dissipate the proton gradient, uncoupling it from oxidative phosphorylation, allowing the rapid substrate oxidation without ATP synthesis, leading tissues with high levels of UCP-1 to generate heat. This occurs in brown fat and is important especially in newborns (in which brown adipose tissue is about 5% of the body mass) to avoid hypothermia. Recently, the reactivation of brown fat as a technique to reduce obesity and treat metabolic syndromes (involving the accumulation of white fat in visceral organs) had been studied. In oxidative phosphorylation, the substrates are burned to CO2 and H2O, leaving no carbon moieties to use for building lipids and proteins. In Warburg effect, proliferative cells preferentially use glycolysis for respiration, instead of oxidative phosphorylation, utilizing the intermediates to convert them into new lipids, amino acids and proteins, and nucleic acids (important for supporting rapid cell growth and division). Thus, proliferative cells carefully balance energy production with the need for metabolic intermediates to support their growth demands and, in pathological conditions, cancerous cells can take advantage of the latter property to proliferate in the organism. Tumor cells are more related to anaerobiosis but in general this process can occur both in aerobic and anaerobic conditions, also to repair the cell after damage. Mitochondrial role in cell death Both in apoptosis and in necrosis, cell death starts from mitochondria due to the formation of pores at the level of the membrane à electron flux impaired à ¯ATP During apoptosis we have the integration of proapoptotic and antiapoptotic effector signals. A net proapoptotic signal results in mitochondrial outer membrane permeabilization (MOMP), which is crucial for initiating cell 2 death. MOMP leads to the release of cytochrome c from the mitochondria into the cytosol, where it forms the apoptosome. This complex then activates the caspase cascade, a series of proteolytic enzymes that execute the cell death process. If this programmed cell death is suppressed (or we have too many antiapoptotic effectors), it can contribute to the development of cancer, as damaged or dysfunctional cells persists. On the other hand, an excessive activation of apoptosis (or lack of antiapoptotic effectors) can lead to autoimmune diseases or neurodegenerative disorders, such as Alzheimer’s disease. Apoptosis prevents the development of malignant clonal cells and it is our defense against viruses, which can duplicate themselves inside our cells (apoptosis eliminate the viruses by suiciding the cells). N.B. Apoptosis presents NO INFLAMMATION, which is cauded by DAMPs For example: in case of ischemia, we observe inflammation in myocardial tissue thanks to DAMPs which, not only cause inflammation but are released in circulation in order to be biomarkers for myocardial damage. After a mitochondrial damage from external cellular injury (toxin, ischemia, trauma) we have the formation of mitochondrial permeability transition pores (MPTP) in the outer membrane, which starts necrosis. Consequently, we will have the loss of the proton gradient (¯ATP) à cell dies In this type of cell death, membrane is abruptly destroyed, leading to inflammation, while during apoptosis the discarded material is packed in vesicles to be phagocyted. Consequences of mitochondrial dysfunction cytosolic [Ca2+] à ATP depletion will cause the non-proper functioning of the pump, leaving the calcium free to enter and move across the cytoplasm and activate different reaction, degrading cell components. Ca2+ activate enzymes such as ATPases, phospholipases, proteases, endonucleases, that can lead to cell injury and death. Calcium is a pro-apoptotic signal. ROS: they amplify the damage through other cell components; indeed, as they are so reactive, they interact with the lipids of the membrane and secondly with proteins and nucleic acids. Oxygen deprivation Toxins Mutations in mitochondrial genes ¯ATP and depletion: frequently associated with both hypoxia and toxins (cyanide) MPTP: loss of membrane potential, failure of oxidative phosphorylation and progressive ATP depletion ATP reduction of 5-10% of normal levels affects critical cellular systems à the activity of the plasma membrane energy-dependent sodium pump (Na+, K+-ATPase) is reduced à sodium enters and accumulates inside cells with osmotically driven water à cell swelling and ER dilation (due to the accumulation). 3 With ATP depletion cellular energy metabolism is altered: ¯ O2 and nutrients (ischemia) à decrease in oxidative phosphorylation and of ATP Stimulation of phosphorylase and phosphofructokinase activities, with an increase of glycogenolysis and glycolysis to generate ATP through metabolism of glucose derived from glycogen o Glycogen stores are rapidly depleted à anaerobic glycolysis à accumulation of lactic acid and Pi (from the hydrolysis of phosphate esters) à ¯ intracellular pH à ¯ activity of cytosolic enzymes (damaged by acidosis) ATP depletion reduce also the protein synthesis: Due to prolonged or worsening depletion, structural disruption of the protein synthetic apparatus occurs (detachment of ribosomes from rER) protein misfolding This will cause an irreversible damage to mitochondrial and lysosomal membranes à leakage of their enzymes in the cytoplasm à activation of acid hydrolases in the acidic pH (RNases, DNases, proteases, phosphatases, glucosidases, whose function is to degrade à NECROSIS DNA damage: irreversible stage Damage to nuclear DNA activates sensors that triggers p53-dependent pathways (p53 is an onco-suppressor gene). P53 will stop the cell cycle to activate a group of genes devoted to DNA repair and, if the repair is not possible, another group of genes devoted to activation of apoptosis will be involved. When, during cancer, mutation impairs p53 or the genes involved in DNA repair/apoptosis, the tumor acquire an advantage in terms of proliferation. MDM2 is the normal inhibitor of p53 which activates itself when the signals coming from the damage are strong. ROS and DNA damage ROS are produced not only in oxidative phosphorylation but also in response by drugs, UV light, ionizing radiation or other components that may be absorbed by the environment (smoking or air pollution). These chemical species have a single unpaired electron in an outer orbit and, for this reason, are highly reactive and attack (and modify) proteins, lipids, carbohydrates, nucleic acids, with damage of cell membranes and nuclei. Molecules that react with free radicals are themselves converted into free radicals, propagating the damage (autocatalytic reactions). ROS are implicated in a wide variety of pathological process, including cell injury, cancer, aging, and some degenerative diseases, such as Alzheimer’s. They are also produced in large amounts by activated leukocytes, particularly neutrophils and macrophages, during inflammatory reactions aimed at destroying microbes and cleaning up dead cells and other unwanted substances. 4 SUMMARY ABOUT MOST IMPORTANT ROS Superoxide anion: produced by oxidative phosphorylation Hydrogen peroxide: very common by-product of reactions inside cells Hydroxyl radical Peroxynitrate PHAGOCYTOSIS ROS are also useful to destroy pathogens, indeed they are produced during phagocytosis to destroy microbes, clean up dead cells and unwanted substances. The cells in charge of phagocytosis are the macrophages (resident in tissue) and neutrophils (present in peripheral blood and are the most numerous types of leukocytes, making the 60%). During this process, we will have an increase in O2 consumption (respiratory burst) and the enzymes catalyzing ROS production are activated (e.g., NADPH oxidase). NADPH oxidase catalyze the production of superoxide, which dismutase to hydrogen peroxide. This last one will react with chloride producing hypochlorous acid that, with ROS, are lethal for bacteria (process on the images on the right). This overall reaction is also the one responsible for the yellowish/whitish substance in tissue called pus (color is due to the ROS presence). 5 Activation of the inflammation in response to DAMPs or pumps as LPS. Thanks to the TLR4 (toll like receptor 4) there is the activation of NF-kB, transcriptional activator of genes involved in inflammation; then follows the activation of enzymes involved in phagocytosis and the production of cytokines. CHRONIC GRANULOMATOUS DISEASE Immune-deficiency disease involving mutations in the NADPH oxidase genes. Patients are not able to kill phagocyted microbes, producing diffuse granuloma due to the pathogens surviving inside the phagosome. The inflammation becomes chronic. GENERATION OF FREE RADICALS 1. Metabolic processes à reduction-oxidation reaction 2. Absorption of radiant energy à ionizing radiation can hydrolyze water into OH and H free radicals 3. Inflammation à phagocytosis (NADPH oxidase) 4. Enzymatic metabolism of exogenous chemicals or drugs 5. Transition metals: can donate or accept free electrons during intracellular reactions and catalyze free radical formation, as in Fenton reaction FENTON REACTION: transitional metals (iron, cupper) have a special oxygen transfer property, which improve the use of hydrogen peroxide generating highly reactive hydroxyl radicals ( OH). Nowadays, it is used to treat a large variety of water pollution such as phenols, formaldehyde, pesticides, rubber chemicals. Fenton reaction is also used to reduce toxicity, improve biodegradability, remove odor and color, destruction of resin in radioactive contaminated sludge 6 NITRIC OXIDE (NO) Important chemical mediator generated by endothelial cells, macrophages, neurons during inflammation, produced by iNOS in response to an injury. Can act as a free radical and may also be converted to highly reactive peroxynitrite anion (ONOO-). It is a powerful vasodilator, acting on muscle cells surrounding the vessels, inducing their relax. During acute inflammation, regarding the vessels, we have: Vasodilation à to allow more amount of blood to arrive to the site of inflammation (thanks to NO, histamine, and cytokines) Increased permeability REMOVAL OF FREE RADICALS The physiological amount of ROS produced by metabolism is neutralized by antioxidant defense like vitamin E or A, as well as ascorbic acid and glutathione in the cytosol. Antioxidants either block free radical formation or inactivate (i.e., scavenge) free radicals. Most well known: Catalase: active in peroxisomes; decomposes H2O2 in O2 and H2O Superoxide dismutase (SODs); convert O2- in H2O o Manganese-SOD: localized in mitochondria o Copper-zinc-SOD, found in the cytoplasm Glutathione peroxidase: it is the biomarker for checking the capability of a cell to prevent ROS injuries. o The intracellular ratio GSSG/GSH (oxidized to reduced glutathione) reflects the oxidative state of the cell and is an important indicator of the cell’s ability to detoxify ROS) Moreover, cells have proteins for the storage and transport for metals to prevent them from participating in reactions that generate ROS (e.g., transferrin, ferritin, lactoferrin, and ceruloplasmin). In hemolytic anemia we have a deficiency of glucose-6-phosphate dehydrogenase (G6PDH) which do not allow to neutralize ROS, resulting in hemolysis. Why? à G6PDH is critical in the PPP, responsible for producing NADPH. NADPH is necessary to maintain glutathione in its reduced form (GSH) to neutralize ROS. When we have a G6PDH deficiency we have a reduced production of NADPH, which impairs the ability of cells – particularly RBCs – to detoxify ROS. This oxidative stress weakens RBCs and makes them more susceptible to destruction by the spleen (extravascular hemolysis) while, in severe cases, intravascular hemolysis can also occur (RBCs rupture directly in the bloodstream due to the oxidative stress). Symptoms include fatigue, jaundice (due to bilirubin from RBC breakdown; it is the first sign in a few hours), dark urine, and in severe cases, shortness of breath or rapid HR. 7 PATHOLOGIC EFFECTS OF FREE RADICALS 1. Lipid peroxidation in membranes: double bonds in unsaturated fatty acids of membrane lipids are attacked by ROS (OH). The lipid-free radical interactions yield peroxides, which are themselves unstable and reactive, with propagation of membrane damage. 2. Oxidative modification of proteins: covalent protein-protein cross-links (disulfide bonds), and oxidation of the protein backbone. May also damage the active sites of enzymes, disrupt the conformation of structural proteins, and enhance proteasomal degradation of unfolded or misfolded proteins. 3. Damages in DNA: single- and double-strand breaks in DNA, cross-linking DNA strands, and forming adducts. Oxidative DNA damage has been implicated in cell aging and in malignant transformation of cells. ER STRESS Unfolded protein response (UPR) Misfolding of proteins represent a late phase of the damage for the cells and the accumulation of them – exceeding the capacity of the ER to edit and degrade them – leads to the ER stress response. The failure to correct the overload can trigger cell death through apoptosis. Chaperones in the ER control the proper folding of newly synthesized proteins, and misfolded polypeptides are shuttled into the cytoplasm where they are ubiquinated and targeted for proteolysis in proteasomes. What are the stressors? Mutations ¯capacity to correct misfolded protein à aging Viral infections: viral proteins are synthesized in such large quantities that they overwhelm the quality control system of proper folding demand for secretory proteins (insulin in insulin-resistant states in diabetes type 2) Changes in intracellular pH and redox state Moreover, protein misfolding is thought to be the causative cellular abnormality in several neurodegenerative disorder (e.g. Alzheimer) due to the stimulus of apoptosis that this stress gives. 8 UNFOLDED PROTEIN: mutation of CFTR in cystic fibrosis In mutant cystic fibrosis transmembrane regulator (CFTR) gene, a codon deletion leads to the absence of a single amino acid (Phe508) which results in its misfolding, ER retention and catabolism and therefore reduced surface expression. The resulting abnormal channel breaks down shortly after it is made, so it never reaches the cell membrane with failure in transport chloride ions. Other examples of diseases correlated to misfolding of proteins 9 Mechanisms of cell injury – clinical examples 1) Ischemia (and damage due to reperfusion): ¯O2 and ¯nutrients (HYPOXIA INDUCED BY A REDUCTION OF BLOOD FLOW) in cells for several reasons (e.g., embolus, thrombus and atherosclerotic plaques) leading to: a. ¯ATP and pump impairment b. Swelling generation c. Ca2+ movement d. consumption of glycogen stores e. Shifting to anaerobic metabolism due to ¯O2 After a while the anaerobic generation fails due to the deficiency of glycolytic substrates à (no O2 – no glucose) f. Chromatin damage g. Alterations in protein synthesis (final stage) Ischemia causes more rapid and severe cell and tissue injury than hypoxia Mammalians have a defense mechanism against hypoxia which involves the hypoxia-inducible factor-1 (HIF-1): Promotes new blood vessels formation through stimulation of § FGF (fibroblast growth factor) § VEGF (vascular endothelial growth factor) § EPO: hormone stimulating bone marrow to produce erythrocytes. This can take place only when hypoxia/ischemia is set in time and not in acute way. The time of setting is different among different types of cells (e.g., muscle cells are more resistant compared to others). Stimulates cell survival pathways through: § P53 § Cyt C Enhance glycolysis through: § GLUTs § Glycolytic enzymes Nowadays, there are still no reliable therapeutic approaches for reducing the injurious consequences of ischemia in clinical situations. The most useful in ischemic (and traumatic) brain and spinal cord injury is the transient induction of hypothermia (lowering the core body temperature to 92ºF/33,33ºC). This treatment reduces the metabolic demands of stressed cells by reducing the production of ROS. It also decreases cell swelling, and inhibits the host inflammatory response (caused by production of DAMPs during necrosis, which are able to activate the innate immune system). Hypoxic brain damage We have a depolarization of the neuronal membrane with the release of glutamate into the extracellular space. The excessive activation of the glutamate membrane receptors leads to the activation of Ca channels, thus further increasing the intracellular concentration of calcium itself. Persistent activation of glutamate receptors is considered an important mediator in the process that leads to the death of brain cells. 10 Neonatal brain injury Hypoxic-ischemic encephalopathy (HIE) is a permanent neonatal brain injury caused by oxygen deprivation and/or limited blood flow to the brain at or near the time of birth. When this happens, brain cell dies off in a cascade reaction, causing widespread damage. In the majority of cases, HIE is preventable through timely delivery. Doctors may limit the long-term damage caused by HIE by performing hypothermia treatment (also known as therapeutic hypothermia or brain cooling). If doctors suspect that a baby has HIE, it is mandatory that they provide hypothermia treatment. Other terms for HIE are perinatal asphyxia, birth asphyxia, intrapartum asphyxia, and neonatal encephalopathy Ischemia-Reperfusion (IR) injury Restoration of blood flow to ischemic tissues can promote recovery of cells if they are reversibly injured but can also exacerbate cell injury IR can cause oxidative stress after reoxygenation, indeed we will have an increased generation of ROS and RNS produced in reperfused tissue as a result of incomplete reduction of oxygen in leukocytes, and in damaged endothelial cells and parenchymal cells. Impairment of cellular antioxidant defense mechanisms during ischemia may sensitize cells to free radical damage. During ischemia we have a ¯ATP and catabolism of AMP (from ATP degradation due to the necessity of having energy available). 1. AMP can be deaminated to IMP (inosin monophosphate) or hydrolyzed directly to adenosine 2. Adenosine is deaminated to inosin (thanks to the enzyme adenosine deaminase) 3. Inosine: degraded to ipoxanthine through the removal of one ribose molecule (catalyzed by purine nucleoside phosphorylase) 4. Ipoxanthine is oxidized to xanthine and then to uric acid (last product of purine catabolism in humans) through the enzyme xanthinie oxidase (XO) 11 Oxidative stress after reperfusion – Xanthine Oxidase During ischemia, due to the decrease of O2 the enzyme xanthine oxidase cannot complete the conversion of ipoxanthine to uric acid. However, when O2 is restored thanks to reperfusion the xanthine oxidase can again oxidize ipoxanthine to xanthine and then to uric acid. As a side effect, we will have a ROS from damaged mitochondria for the activation of oxidase enzymes; these can cause oxidative damage to tissues, worsening the inflammation and the cellular damage. However, a drug used is allopurinol (used for the long-term treatment and prevention of gout) to inhibit XO reducing hypoxanthine in xanthine (and the latter in uric acid) and, so, prevent uric acid accumulation. Intracellular Calcium Overload due to IR Intracellular and mitochondrial Ca2+ overload begins during acute ischemia (for impairment of Ca-ATP pump due to ATP depletion) and it is exacerbated during reperfusion (which increase the blood flux of Ca2+) due to influx of calcium from cell membrane damage and ROS-mediated injury to SR. Calcium overload favors opening of the MPTP with resultant ATP depletion à intracellular Ca2+ causes further cell injury pH correction after IR With reperfusion, lactate is removed from the interstitium, while a greater amount of H+ is still within the cytoplasm: this gradient activates the Na+/H+ exchange with an influx of Na+ inside the cell, with liquid retention. Activation of Na+/Ca2+ exchange (if Na+/K+-ATP pump is impaired) with influx of Ca2+ inside Restoration of intracellular pH may activate MPTP with lost of H+ ions that flow out of mitochondira with dissipation of the proton gradient, uncoupling it from oxidative phosphorylation (¯ATP) 12 Inflammation due to IR injury Ischemic injury induce inflammation as result of danger signals (DAMPs – damage-associated molecular patterns) released from dead cells, cytokines secreted by resident immune cells such as macrophages, and increased expression of adhesion molecules by hypoxic parenchymal and endothelial cells. All these molecules recruit circulating neutrophils arriving with reperfusion to damaged tissue (inflammation cause additional tissue injury; why? à because neutrophils moving out of the blood vessels and into the tissue, release enzymes and ROS to kill any perceived threats; all of this can worsen tissue damage ì, because neutrophils inadvertently harm surrounding cells and tissue structures). The role of neutrophil influx in reperfusion injury has been demonstrated experimentally by the beneficial effects of antibodies that block cytokines or adhesion molecules, preventing neutrophils from entering the damaged tissue, reducing the additional damage that they can create. Activation of the complement system: IgM antibodies have a propensity to deposit in ischemic tissues. When blood flow is resumed, complement proteins bind to the deposited antibodies (both IgG and IgM can activate complement proteins, but IgM are more powerful) triggering a complement cascade, which exacerbate cell injury and inflammation. 2. Chemical (toxic) induced cell injury: Direct toxicity: by combining with critical molecular components o Mercuric chloride poisoning: mercury binds to the sulfhydryl groups of cell membrane proteins, with increased membrane permeability and inhibition of ion transport. The greatest damage is usually to the cells that use, absorb, excrete, or concentrate the chemicals (GI tract and kidney) § Mercuric chloride can produce a range of toxic effects, including corrosive injury, severe GI disturbances, acute renal failure, circulatory collapse, and eventual death. o Cyanide: bloc mitochondrial cytochrome oxidase inhibiting oxidative phosphorylation § Can be found in small amounts in almonds for example, which contain amygdalin that is broken down in the intestine, by the bacterial flora, releasing cyanide. § Symptoms of intoxication appear immediately in the event of inhalation while, if cyanide has been ingested, they appear within a few tens of minutes or more (depending on the state of filling of the stomach) o Several antineoplastic chemotherapeutic agents and antibiotics. 13 Indirect toxicity by conversion to toxic metabolites of substances not biologically active in their native form but must be converted to reactive toxic metabolites. o Modification by the cytochrome p-450 mixed-function oxidases in the sER of the liver and other organs The toxic metabolites cause membrane damage and cell injury mainly by formation of free radicals and subsequent lipid peroxidation; direct covalent binding to membrane proteins and lipids may also contribute CCl4, once used in the dry-cleaning industry, is converted by cytochrome p-450 to the highly reactive free radical CCl3, which causes lipid peroxidation and damages many cellular structures. Analgesic drug acetaminophen is also converted to a toxic product during detoxification in the liver, leading to cell injury. LIVER is the major target of drug toxicity. Toxic liver injury is often the major limitation to drug therapy. Summary 14 AGING Some signs of accelerated aging are evident in genetic human diseases, putting in result the role of some genes In aging. One of these diseases is Hutchinson-Gilford progeria syndrome. No genes are known up to know whose primary function is to program the pace of aging in human, but a lot of genes associated with premature aging are well known, as a secondary effect in their primary function. Is known that aging is caused by accumulation of cellular and molecular damage from the exposure to exogenous influences (physical, chemical, biological) but also from cell intrinsic (ROS) and environmental mechanisms, which can impair the integrity of nuclear and mitochondrial DNA. Importance of DNA repair in the aging process Werner syndrome à the defective gene product is a DNA helicase involved in DNA replication and repair à the defect causes rapid accumulation of chromosomal damage thay may mimic some aspects of the injury that normally accumulates during cellular aging à premature aging. Genetic instability in somatic cells Ataxia-telangiectasia (A-T) à caused by a defect in the ATM gene, which is involved in the recognition and repair of damaged DNA Bloom syndrome à BLM gene products belongs, including TOP3A, which encodes the type I topoisomerase, topoisomerase 3 alfa. Hutchinson-Gilford progeria syndrome à genetic syndrome characterized by a point mutation in the gene of laminin A (LMNA gene) which creates an abnormal LMNA causing the cell membrane to be unable to support an adequate structure Recently, it has been found that rare pleiotropic genetic disorders, A-T, Bloom syndrome and Nijmegen breakage syndrome (NBS) are characterized by immunodeficiency, extreme radiosensitivity, higher cancer susceptibility, premature aging , neurodegeneration and insulin resistance. Moreover, they all possibly have as a common denominator chronic oxidative stress caused by endogenous ROS overproduction and impairment of mitochondrial homeostasis. Replicative senescence All normal cells have a limited capacity for replication, and after a fixed number of divisions they become arrested in a terminally nondividing state. Aging, indeed, is associated with progressive replicative senescence of cells. But why they arrest in this nondividing state? Due to the progressive shortening of telomeres. Telomeres are short repeated sequences of DNA at the ends of linear chromosomes, important for ensuring the complete replication of chromosome ends and for protecting the ends from fusion and degradation. When somatic cells replicate, a small section of the telomere is not duplicated, and telomeres become progressively shortened. As the telomeres become shorter, the ends of chromosomes cannot be protected and are seen as broken DNA, which signals cell cycle arrest. Telomere length is maintained by nucleotide addition mediated by the enzyme telomerase, expressed in germ cells and at low levels in stem cells, but absent in most somatic tissues. In immortalized cancer cells, telomerase is reactivated and telomere length is stabilized, allowing the cells to proliferate indefinitely. 15 Activation of tumor suppressor genes The CDKN2A locus encodes for p16 or INK4a and is correlated with chronologic age in virtually all human and mouse tissue examined. By controlling G1- to S-phase progression during the cell cycle, p16 protects cells from uncontrolled mitogenic signals and pushes cells along the senescence pathways. In addition to p16, also p53 and p21 are important for controlling DNA erroring and stopping the cell cycle and induce apoptosis. All of these are collectively known as the DNA-damage response (DDR). Defective protein mechanism The maintenance of proteins in their correctly folded conformations is mediated by chaperones. Degradation of misfolded, damaged, or unneeded proteins is mediated by the autophagy-lysosome system and ubiquitin- proteasome system. There is evidence that both normal folding and degradation of misfolded proteins are impaired with aging. Mice deficient in chaperones age rapidly Mice that overexpress such chaperones are long-lived Rapamycin (inhibitor of mTOR) promotes autophagy, increases the lifespan of middle-aged mice AUTOPHAGY It involves the delivery of cytoplasmic materials to the lysosome for degradation. It is an evolutionarily conserved survival mechanism whereby, in states of nutrient deprivation, starved cells live by cannibalizing themselves and recycling the digested contents. Moreover, it is implicated in many physiological states (aging) and pathological processes. Dysregulated nutrient sensing Two major pathways are involved: 1. Insulin and Insulin-like growth factor (IGF-1): produced in many cell types (in response to GH). IGF-1 informs cells of the availability of glucose, promoting an anabolic state as well as cell growth and replication. IGF-1 signaling has multiple downstream targets; mTOR (molecular target of rapamycin) 2. Sirtuins: a. Family of NAD-dependent protein deacetylases, important to adapt bodily functions to environmental stresses, including food deprivation and DNA damage b. Promote the expression of genes whose products increase longevity: inhibit metabolic activity, reduce apoptosis, stimulate protein folding, and counteract the harmful effects of ROS c. Increase insulin sensitivity and glucose metabolism, and may be targets for the treatment of diabetes By introducing caloric restriction, we reduce the signaling intensity of the IGF-1 pathways and increase sirtuins: Attenuation of IGF-1 signaling leads to lower rates of cell growth and metabolism and possibly reduced cellular damage. This effect can be mimicked by rapamycin (inhibitor of mTOR à lower production of misfolded protein à increased longevity). An increase in sirtuins, contribute to metabolic adaptations of caloric restriction and promote genomic integrity by activating DNA repair enzymes through diacylation. As an overall result, caloric restriction increases longevity. 16 Is important to know that everything that stimulates proliferation, nutrients and metabolism activate the metabolism and indirectly produce mediators of damage (ROS and DNA damage) that accumulate in our cells (telomere attrition has not to be forgotten because even if is not dangerous due to its physiological role, it is still involved in aging). These mediators, by time, may be risk of factors for cancer, cardiovascular and neurodegenerative diseases. Of course, on the other hand, DNA repair and protein homeostasis counteract aging. Summary 17