Pharmacokinetics PDF
Document Details
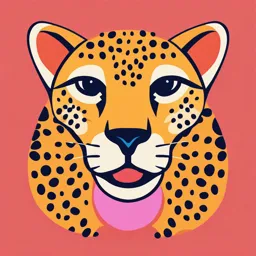
Uploaded by BonnyAntigorite9581
Tags
Related
- Pharmacology Basic Principles PDF
- Pharmacokinetics II: Drug Metabolism Lecture Notes (PDF)
- Pharmacology Introduction - PDF
- Introduction to Pharmacology Lecture 2: Pharmacokinetics PDF
- Concepts In Pharmacology Lectures 1 & 2 (University of Bradford) PDF
- PHAR435 Drug Metabolism Lecture Notes Fall 2024 PDF
Summary
This document provides an overview of pharmacokinetics, explaining how drugs move through the body. It details the four key processes: absorption, distribution, metabolism, and excretion (ADME). This information is crucial to understanding drug action and response.
Full Transcript
Pharmacokinetics Pharmacokinetics (PK), sometimes described as what the body does to a drug, is the study of how administered medications move throughout the body. There are four main things that the body does to a drug: it absorbs it into the bloodstream; distributes it to various areas of the body...
Pharmacokinetics Pharmacokinetics (PK), sometimes described as what the body does to a drug, is the study of how administered medications move throughout the body. There are four main things that the body does to a drug: it absorbs it into the bloodstream; distributes it to various areas of the body; metabolizes it into different compounds that are easier to eliminate; and excretes it from the system. Remember, there can be significant interpatient variability with all of these processes. Individual characteristics such as genetics, disease factors such as diarrhea, or non- modifiable factors such as age may affect these steps. A useful mnemonic that can help you remember this process is ADME: Absorption Distribution Metabolism Excretion The ADME process: 1. Site of Administration: There are several routes of drug administration. In this example, the drug is administered orally, which is the most common route of administration. 2. Absorption: When the drug enters the gastrointestinal tract, drug release and dissolution occurs, followed by absorption into systemic circulation. 3. Distribution: After absorption, the drug is distributed throughout the bloodstream and various tissues, which is necessary for the drug to have a pharmacological effect. 4. Metabolism: Although it depends on the drug itself and route of administration, the drug is most commonly metabolized in the CYP450 system, as well as through extrahepatic mechanisms. 5. Excretion: After the drug has been absorbed, distributed, and metabolized, it is excreted or eliminated from the body, usually renally and/or through the hepatic function. Study Guide: Pharmacokinetics – The Journey of Drugs in the Body Overview of Pharmacokinetics Pharmacokinetics is the study of how drugs move through and interact with the body. It involves four main processes: 1. Absorption: How drugs enter the body and move into the bloodstream. 2. Distribution: How drugs travel through the bloodstream to their target cells or molecules. 3. Metabolism: How drugs are chemically modified by the body, usually to render them inactive. 4. Excretion: How drugs and their metabolites are eliminated from the body. 1. Absorption Definition: Movement of a drug from its administration site into the bloodstream. Pathways of Absorption: o Topical Administration: Through the skin or mucous membranes into nearby blood vessels. o Oral Administration: Through the lining of the stomach or intestines. § Influenced by Food: Food slows the process of absorption. § Transport Mechanisms: § Passive Transport: Diffusion through cell membranes. § Active Transport: Requires energy to move drugs through membranes. Key Concept: Bioavailability – The proportion of a drug that successfully enters systemic circulation and is available for use. 2. Distribution Definition: The movement of drugs through the bloodstream to their target cells or molecules. Influencing Factors: o Plasma Proteins: Drugs may bind to proteins in the blood. § Tight binding limits the drug’s ability to reach target cells. § A second drug may be used to displace the first, improving its effectiveness. o Anatomical Barriers: § Blood-Brain Barrier: Blocks certain substances from entering brain tissue; allows psychotropic drugs to pass. § Blood-Placental Barrier: Regulates substances passing from a pregnant woman’s bloodstream to the fetus. § Blood-Testicular Barrier: Limits drug access to the testes. 3. Metabolism Definition: The chemical modification of drugs by enzymes, typically to inactivate them for excretion. Key Processes: o Drugs are often metabolized in the liver, especially after oral administration. § First-Pass Effect: Oral drugs are heavily metabolized during their first pass through the liver, reducing bioavailability. § In rare cases, metabolism in the liver may activate a drug. o Enzymes play a crucial role in breaking down drugs into forms that the body can excrete. Purpose: Protects the body by detoxifying small molecules, which are not managed by the immune system. 4. Excretion Definition: The removal of drugs and their metabolites from the body. Excretion Pathways: o Urination: Primary pathway via the kidneys. o Defecation: Through bile secreted by the liver and reabsorbed via enterohepatic recirculation. o Exhalation: For drugs metabolized into gaseous forms. o Sweating and Saliva: Minor pathways for drug excretion. Role of the Kidneys: Filter harmful substances and remove them from the bloodstream. Factors Affecting Pharmacokinetics Food and Drink: Can slow or alter absorption. Other Drugs: Can compete for binding to plasma proteins or interact with metabolic enzymes. Individual Variability: Genetics, age, liver and kidney function, and health conditions. Key Terms Absorption: Entry of drugs into the bloodstream. Bioavailability: The proportion of a drug available in circulation. Distribution: Movement through the bloodstream to target cells. Metabolism: Enzyme-driven modification of drugs. First-Pass Effect: Significant reduction of drug bioavailability due to liver metabolism. Excretion: Removal of drugs and their byproducts from the body. 2. Routes of Administration NURS 6020O 01 (SP25): Advanced Pharmacology Overview There are many routes of administration, with most drugs ultimately entering into systemic circulation. Different routes of administration may affect drug absorption and distribution differently and are therefore important to consider. 1. Oral Administration The most common route of administration is via the mouth, giving access to the gastrointestinal tract (GI). Here the drug metabolizes in the liver before reaching the systemic circulation. Drugs could also be administered sublingually, or under the tongue, entering the bloodstream directly via capillaries under the tongue and bypassing the liver. There are fundamental processes that describe oral drug absorption depending on the formulation of the drug (liquid or solid) including: 1. The disintegration of a solid tablet into smaller particles. 2. The dissolution of a drug into gastrointestinal (GI) fluid. 3. The permeation of a dissolved drug through the intestinal wall and into the bloodstream. There are several factors that influence how quickly and how much of the drug is absorbed into systemic circulation. Processes for Oral Drug Absorption: 1. Disintegration of a solid tablet into smaller particles. 2. Dissolution of a drug into gastrointestinal (GI) fluid. 3. Permeation of a dissolved drug through the intestinal wall into the bloodstream. Factors Influencing Absorption: Physical State of the Drug: Solutions (fully dissolved drugs) are absorbed faster than suspensions or solid forms. Surface Area: Smaller granules dissolve faster and have greater absorption compared to solid tablets. Blood Flow: Higher blood flow leads to better absorption (e.g., GI tract has high blood flow). Lipid Solubility: Lipophilic drugs penetrate cell membranes more effectively (e.g., ciprofloxacin). pH of the GI Tract: Weak acids absorb better in acidic environments (stomach), while weak bases are better absorbed in basic environments (intestines). Dosage Form: Controlled release or enteric-coated drugs can alter absorption location and speed. Gastric Emptying Time: Affected by factors like food, menstruation, posture, smoking, and medications. 2. Intravenous Administration Intravenous (IV) administration delivers concentrated medications directly into the vein using a syringe or tube. Advantages: Drawbacks to oral absorption are bypassed with intravenous (i.e. no first-pass effect) Potential higher peak concentrations Potential for immediate effects (shorter time to max concentration) Allows rapid dose titration Disadvantages: High initial concentration = risk of toxicity Invasive = risk of infection Must be administered by health care provider Diluent-related complications (e.g. voriconazole, phenytoin) Cannot take back the dose 3. Subcutaneous (SC) and Intramuscular (IM) Administration A subcutaneous (meaning ‘under the skin’) injection is a method of administering medication directly into the tissue layer between the skin and the muscle, usually with a hypodermic needle or syringe. Medication given this way is usually absorbed more slowly than if injected into a vein, sometimes over a period of 24 hours. It is a convenient way for a patient to administer medications to themselves. Subcutaneous injections are highly effective in administering medications such as insulin, morphine, and some vaccines. An intramuscular injection delivers medication into a muscle. This allows for faster absorption into the bloodstream without the tools needed to give IV. Examples of intramuscular injections are the flu vaccine and diazepam for seizures. Advantages: Both can have prompt absorption from aqueous solutions (water soluble) Easier administration than IV SC can be used for suspensions Can extend duration of release of drug IM can be used for drugs in oil-based vehicles Feasible for outpatient/self-administration Disadvantages: Pain with IM injections is greater than with SC injections Possible tissue damage Variability in absorption Based on lipid solubility of the drug, absorption can change Different sites of administration have different compositions and therefore different absorption Exercise changes the temperature of the tissue, changing absorption Avoid IM in patients with significant thrombocytopenia Avoid SC in patients with significant edema IM volume > 1 ml (non-buttocks) or > 2.5 ml (buttocks) should be divided into multiple injections 4. Topical Administration Topical administration refers to the application of medication to the surface of the skin or mucous membrane of the eye, ear, nose, mouth, vagina, etc. with the intent of containing the pharmacological effect of the drug only to the surface, or within the layers, of skin or mucous membrane. Drugs for topical application are usually available as creams, ointments, gels, lotions, sprays, powders, aerosols, liniments, and drops. Mucous Membranes: Mucous membranes include conjunctiva, nasopharynx, oropharynx, and the urinary bladder. The primary objective in administering to mucous membranes is to localize the effect of the drug and therefore minimize systemic exposure. This type of administration is also advantageous because of its rapid onset of action. However, there are times when significant systemic absorption does occur. If the mucous membrane is damaged from a burn or other causes that have led to abrasions, drugs will more likely be absorbed into the bloodstream. Some mucous membranes are used intentionally for their systemic absorption, for example, intranasal administration of fluticasone is used for systemic allergic rhinitis symptoms. Skin: Transdermal/topical drug administration involves drug diffusion through distinct layers of the skin into systemic circulation, bypassing the GI tract. Absorption is, therefore, dependent on several factors, including: Surface area over which drug is applied Blood flow (inflammation increases cutaneous blood flow, thus increasing absorption) Skin thickness (e.g. thin skin of face versus thick skin of palms) Lipid solubility (higher lipid solubility leads to greater absorption) Another important factor to note in transdermal drug administration is that the epidermis acts as a lipid barrier and drugs that are lipophilic will be easily absorbed, potentially into the bloodstream. Also, the dermis is freely permeable to many drugs and will allow for ready absorption. 5. Pulmonary Administration Pulmonary administration, or inhalation, is typically used for local administration for medications in which the site of action is the lungs. These medications are inhaled through the mouth with a breath and delivered directly to the lungs. An example of this is albuterol, which is used to treat an acute asthma attack. Some medications can be delivered through the lungs for a systemic effect specifically due to the properties of the medications, such as some anesthesia. Advantages: Rapid onset Can be used for local effect or to minimize systemic adverse effects Gaseous and volatile drugs, such as anesthesia, can be administered for systemic effects due to absorption via the large surface area of the respiratory tract Disadvantages: May require more coordination from patient (consider using nebulization or “spacer”) Potential variability in delivery Inhalers must be primed, may be difficult to tell when nearing empty 6. Alternative Routes of Administration Alternative routes of administration that bypass the GI tract include: ophthalmic (eye drops), intra-arterial (directly into the arteries), and intrathecal (directly into the spinal cord). Infiltration injection and rectal administration are additional alternate routes of administration. Ophthalmic: Eye drops for localized effects. Intra-Arterial: Injection directly into arteries. Intrathecal: Injection into the spinal cord. Infiltration Injection: Injection into tissue for localized effects. o Injection directly into tissue o Duration significantly increased when administered with vasoconstrictor (epinephrine) o Can be used to provide local anesthesia without significant systemic absorption Rectal Administration: Useful for patients unable to take oral medications; partially bypasses the liver and first-pass effect. This administration route is useful for patients who may be unable to take drugs by mouth, and will deliver the drug partially to the gastrointestinal tract or otherwise directly into systemic circulation. o 50% of drug absorbed will bypass liver/first-pass effect o Absorption may be irregular, incomplete, and can potentially cause irritation to rectal mucosa. Advantages of Rectal Administration: 50% of drug absorbed bypasses the liver. Disadvantages of Rectal Administration: Irregular and incomplete absorption. Potential irritation of rectal mucosa. 3. Absorption Absorption is the first factor that influences the movement of a drug throughout the body, from its site of administration to the circulatory system. Important effects and phenomena in relation to absorption include the bioavailability, the first-pass effect, enterohepatic recirculation, and the transporter p-glycoprotein. Bioavailability It is the fraction or percentage of the drug that reaches the systemic circulation and is denoted by the letter f (range 0-1) or F when the range is a percentage (0-100%). Put simply, the bioavailability of a drug is the rate and extent of its absorption into the systemic circulation. When a drug is administered intravenously, its bioavailability is 100%. However, when a medication is administered via other routes, its bioavailability is generally lower than that of intravenous due to intestinal endothelium absorption and the first-pass effect. While there are many factors that might influence the bioavailability of a drug, including multiple differences between individuals, interpatient variability in absorption is most important with drugs that are poorly bioavailable. When converting intravenous (IV) therapy to oral therapy (PO), bioavailability and duration of action must be taken into account. First-Pass Effect Drugs that are administered orally must first pass from the intestine to the liver before reaching the systemic circulation. This phenomenon — in which a drug gets metabolized in the liver before reaching the systemic circulation, resulting in a reduced concentration of the active drug — is called the first-pass effect. Drugs that are metabolized highly will be those that are most affected by the first-pass effect. The first-pass effect also decreases bioavailability. Another interesting effect to be aware of in drug absorption is enterohepatic recirculation, in which the circulation of biliary acids, bilirubin, drugs, or other substances from the liver are transported back to the liver. This effect has several important results: Recirculating the drug in the body Increases the extent of drug absorption Increases drug half-life Larger area under the curve (AUC) or total drug exposure P-Glycoprotein P-glycoprotein is one of the drug transporters that determine the uptake and efflux of a range of drugs. This process affects the drugs’ plasma and tissue concentrations and, ultimately, their final effects. P-glycoprotein functions as a transmembrane efflux pump, pumping its substrates from inside to outside the cell. The efflux pump is located in the esophagus, stomach, and small/large intestine that pumps drugs back into the GI tract from inside cells, thus decreasing absorption. Interacting drugs affect absorption when a p-glycoprotein inducer or inhibitor is given with a p-glycoprotein substrate. For example, rifampin induces p-glycoprotein, leading to more efflux pumps. When given with dabigatran (Pradaxa®), more dabigatran is pumped out of the cells and back into the lumen of the GI tract, leading to further decreased absorption. 4. Distribution Once a drug has been absorbed, it moves from the site of absorption to tissues around the body. This distribution from one part of the body to another is typically accomplished via the bloodstream, but it can also occur from cell to cell and other tissues such as brain tissue, fat, and muscle. The rate of delivery to tissues is called perfusion and is contingent on: Cardiac output Regional blood flow Capillary permeability Tissue volume The ability of a drug to pass from systemic circulation to tissue is called diffusion, or the mechanism of drug transport through a biological membrane. Diffusion is influenced by several factors, including: Lipid solubility of drug Transmembrane pH gradient of weak acids/bases (not a major factor) Size of the drug molecule The physiologic volumes of different pockets in the body (e.g. blood volume, lymph) will also influence where drugs are most likely to be distributed. The affinity of a drug to be bound to tissue or to circulating proteins will affect where the drug is likely to be primarily distributed. The process of drug distribution is important because it can affect how much drug ends up at the active sites, thus affecting both drug efficacy and toxicity. Examine the graphic below to view where distribution occurs. Protein Binding Some drugs are highly bound to certain proteins in the bloodstream. Changes in the availability of these proteins alter how much drug is available for distribution into the tissues. There are many multiple proteins found in the plasma such as albumin (most important), alpha-1-acid glycoprotein (AAG), and other lipoproteins and globulins. Drugs that are bound to these proteins are not free to bind to receptors of target tissues as the proteins generally cannot leave the bloodstream. The drug is released gradually from the plasma proteins. Only the amount of the drug that is unbound, or free, from the plasma proteins is available to bind to target tissues and receptors to induce a response. Not all drugs are highly bound to proteins, but in diseases where patients do not make normal amounts of albumin, such as liver disease and malnutrition, this leads to higher levels of drugs that do want to bind to these proteins. This can lead to adverse effects. Tissue Binding o Most drugs work by binding to specific target tissues. Adverse effects may happen when drugs bind in other tissues. Some drugs accumulate at higher levels in tissues than in the blood. High rates of tissue binding can result in prolonged release of the drug from the tissue. An example of this is marijuana. Some specific tissue considerations are: o Fat o Highly lipid soluble drugs can easily accumulate o Low blood flow o Most common area where drug can bind § Bone o Potential for absorption into bone-crystal surface Central Nervous System The central nervous system (CNS) has a unique distribution compared to other tissues. The tight junctions in the capillary endothelial and pericapillary glial cells of the CNS, which comprise the blood-brain barrier, are much harder to distribute across compared to other epithelial cells. Lipid solubility is a major determinant in ability to cross blood- brain barrier, as the higher the lipid solubility, the greater the likelihood of the drug crossing the blood-brain barrier. It is important to note that both meningeal and encephalic inflammation can increase local permeability. So some drugs may cross the blood-brain barrier with ease when patients are experiencing this inflammation, which can lead to adverse effects. Volume of Distribution The distribution of a drug is often measured as a volume of distribution (Vd) and is a measure of the fluid volume that would be required to contain the amount of a drug present in the body at the same concentration as that measured in the plasma. Often this is normalized to body weight (kg) in which case the units are L/kg. Large volumes of distribution (greater than 42L) distribute extensively into tissues, especially fat. Small volumes of distribution (< 5L) remain primarily in the bloodstream. It is important to note that: The measured volumes are not actual physical volumes but mathematical values, which relate the amount of drug in the body to the concentration of drug in the plasma. Drugs that have weight-based dosing may require dosing based on ideal body weight or adjusted body weight if the Vd for the drug is small. The calculation for the volume of distribution (Vd) is as follows: The Vd of a drug can help us determine if we need to give a loading dose to get the levels of a drug up quickly. We can use Vd to calculate a loading dose. The calculation for the loading dose is as follows: Loading dose = (Cp x Vd) / (F x S) Cp = Desired peak plasma concentration Vd = Volume of distribution F = Bioavailability S = Salt factor Distribution Models Distribution models are important for us to consider to help predict how much of a dose that is administered to a patient will reach the target tissues and thus be excreted. - One-Compartment Model: is an oversimplified model for drug distribution. It suggests that all the drug that is administered reaches the body and is then eliminated. - Two-Compartment Model: is the simplest model of distribution that is the most accurate. The drug is administered and reaches the blood, which then distributes back and forth between the blood. The drug can then be eliminated while it is in the blood compartment. Most drugs are not going to have equal concentrations in both the tissues and the blood. Most drugs, based on their properties, are going to favor being either in the blood compartment or the tissue compartment. Drug properties that prefer blood compartment: Hydrophilic drugs Usually have small Vd Drug properties that prefer tissue compartment: Lipophilic drugs Usually have large Vd Most drugs are not going to have equal concentrations in both the tissues and the blood. Most drugs, based on their properties, are going to favor being either in the blood compartment or the tissue compartment. Drug properties that prefer blood compartment: Hydrophilic drugs Usually have small Vd Drug properties that prefer tissue compartment: Lipophilic drugs Usually have large Vd 5. Metabolism Drug metabolism is the biotransformation of a drug by organs or tissues (primarily the liver, kidney, skin, or digestive tract) so that the drug can be excreted. To facilitate removal via feces or urine, the drug compound is altered to become more water- soluble. The cytochrome P (CYP)-450 enzymes are responsible for the metabolism of most medications, with the most clinically important being: CYP1A2 CYP2C9 CYP2C19 CYP2D6 CYP3A4 CYP3A5 Drugs can go through phase I metabolism, phase II metabolism, or both before being excreted from the body. Some drugs may even be excreted completely unchanged. Phase I Metabolism Phase I metabolism consists of reduction, oxidation, or hydrolytic reactions. These reactions serve to convert lipophilic drugs into more polar (water soluble) molecules and generally lead to the introduction of functional groups and the biological inactivation of the drug. These reactions may create active metabolites that have some activity of the original drug and may have adverse effects. Note that phase I metabolism does decrease with age. Phase I includes the cytochrome P450 system. This is the largest group of enzymes that are used in metabolism and is the cause of many drug-drug interactions. For example, if another drug inhibits a CYP450 enzyme, the levels of other drugs metabolized by that enzyme will increase. Alternatively, if a drug induces the CYP450 enzyme, the levels of other drugs metabolized by that enzyme will decrease. Let’s examine some examples of phase I metabolism below: Oxidation reactions o Cytochrome P450 reactions (CYP450) o Most common cause of drug interactions Reduction reactions o Azo reductions, nitro reductions, carbonyl reductions Hydrolysis reactions o Esters (e.g. procaine) o Amides (e.g. lidocaine) Phase II Metabolism Phase II reactions involve conjugation by coupling the drug or its metabolites (from phase I reactions) to another molecule. In addition to producing metabolites that improve water solubility, they increase molecular weight rather than biologically inactivating the drug. The ultimate goal of phase II reactions is to form water-soluble products that can be excreted by the body. Phase II metabolism is generally preserved in elderly patients. There are several types of phase II reactions that occur, including: Glucuronidation Acetylation Glutathione conjugation Glycine conjugation Sulfation Methylation 6. Excretion Excretion is the process by which the metabolized drug compound is eliminated from the body, most commonly as feces or urine. Important considerations for drug excretion include how rapidly the drug is eliminated and what pathway it takes to exit the body. Molecular size and charge influence the excretion pathway. When the drug itself or metabolic by-products bioaccumulate because they are not excreted appropriately, adverse effects can occur. This can impact kidney function, liver function, or even overwhelm the metabolic system before the point of excretion. Liver metabolism and kidney elimination most commonly determines when the action of a drug is terminated. Drugs that are highly lipid soluble are also difficult to excrete. They often have to be metabolized multiple times until the drugs are water soluble enough to be excreted by the kidneys. Elimination or excretion is the final step to terminating the pharmacokinetics of a drug. Elimination Models It is often important to predict how quickly a drug will be eliminated from the body. This helps to determine when drugs should be redosed or when their action is no longer relevant. Select the headings below for details about each model. (tabs or accordions here) Half-Life The half-life of a drug (t1⁄2) is the time it takes for the amount of drug in the body to be reduced by half. This is dependent on several factors, including: Dosing interval Drug duration Time to steady-state In most cases — drugs that behave with first-order elimination — 97% of the drug is eliminated after five half-lives. It is important to take note of the patient’s age, kidney, and/or liver function when considering the half-life of a drug. The half-life value is estimated using the following equation, where k is a constant dependent on the drug itself: t1/2 = 0.693/k Therapeutic Drug Monitoring Therapeutic drug monitoring (TDM) is the clinical practice of measuring specific drugs at designated intervals to maintain a constant concentration in a patient’s bloodstream. TDM is important in optimizing individual dosage regimens and minimizing toxicity. It is unnecessary to employ TDM for the majority of medications. It is used mainly for monitoring drugs with narrow therapeutic ranges, drugs with marked pharmacokinetic variability, medications for which target concentrations are difficult to monitor, and drugs known to cause therapeutic and adverse effects. Study Guide: The Journey of Aspirin Through the Body 1. Absorption Pathway: o After swallowing, the aspirin tablet travels down the esophagus into the stomach. o The tablet dissolves into particles, which pass into the small intestine. o Small Intestine: § The primary site of absorption. § Lined with villi (tiny, finger-like projections) that increase the surface area for absorption. § Villi are rich in blood supply and lined with thin epithelial cells. o Aspirin molecules pass through the epithelial cells into the bloodstream. Key Points: o The small intestine is the most efficient site of aspirin absorption due to its large surface area. o Epithelial cells and villi play critical roles in allowing aspirin molecules to enter the blood. 2. Distribution Pathway: o Aspirin molecules enter the bloodstream. o In the blood, albumin (a liver-produced protein) binds to the aspirin molecules. o Bound vs. Free Molecules: § Bound molecules are temporarily inactive as they travel in the bloodstream. § Only unbound aspirin molecules exert an effect on the body. o The liver filters a portion of the aspirin through the first-pass effect, reducing the active dose. Key Points: o Albumin acts as a transport vehicle for aspirin and other nutrients. o The amount of aspirin available to act on the body depends on its binding affinity to albumin and the first-pass metabolism in the liver. 3. Metabolism Pathway in the Liver: o Aspirin is metabolized in two phases: 1. Phase One: § Aspirin undergoes hydrolysis, breaking into ethanoic acid and salicylic acid. § Salicylic acid is not water-soluble. 2. Phase Two: § Salicylic acid is conjugated with an ionized group (e.g., glucuronide). § This forms a water-soluble compound that can be excreted in urine. Oral Bioavailability: o Only about 68% of orally taken aspirin has an effect due to extensive filtering by the liver and other processes. o Non-oral administration (e.g., intravenous) bypasses the liver's first-pass effect, resulting in 100% bioavailability. Key Points: o The liver plays a significant role in modifying aspirin for safe excretion. o The first-pass metabolism is a crucial step in reducing the drug's active presence in the body. 4. Excretion Pathway: o Remaining aspirin and its metabolites circulate through the blood supply multiple times. o Eventually, they are transported to the kidneys for filtration. o The kidneys excrete the water-soluble metabolites (e.g., glucuronide) in the urine. Key Points: o The kidneys are the primary site for aspirin excretion. o Continuous filtration ensures aspirin is cleared from the body. Mechanism of Action at the Site of Pain Prostaglandin Inhibition: o Aspirin blocks the production of prostaglandins, which cause inflammation and pain. o By stopping prostaglandin synthesis, aspirin reduces swelling and inhibits pain signals sent to nerves. Key Points: o Aspirin's effect at the pain site depends on its availability in the blood. o The drug prevents inflammation and reduces pain perception. Quick Summary Table Process Description Aspirin dissolves in the stomach, absorbed in the small intestine into the Absorption blood. Aspirin binds to albumin; only unbound molecules act on the body. Liver Distribution filtering occurs. In the liver, aspirin is converted to water-soluble compounds for Metabolism excretion. Excretion Metabolized aspirin is filtered by the kidneys and excreted in urine. Tips for Understanding Focus on how aspirin's journey changes its chemical form at each step. Remember the role of key organs: small intestine (absorption), liver (metabolism), and kidneys (excretion). Relate the bioavailability concept to how different drug administration methods impact efficacy. 1. Pharmacodynamics Pharmacodynamics (PD) is sometimes described as what a drug does to the body. It is the study of the biochemical, physiologic, and molecular effects of drugs on the body and involves: Receptor binding (including receptor sensitivity) Postreceptor effects Chemical interactions Pharmacodynamics, together with pharmacokinetics, helps explain the relationship between dose and response — that is, the drug’s effects. The pharmacologic response depends on the drug binding to its target. The concentration of the drug at the receptor site influences the drug's effect. Pharmacodynamics ultimately drives both the efficacy and toxicity of drugs. A drug’s pharmacodynamics can be affected by factors of interpatient variability including: A disorder or disease Receptor density and sensitivity Genetic factors Drug interactions Tolerance (i.e.diminished effectiveness with continuous use) Study Guide: Key Pharmacological Concepts 1. Drug-Receptor Interactions Mechanism: o Drugs interact with specific cellular receptors, typically proteins, to elicit physiological responses. o Agonists: Bind to receptors and activate them, mimicking the action of endogenous substances. o Antagonists: Bind to receptors without activating them, blocking the action of endogenous substances or agonists. Example: o Beta-blockers are antagonists that bind to beta-adrenergic receptors, preventing activation by endogenous catecholamines like adrenaline, thereby reducing heart rate and blood pressure. 2. Potency Definition: o Potency refers to the amount of a drug needed to produce a given effect. A more potent drug elicits the desired response at a lower dose compared to a less potent drug. Measurement: o Often assessed by the drug's EC50 (the concentration required to achieve 50% of the maximal effect). A lower EC50 indicates higher potency. Example: o Fentanyl is more potent than morphine; it requires a much smaller dose to achieve similar analgesic effects. 3. Efficacy Definition: o Efficacy refers to the maximum effect a drug can produce, regardless of dose. It indicates the drug's ability to activate receptors and elicit a full response. Distinction from Potency: o A drug can have high potency (effective at low doses) but low efficacy (unable to produce a maximal response), and vice versa. Example: o Partial agonists, like buprenorphine, have lower efficacy compared to full agonists like morphine, as they cannot produce the same maximal effect even at full receptor occupancy. 4. Binding Affinity Definition: o Binding affinity describes the strength of the interaction between a drug and its receptor. High affinity means the drug binds tightly and remains associated with the receptor longer, often leading to prolonged effects. Measurement: o Quantified by the dissociation constant (KD); a lower KD indicates higher affinity. Example: o Naloxone has a high affinity for opioid receptors, allowing it to displace opioid agonists like morphine or heroin, effectively reversing opioid overdose effects. Key Relationships Potency and Efficacy: While potency indicates the dose required for effect, efficacy denotes the maximum effect achievable. A highly potent drug isn't necessarily the most efficacious. Affinity and Efficacy: High binding affinity doesn't always correlate with high efficacy. A drug may bind tightly to a receptor (high affinity) but not activate it fully (low efficacy), as seen with antagonists. 2. Receptor Binding Receptors are protein molecules that are generally present on the cell surface or within the cell in the human body. They receive signals (chemical information) from outside the cell. This information comes from other molecules such as hormones, neurotransmitters, and drugs. Activated receptors directly or indirectly regulate cellular biochemical processes. Drugs and other molecules inside your body that bind to a receptor are called ligands. The effects of most drugs result from interaction with various types of receptors. The binding can be specific and reversible. A ligand may activate or inhibit a receptor and thus can increase or decrease a particular reaction or cell function. Most ligands can interact with multiple receptor subtypes. Few, if any, drugs are absolutely specific for one receptor or subtype, but most have relative selectivity. Selectivity is the degree to which a drug acts on a given site relative to other sites. The characteristics of receptor binding can be summarized as: Specificity Affinity Intrinsic activity Potency Receptor Specificity Ligands, including drugs, want to bind to specific receptors. Not all ligands can bind to all receptors; otherwise, drugs wouldn’t work! In the graphic below we see a synaptic cleft between two neurons. The pre-neuron is releasing ligands and the post-neuron has the receptors that will interact with the ligands. Here we can see that the ligands represented by blue triangles bind well into the receptor. The ligands represented by red circles would not bind to this receptor. Receptor Affinity Receptor affinity is the extent to which the ligand is capable of binding and remains bound to a receptor. When there is high receptor affinity, the ligand binds well and remains bound long enough to interact with the receptor. When there is low receptor affinity, the ligand does not bind well and may not remain bound long enough to interact with the receptor. Intrinsic Activity Intrinsic activity is the extent to which the ligand activates the receptor. When there is high intrinsic activity, as demonstrated in the graphic below, the ligand produces a large effect on the postsynaptic cell. This is considered a full agonist, or a ligand that elicits a full physiologic response when it interacts with the receptor. In the image below, the dark blue arrows represent the full physiologic response. When there is low intrinsic activity, as demonstrated in the graphic below, the ligand produces a small or inconsistent effect through its interaction with the receptor. It is important to note that this is different from low affinity in that these drugs still bind tightly to the receptor. This is a partial agonist, or a ligand that elicits only a partial physiologic response when it interacts with the receptor. In the image below, the single dark blue arrow represents only a partial response. Agonist and Antagonist Reactions An agonist is a drug that binds to a receptor, producing a biological response in the receptor. Agonists usually have high affinity and high intrinsic activity. An antagonist is a drug that binds to a receptor and stops the receptor from producing a response. It blocks these receptors and renders them ineffective. This can be achieved through a blockade of binding of endogenous compounds (e.g. enzyme inhibition), or through conformational change of the receptor to render it inactive or block further biochemical steps (e.g. antibiotics). Antagonists usually have high affinity and low intrinsic activity. In other words, the agonists turn the receptors on and the antagonists turn them off. There are two types of agonist and antagonist drugs: reversible/irreversible and competitive/noncompetitive. Reversible/Irreversible Competitive/Non-competitive If a drug is reversible, it can be unbound Competitive drugs compete with other from the receptor. A reversible drug is ligands for binding to the same receptor. almost always competitive and has weak Non-competitive drugs exert their effect chemical bonds. For example, hydrogen without competition for occupancy of the bonds or Van der Waals. same receptor. Irreversible drugs cannot be unbound from the receptor. They are almost always non-competitive and often have covalent/strong bonds. 3. Efficacy and Potency Efficacy is the term used to describe the extent to which a drug can produce a response when all available receptors or binding sites are occupied (i.e. Emax on the dose-response curve). When comparing drugs acting at the same receptor, a full agonist will have the greatest efficacy and can produce the maximum response of which the receptor is capable. Potency is a term used to describe the amount of a drug required for a given response. The potency of a drug is related to its affinity for the receptor (i.e. how readily the drug-receptor complex is formed). Less potent drugs can have an efficacy similar to that of a more potent one; the difference in potency can be readily overcome by giving the less potent drug in higher doses. This is illustrated by the varying recommended dose ranges of drugs acting at the same drug target (e.g. H2 antagonists, ACE inhibitors). In summary: Potency is the concentration (EC50) or dose (ED50) of a drug required to produce 50% of that drug’s maximal effect. Efficacy (Emax) is the maximum effect that can be expected from this drug (i.e. when this magnitude of effect is reached, increasing the dose will not produce a greater magnitude of effect). The image below shows a dose-response curve. These drugs have equal efficacy as they both reach 100% response. However, the blue drug has a higher potency so you need a lower dose to get the same response. 4. Drug Interactions There are many interactions that can affect the response to a drug. Drugs may interact with any of the following factors, which will lead to a change in drug levels and thus response: Drug(s) Food Supplements Disease Patient Factors There are multiple individual patient factors that can influence drug interactions and can explain why we may see a drug interaction in one patient, but not another. Clearance Genetics Hormones Comorbid conditions Diet Drug-Specific Factors It’s important to note that you may be concerned about a drug interaction only in certain situations, and drug interactions may be avoided by changing some of these factors: Formulation Route Sequence of drug administration Diet Metabolism Drug interactions due to metabolism tend to be the interaction we think about the most. Since enzymes are used in phase I and phase II reactions, patients can have genetic polymorphisms which make them slow-, rapid-, or even ultra rapid- metabolizers. These enzymes, especially CYP450, can also be inhibited or induced by other drugs. Inhibition can occur very quickly with a rapid onset and offset, where induction takes time to have an effect due to the need to make more enzymes. We use the following terms to define how drugs interact with enzymes: Substrate: metabolized by enzyme Inducer: increased expression of enzyme Inhibitor: decreased activity/expression of enzyme Pharmacodynamics Drug interactions can also be related to pharmacodynamics. Imagine that you might have two drugs that do something similar, like lower heart rate. This would be synergistic. You may also have two drugs that work against each other, like a drug that increases blood pressure and one that decreases blood pressure. This would be antagonistic. Synergistic effects (1+1= >2) Bind at different receptors (i.e. allosteric sites) but have additive effects Physiologic antagonism (1+1=