Chemistry Syllabus - Aromatic Hydrocarbons PDF
Document Details
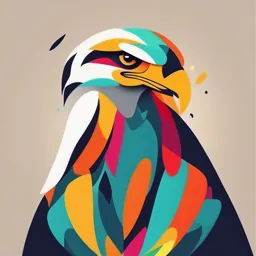
Uploaded by FastPacedConsciousness9847
Mount Carmel College Autonomous Bangalore
Tags
Summary
This document is a chemistry syllabus focusing on aromatic hydrocarbons. It covers topics such as nomenclature, structure, and properties of benzene, along with various reactions and substituents. The document includes information relating to aromatic, antiaromatic and non aromatic compounds.
Full Transcript
1 II Semester BSc (SEP Scheme) (2024 batch) Chemistry Syllabus Module 4: Aromatic Hydrocarbons 10 hours Nomenclature. Structure of benzene - using molecular orbital theory. Criteria for aromaticity- Huckel’s rule (Example...
1 II Semester BSc (SEP Scheme) (2024 batch) Chemistry Syllabus Module 4: Aromatic Hydrocarbons 10 hours Nomenclature. Structure of benzene - using molecular orbital theory. Criteria for aromaticity- Huckel’s rule (Examples: cyclopentadienyl anion, cycloheptatrieneyl cation, benzene, naphthalene, anthracene and phenanthrene). Antiaromaticity. General mechanism of aromatic electrophilic substitution. Mechanism of nitration of benzene including evidence for the formation of nitronium ion, energy profile diagram. Orienting influence of substituents in toluene, chlorobenzene, nitrobenzene and phenol. Aromatic nucleophilic substitution via benzyne intermediate, mechanism with evidences for the formation of benzyne by trapping with anthracene, Birch reduction, Side chain oxidation of toluene to benzaldehyde and benzoic acid, Oxidation of naphthalene, anthracene and phenanthrene. Diels-Alder reaction of anthracene with 1,2- dichloroethene. Alkenyl benzenes: Styrene, cis- and trans-stilbenes and their preparations. Biphenyl: Preparation-Ullmann reaction. ----------------------------------------------------------------------------------------------------- Introduction Benzene is a hydrocarbon with the chemical formula C6H6. It has 6 carbon atoms joined in a ring and has 1 hydrogen atom attached to each of the carbon atoms. By replacing one or more of the hydrogen atoms with some functional group, we get several benzene compounds. While naming the substituted benzene compounds, we prefix the name of the substituent to the word benzene. Monosubstituted benzene compounds For the benzene compounds consisting of a single substituent, we simply prefix the name of the substituent to benzene. Some examples along with their common names are listed below. Methylbenzene Hydroxybenzene Aminobenzene Ethylbenzene or Toluene or Phenol or Aniline Disubstituted benzene compounds When there are two substituents present in the compound, we number each of the carbon atoms in such a manner that the substituents are attached to the lowest possible numbered carbon atom. 1,3-dinitrobenzene is the name of the following compound. Naming it as 1,5-dinitrobenzene is incorrect because the carbon atom would not be numbered lowest. 1,3-dinitrobenzene 1,3-dichlorobenzene Moreover, when there is more than one substituent, we also name their positions as ortho- (o), meta- (m) and para- (p). They refer to the positions 1,2-; 1,3- and 1,4- respectively. Thus, we can name the compound 1,3-dichlorobenzene as m-dichlorobenzene. 1 2 Instead of using numbers to indicate substituents on a benzene ring, ortho- (o-), meta- (m-), or para (p-) can be used in place of positional markers when there are two substituents on the benzene ring (disubstituted benzenes). They are defined as the following: ortho- (o-): 1,2- (next to each other in a benzene ring) meta- (m): 1,3- (separated by one carbon in a benzene ring) para- (p): 1,4- (across from each other in a benzene ring) Poly-substituted Benzene Compounds In case of poly-substituted compounds, if there is a base compound present, we assign it as the position 1. We then choose the next compound for numbering such that it gets the lowest number. However, if there are no special or base group present compound (A), we list them in the alphabetical order, giving them the lowest numbers. For compound (B), aniline is the base compound. So, we name it as 3-chloro-2-nitroaniline. (A) (B) 1-Bromo-2,4-dinitrobenzene 3-chloro-2-nitroaniline Some examples of monosubstituted benzene compounds Benzonitrile Benzaldehyde Benzoic acid Toluene Phenol Aniline Acetophenone Benzene Anisole Mesitylene o-Xylene Styrene sulphonic acid Aromaticity Aromaticity is a property of conjugated cycloalkenes in which the stabilization of the molecule is enhanced due to the ability of the electrons in the π orbitals to delocalize. This act as a framework to create a planar molecule. 2 3 A molecule must fulfill four crucial requirements in order to be classified as aromatic. If any of these conditions are violated, no aromaticity is possible. (i) First, the molecule must be cyclic. cyclic acyclic cyclic acyclic Benzene 1,3,5-hexatriene pyrrole Aromatic not aromatic Aromatic not aromatic (ii) Second, every atom in the ring must be conjugated. “Every atom in the ring must have an available p orbital”, or “Every atom in the ring must be able to participate in resonance”. (iii) Third, the molecule must have [4n+2] pi electrons The third condition is that the cyclic, conjugated molecule must have the correct number of pi electrons. Benzene and cyclooctatetraene are both cyclic and conjugated, but benzene is aromatic and cyclooctatetraene is not. Other Examples: 3 4 for n = 0 , we have [4 (0) + 2] = 2 for n = 1 , we have [4 (1) + 2 ] = 6 for n = 2, we have [4 (2) + 2 ] = 10 for n = 3, we have [4 (3) +2 ] = 14 The numbers in this “magic series” are sometimes referred to as “Hückel Numbers” after Erich Hückel, who proposed this rule back in 1931. The condition that aromatic molecules must have [4n+2] pi electrons is sometimes called “Hückel’s rule”. (iv) Fourth, the molecule must be flat Generally if the first three conditions are met, then it is safe to assume that the molecule is flat. (but there are some exceptions). A prominent exception is the isomer of annulene as shown below Annulene is a cyclic, conjugated molecule and has 10 pi electrons. It is not flat due to repulsions between the hydrogens. However replacing the hydrogens with bonds to a bridging carbon allows all C-C pi bonds to be in the same plane. Thus making it aromatic. In 1931, German chemist and physicist Erich Hückel proposed a theory to help determine if a planar ring molecule would have aromatic properties. His rule states that if a cyclic, planar molecule has 4n+2 π electrons, it is considered aromatic. This rule is known as Hückel's Rule. Using the criteria for aromaticity, determine if the following molecules are aromatic: 1. Aromatic - only 1 of S's lone pairs counts as π electrons, so there are 6 π electrons, n=1 2. Not aromatic - not fully conjugated, top C is sp3 hybridized 3. Not aromatic - top C is sp2 hybridized, but there are 4 π electrons, n=1/2 4. Aromatic - N is using its 1 p orbital for the electrons in the double bond, so its lone pair of electrons are not π electrons, there are 6 π electrons, n=1 5. Aromatic - there are 6 π electrons, n=1 6. Not aromatic - all atoms are sp2 hybridized, but only 1 of S's lone pairs counts as π electrons, so there 8 π electrons, n=1.5 7. Not aromatic - there are 4 π electrons, n=1/2 4 5 8. Aromatic - only 1 of N's lone pairs counts as π electrons, so there are 6 π electrons, n=1 9. Not aromatic - not fully conjugated, top C is sp3 hybridized 10. Aromatic - O is using its 1 p orbital for the elections in the double bond, so its lone pair of electrons are not π electrons, there are 6 π electrons, n=1 Antiaromaticity is characteristic of a cyclic molecule with a π electron system that has higher energy due to the presence of 4n delocalised (π or lone pair) electrons in it. So a non-aromatic compound is neither cyclic nor planar. And an antiaromatic compound is cyclic, planar, and has the conjugation of p orbitals, but the difference is the number of electrons in the conjugated p orbitals. Examples of antiaromatic compounds Cyclobutadiene, pentalene, and cyclooctatetraene are examples of antiaromatic molecules. Cyclopropenyl anion is also antiaromatic because the carbanion can resonate with the double bond, which means the molecule is fully conjugated. Cyclopropenyl cation, cyclopropenyl anion Cyclopropene satisfies 4n+2 rule but it is not planar, it has 2 sp2 hybridised C and 1 sp3 hybridised C and no conjugation can occur and so this is not aromatic. cyclopropene cyclopropenyl cyclopropenyl cycloheptatrienyl anion cation cation 5 6 Cyclopropenyl anion has 4n pi electrons and planar so it is anti aromatic But cyclopropenyl cation is planar, having 2 pi electrons and conjugation so it is aromatic. cyclopropene cation The cycloheptatrienyl (tropylium) cation is aromatic because it also has 6 electrons in its pi system. It is planar and has conjugated system. Benzene It has 6π electrons: n = 1, 4n + 2 = 6: satisfies Huckle rule - Aromatic Cyclopentadienyl anion The cyclopentadienyl anion is a planar, cyclic, regular-pentagonal ion; it has 6 π-electrons (4n + 2, where n = 1), which fulfills Hückel's rule of aromaticity. Cyclopentadienyl cation Cyclopentadiene Cyclopentadienyl cation If a hydride anion (H−) is removed from the sp3 hybridized ring carbon, the cyclopentadienyl cation is formed, and the hybridization of the sp3 hybridized ring carbon is altered to sp2. Consequently, the cyclic cyclopentadienyl cation is planar and it possesses a cyclic uninterrupted π electron cloud. However, it does not meet Hückel's rule, as it has 4 π electrons. Therefore, it is antiaromatic. Abstraction of a hydrogen radical from the sp3 hybridized ring carbon yields the cyclopentadienyl radical. The hybridization of the sp3 hybridized ring carbon is transformed to sp2, as well. Analogous to the cyclopentadienyl cation, the cyclopentadienyl radical does not meet Hückel's rule, as it possesses five π electrons. Thus, it is not aromatic. The abstraction of a proton (H+) from the sp3 hybridized ring carbon yields the cyclopentadienyl anion. The hybridization of the sp3 hybridized ring carbon is consequently altered to sp2, as well. The cyclic cyclopentadienyl anion is planar, it possesses a cyclic uninterrupted π electron cloud, and it meets Hückel's rule, as it has 4x1 + 2 (n = 1) π electrons. Therefore, the cyclopentadienyl anion is a relatively stable aromatic species. The cyclopentadienyl cation and radical are considerably unstable and reactive species. 6 7 Structure of benzene 1. Molecular formula: Elemental Analysis and molecular weight determination have proved that the molecular formula of benzene is C6H6. This indicates that benzene is a highly unsaturated compound. 2. Straight chain structure not possible: Benzene could be constructed as a straight chain or ring compound but it is not feasible to have a straight chain structure since it does not show the properties of alkenes or alkynes. For example, it did not decolourise bromine in carbon tetrachloride or acidified KMnO4. It did not react with water in the presence of acid. 3. Evidence of cyclic structure: i) substitution reaction of benzene: Benzene reacts with bromine in the presence of AlCl3 to form mono bromobenzene. Formation of only one monobromo compound indicates that all the six hydrogen atoms in benzene were identical. This is possible only if it has a cyclic structure of six carbons each containing one hydrogen. ii) addition of hydrogen: Benzene can add on to three moles of hydrogen in the presence of nickel catalyst to give cyclohexane. cyclohexane This confirms cyclic structure of benzene and the presence of three carbon – carbon double bond. 4. Kekule’s structure of benzene: In 1865, August Kekule suggested that benzene consists of a cyclic planar structure of six carbon with alternate single and double bonds. There were two objections: i) Benzene forms only one ortho disubstituted products whereas the Kekule’s structure predicts two o-disubstituted products as shown below. In one of the structures there is a double bond between the carbons carrying the substituents whereas in the other there is a single bond. 7 8 ii) Kekule’s structure failed to explain why benzene with three double bonds did not give addition reactions like other alkenes. To overcome this objections, Kekule suggested that benzene was mixture of two forms (1 and 2) which are in rapid equilibrium. 5. Resonance description of benzene: The phenomenon in which two or more structures can be written for a substance which has identical position of atoms is called resonance. The actual structure of the molecule is said to be resonance hybrid of various possible alternative structures. In benzene, Kekule’s structures I & II represent the resonance structures, and structure III is the resonance hybrid of structure I & II I II III The structures I and II exist only in theory. The actual structure of benzene is the hybrid of two hypothetical resonance structures. 6. Spectroscopic measurements: Spectroscopic measurements show that benzene is planar and all of its carbon-carbon bonds are of equal length 1.40A°. This value lies between carbon-carbon single bond length 1.54A° and carbon- carbon double bond length 1.34A°. 7. Molecular orbital structure: Building the orbital model Benzene is built from hydrogen atoms (1s1) and carbon atoms (1s22s22px12py1). Each carbon atom has to join to three other atoms (one hydrogen and two carbons) and doesn't have enough unpaired electrons to form the required number of bonds, so it needs to promote one of the 2s2 pair into the empty 2pz orbital. Promotion of an electron There is only a small energy gap between the 2s and 2p orbitals, and an electron is promoted from the 2s to the empty 2p to give 4 unpaired electrons. The carbon atom is now said to be in an excited state. Hybridisation Because each carbon is only joining to three other atoms, when the carbon atoms hybridise their outer orbitals before forming bonds, they only need to hybridise three of the orbitals rather than all four. They use the 2s electron and two of the 2p electrons, but leave the other 2p electron unchanged. 8 9 The new orbitals formed are called sp2 hybrids, because they are made by an s orbital and two p orbitals reorganizing themselves. The three sp2 hybrid orbitals arrange themselves as far apart as possible which is at 120° to each other in a plane. The remaining p orbital is at right angles to them. Each carbon atom uses the sp2 hybrids to form sigma bonds with two other carbons and one hydrogen atom. The structure of benzene is best described in terms of the molecular orbital theory. All the six carbon atoms of benzene are sp2 hybridized. One sp2 hybrid orbital of each carbon linearly overlap with 1s orbital hydrogen atom to form 6 C – H sigma bonds. Overlap between the remaining sp2 hybrid orbitals of carbon forms six C – C sigma bonds. Formation of sigma bond in benzene All the σ bonds in benzene lie in one plane with bond angle 120°. Each carbon atom in benzene possess an unhybridized p-orbital containing one electron. The lateral overlap of their p-orbital produces 3 π- bond. The six electrons of the p-orbitals cover all the six carbon atoms and are said to be delocalised. Due to delocalization, strong π-bond is formed which makes the molecule stable. Hence unlike alkenes and alkynes benzene undergoes substitution reactions rather addition reactions under normal conditions. Delocalised π molecular orbital 9 10 Actually these 2pz orbital produce a π (pi) molecular orbital containing six electrons. One half of this π (pi) molecular orbital lies above the plane of hexagonal ring and remaining half below the ring like a sandwich. The overlap of these 2pz orbital results in the formation of a fully delocalized π (pi) bond, which extends all over the six C atoms of benzene nucleus. The molecular orbital approach clearly indicates that these six electrons could be found anywhere in a highly delocalized manner. As a result of delocalization, a stronger π (pi) bond and a more stable benzene molecule is obtained which undergo substitution reactions more frequently than addition reactions. Molecular orbital theory predicts that overlapping six atomic p orbitals will lead to the generation of six π molecular orbitals. Three of these π molecular orbitals will be bonding orbitals, while the other three will be antibonding orbitals, as shown below. The three low‐energy orbitals, denoted π1, π2, and π3, are bonding combinations, and the three high‐ energy orbitals, denoted π4*, π5*, and π6*, are antibonding orbitals. Two of the bonding orbitals (π2 and π3) have the same energy, as do the antibonding orbitals π4 and π5. Such orbitals are said to be degenerate. Because the electrons are all located in bonding orbitals, the molecule is very stable. Additional stability occurs because all the bonding orbitals are filled and all the π electrons have paired spins. Molecules that possess all these characteristics are said to have a closed bond shell of delocalized π electrons. Molecules such as benzene that possess a closed bond shell of delocalized π electrons are extremely stable and show great resonance energies. 8. Representation of benzene: Hence, there are three ways in which benzene can be represented. Expanded form Kekule structure Short hand representation Resonance stabilization energy: The difference between the energy of resonance hybrid and that of most stable resonance structure of a molecule is known as the resonance stabilization energy of that molecule. The following resonance structures can be written for benzene which are hypothetically possible due to delocalization of π electrons. The Kekule structures have more weightage than Dewar structures. 10 11 The actual structure of benzene is thus shown to be the hybrid of these contributing structures. One way to estimate the resonance energy of benzene is to compare the heats of hydrogenation of cyclohexene and benzene (benzene can be made to undergo hydrogenation under extreme conditions). The heat released in a hydrogenation reaction of one mole of an unsaturated (double bonded) compound is called the heat of hydrogenation. In the presence of a transition metal catalyst, hydrogen readily reduces cyclohexene to cyclohexane. Heat of hydrogenation of cyclohexene (one double bond) is 120 kJ/mol. Benzene does not react readily with reagents that add to alkenes, hydrogenation of benzene must be performed at extremely high pressures. If we imagine benzene in which the 2p electrons do not overlap outside of their original C - C double bonds, a hypothetical compound with alternating single and double bonds, we might expect its heat of hydrogenation to be 3 x -120 = -360 kJ/mol (-85.8 kcal/mol). Instead, the heat of hydrogenation of benzene is only -210 kJ/mol (-49.8 kcal/mol). The difference of 150 kJ/mol (36 kcal/mol) between the expected value and the experimentally observed value is the resonance energy of benzene. These experimental results are depicted in the form of a graph shown below. The resonance energy of benzene, as determined by a comparison of the heats of hydrogenation of cyclohexene, benzene, and the hypothetical benzene. The Mechanism of Aromatic Electrophilic Substitution Benzene is highly prone to electrophilic substitution reactions compared to addition reactions as it loses its aromaticity during addition reactions. As benzene contains delocalized electrons spanning over carbon atoms in the ring, it is highly attractive to electrophiles and is also highly stable to electrophilic substitutions. Generally, the electrophilic substitution reaction of benzene is a three- step process involving: Generation of the electrophile. Intermediate carbocation formation. Removal of a proton from carbocation intermediate. 11 12 Step 1: A-E → A- + E+ Reagent Step 2: π electrons of benzene attack the electrophile which takes two electrons of the six-electron aromatic system. This forms a σ bond between one carbon atom of the benzene ring and the electrophile. Because of the new sigma bond formed, this intermediate is called a sigma complex. Sigma complex This breaks the highly stable aromatic system since in the intermediate arenium ion, the carbon connected to the electrophile becomes sp3 hybridized and, therefore, cannot be part of the conjugated π bond system. This, energetically unfavorable process of interrupting aromaticity, is the slow-rate determining step of the reaction. On the other hand, the arenium ion has two conjugated double bonds, which in turn are conjugated with the empty p orbital of the positively charged carbon. It has three resonance forms, where the positive charge appears on three carbons and the resonance hybrid can be shown with these carbons having a partial positive charge: sigma complex hybrid Step 3: The hydrogen on the sp3-hybridized carbon is removed by a counterion/conjugate base restoring the aromaticity to the ring: The deprotonation is the driving force of the reaction making it energetically possible to proceed. The activation energy of this step is a lot smaller and the reaction occurs very fast. Energy profile diagram for electrophilic substitution reaction is depicted below. 12 13 Nitration of Benzene Benzene reacts with concentrated nitric acid at 323-333K in the presence of conc. sulphuric acid to form nitrobenzene. This reaction is known as nitration of benzene. The mechanism for nitration of benzene: Step 1: Nitric acid accepts a proton from sulphuric acid and then dissociates to form nitronium ion. Step 2: The nitronium ion acts as an electrophile in the process which further reacts with benzene to form an arenium ion. Arenium ion is resonance stabilised Step 3: The arenium ion then loses its proton to Lewis base forming nitrobenzene. Energy Profile diagram of nitration reaction Energy profile diagram for nitration of benzene 13 14 Presence of nitronium ion in the nitrating mixture has been confirmed by the following facts: i) A solution of nitric acid in sulphuric acid exhibits depression in freezing point consistent with the presence of 4 ions in solution - HSO4-, H3O+, NO2+, H2O+NO2 ii) Crystalline salts of NO2+ ion can be prepared – nitronium perchlorate (NO2+ClO4-) & nitronium nitrate (NO2+NO3-). The relative reactivities of aromatic compounds towards these nitronium salts are the same as their relative reactivities towards nitrating mixture. iii) The presence of nitronium ion in the nitrating mixture has been confirmed by Raman spectra. iv) Other reagents which generate nitronium ions (e.g., N2O5 or CH3COONO2) are also effective nitrating agents. These facts confirm the participation of nitronium ions in nitration reaction. Activating and deactivating substituents. Some groups in organic chemistry have electron donating ability and they can activate the benzene ring by increasing the electron density of benzene ring, are called activating groups. Since these type of groups activate the benzene ring, so they are called activator enhancer or activating groups. Activating groups are also called donating group. Most of the activating groups contain one or more lone pair of electron. This lone pair of electron participate in resonance with the pi electron of benzene ring. As a result, benzene ring with activating groups become electron rich. That is, benzene ring acts as a source of electrons. Therefore, benzene ring with activating group becomes more reactive towards electrophilic substitution reaction than benzene ring without activating groups. Activating groups increase the electron density of benzene ring due to their +I and +M effect. It has been found that, owing to presence of activating groups, the electron density increases on the ortho and para carbon atom of the benzene ring. So, in electrophilic substitution reaction, the electrophile attacks the ortho and para carbon atom only. Hence, activating groups which are already present in the benzene ring, indicate the incoming group to take the ortho and para position of the ring. For example, nitration of phenol gives ortho and para nitro phenol. That is, the –OH group of phenol which already exist in benzene ring , is ortho and para directing. Similarly, chlorination of toluene, gives ortho and para chloro toluene. So, –CH3 group is also an ortho and para directing group. In both cases, para product is predominant than ortho product, due to steric hindrance in ortho position. Phenol o-nitrophenol p-nitrophenol 14 15 For the above reason, activating groups are also called ortho, para directing groups. Groups which decrease the electron density of benzene ring owing to their –I effect as well as –M effect are called deactivating groups. In other words, groups which have electron accepting ability deactivate the benzene ring by reducing the electron density of benzene ring, are called deactivating groups. Since these type of groups deactivate the benzene ring, so they are called deactivating groups. Deactivating groups are also called electron withdrawing group. The – I effect of deactivating groups reduce the electron density from all the position of benzene ring. But, owing to –R effect of deactivating group, the electron density reduces, basically from ortho and para positions. Resonating structures of nitrobenzene molecule So, the density of electron at meta position remain comparatively more than ortho and para position. Hence, in electrophilic substitution reaction, the electrophile attack the meta position only. Nitration of benzaldehyde gives meta nitro benzaldehyde. That is, the –CHO group of benzaldehyde which already exist in benzene ring , is meta directing. Similarly, nitration of acetophenone, gives meta nitro acetophenone. So, –COCH3 group is also a meta directing group. Benzaldehyde m-nitrobenzaldehyde 15 16 Acetophenone m-nitroacetophenone For the above reason, deactivating groups also called meta-directing groups. Electron withdrawing -CN, -CHO, Electron density on Deactivating meta substituted groups -NO2, the benzene ring groups product decrease Electron donating -CH3, -OH, - Electron density on Activating Ortho / para groups NH2 the benzene ring groups substituted increase product Orienting influence of substituents towards electrophilic substitutions reactions of monosubstituted benzene (Z = electron donating substituent) Ortho attack Para attack Meta attack Toluene: Z = CH3 (ortho para director or activating group) Phenol: Z = OH (ortho para director or activating group) Nitrobenzene: Z = NO2 (meta director or deactivating group) Chlorobenzene: Z = Cl (ortho para director or activating group) Aniline: Z = NH2 (ortho para director or activating group) 16 17 Toluene: When the electrophile attacks the ortho position or the para position of toluene, it is seen that one of the resonating structures of the intermediate carbocation has the positive charge on the carbon atom having the – CH3 group. This form is stabilised to a greater extent due to the electron donating nature (+I effect) of the – CH3 group. However such a stabilisation of the intermediate carbocation is not possible when the attack of the electrophile occurs at meta position. Thus a more favourable transition state results when the attack occurs at o- / p- positions in toluene. Toluene on nitration gives mixture of o-nitro toluene and p-nitrotoluene. However if fuming nitric acid and sulphuric acid are used it gives 2,4,6-trinitrotoluene (TNT), which is used as a high explosive for military and industrial applications. It is also an intermediate in the production of dyes and photographic chemicals. Phenol: In phenol, the – OH group has – I effect (electron withdrawing) and this tends to make the intermediate carbocation less stable. However the lone pair of electrons on the oxygen atom of – OH group are involved in resonance (+R effect) with the π electron cloud of benzene ring. 17 18 In such cases where there is – I and +R effects operating, then the resonance effect overpowers the inductive effect. This increases the electron density on the ortho and para carbons when compared to meta carbon. As a result the electrophile prefers to attack o- / p- positions in phenol. Phenols upon treatment with dilute nitric acid undergo nitration at low temperature (298 K) to give a mixture of ortho and para nitrophenols. When phenol is treated with concentrated nitric acid, the nitration results in the formation of 2, 4, 6-trinitrophenol (commonly called picric acid). Nitrobenzene: Nitro group is a deactivating group. i.e., it is an electron withdrawing group. Hence attack of electrophile on nitrobenzene molecule is difficult. However if the reaction does takes place, it occurs slowly and gives meta product. When the electrophile enters the ortho or para carbon of nitrobenzene, one of the intermediate carbocation has a positive charge on the carbon carrying the nitro group. This destabilise the intermediate. Thus a more favourable transition state results when the attack occurs at m-position. 18 19 Thus chlorination of nitrobenzene gives m-chloronitrobenzene Chlorobenzene: Chlorine atom exhibits – I effect due to its electronegativity. Chlorobenzene is less reactive than benzene towards electrophilic substitution reaction due to –I effect. As chlorine is electron withdrawing group it deactivates the benzene ring and reduces the electron density on benzene ring and hence make the aromatic ring less reactive towards the upcoming electrophile. However, the chlorine substituent in chlorobenzene is donating electron density to the ortho and para positions of benzene ring through resonance (+R) because of the presence of lone pair of electrons on chlorine atom. +R effect of chlorine atom is shown below Electrophilic substitution of benzene depends on the π electron density of benzene ring. In chlorobenzene, presence of chlorine atom decreases the π electron density in the benzene ring due to electron withdrawing inductive (-I) effect of Cl. Electron releasing resonance effect (+R effect) is less operative here as chlorine has 3p orbital and carbon has 2p orbital. Due to difference in size of p orbitals resonance does not occur so effectively. As a result, the benzene ring is deactivated towards electrophilic substitution. Thus, reactivity is controlled by the stronger inductive effect, while the weaker resonance effect controls the orientation. 19 20 Thus nitration of chlorobenzene gives o- and p- products Comparison of stability of benzene, naphthalene and anthracene in terms of resonance energy Naphthalene Anthracene Phenanthrene The resonance energy for phenanthrene is 91 kcal/mol, that for anthracene is 83 kcal/mol and for naphthalene and benzene rings are 61 and 36 kcal/mol respectively. For polycyclic aromatic hydrocarbons, stability is said to be proportional to resonance energy per benzene rings. Here resonance energy per benzene ring decreases from 36 kcal/mol for benzene to 30.5 kcal/mol for naphthalene, 30.3 kcal/mol for phenanthene and 27.7 kcal/mol for anthracene. Hence, order of stability (or RE): benzene > naphthalene ~ phenanthrene > anthracene. In fact other fused polycyclic aromatic hydrocarbons react faster than benzene. 20 21 Birch reduction-statement with an example The Birch reduction can be classified as an organic redox reaction. Here, an organic reduction of aromatic rings in liquid ammonia with sodium, lithium or potassium and alcohol occurs. An example of a Birch reduction reaction is the reduction of naphthalene Benzene 1.4-cyclohexadiene Naphthalene 1,4,5,8-tetrahydronaphthalene Anthracene contains three aromatic rings and has a total resonance energy (i.e. the stabilization due to aromaticity) of 83 kcal/mol, or about 27.7 kcal/mol per ring (compare to 36 kcal/mol for benzene). Anthracene undergoes Birch reduction such that the central aromatic ring is reduced and a total of two hydrogens are added to the molecule. The resulting hydrocarbon has two aromatic rings for a total resonance energy of about 72 kcal/mol. Anthracene 9,10-dihydroanthracene Side chain oxidation of toluene to benzaldehyde The Étard reaction is a chemical reaction that involves the direct oxidation of an aromatic or heterocyclic bound methyl group to an aldehyde using chromyl chloride (CrO2Cl2). For example, toluene can be oxidized to benzaldehyde. Toluene Benzylidene chloride benzaldehyde Side chain oxidation of toluene to benzoic acid Toluene is oxidized with alkaline KMnO4 followed by acidification to form benzoic acid. Other oxidising agents that can be used are acidic KMnO4, acidic K2Cr2O7 and dil HNO3 etc. 21 22 Oxidation of Naphthalene (i) Naphthalene on oxidation using acidified KMnO4 gives phthalic acid; with alkaline KMnO4 it forms phthalonic acid Naphthalene Phthalic acid Naphthalene Phthalonic acid Naphthalene is oxidised to Phthalic Anhydride using H2SO4 & mercuric sulphate or by air in presence of V2O5 and is oxidised to 1,4-naphthoquinone using CrO3 Phthalic Anhydride Naphthalene 1,4-naphthoquinone Naphthalene Anthracene on oxidation using acidified potassium dichromate gives Anthraquinone. Anthracene Anthraquinone Phenanthrene on oxidation gives phenanthroquinone. Phenanthrene Phenanthraquinone Diels-Alder reaction The Diels-Alder reaction is a conjugate addition reaction of a conjugated diene to an alkene or alkyne (the dienophile) to produce a cyclohexene. The simplest example is the reaction of 1,3-butadiene with ethene to form cyclohexene. 22 23 The analogous reaction of 1,3-butadiene with ethyne to form 1,4-cyclohexadiene. Since the reaction forms a cyclic product, via a cyclic transition state, it can also be described as a "cycloaddition". The reaction is a concerted process: Example: Anthracene reacts with (i) maleic anhydride and (ii) 1,2-dichloroethene forming an adduct. (i) Anthracene Maleic anhydride 9,10-anthracene-succinic anhydride (ii) Styrene Styrene is an organic compound with the chemical formula C6H5CH=CH2. This derivative of benzene and is a colourless oily liquid, although aged samples can appear yellowish. The compound evaporates easily and has a sweet smell, although high concentrations have a less pleasant odour. Preparation of Styrene 23 24 Stilbene (E)-Stilbene, commonly known as trans-stilbene, is an organic compound represented by the condensed structural formula C6H5CH = CHC6H5. Classified as a diarylethene, it features a central ethylene moiety with one phenyl group substituents on each end of the carbon– carbon double bond. It has an (E) stereochemistry, meaning that the phenyl groups are located on opposite sides of the double bond, the opposite of its geometric isomer, cis-stilbene. Trans-stilbene occurs as a white crystalline solid at room temperature and is highly soluble in organic solvents. It can be converted to cis-stilbene photochemically, and further reacted to produce phenanthrene. cis-stilbene trans- stilbene (a) Reduction of benzoin using zinc amalgam gives trans stilbene Benzoin trans- stilbene (b) Decarboxylation of α-phenylcinnamic acid yields stilbene. E isomer of α-phenylcinnamic acid producing cis-stilbene and the Z-isomer producing trans-stilbene. The E isomer, in which the two phenyl rings are cis to each other. 24 25 Decarboxylation of E isomer of α-phenylcinnamic acid can be achieved using heat or ultraviolet light to produce trans-stilbene Heat or UV light E isomer of α-phenylcinnamic acid cis-stilbene Heat or UV light Z isomer of α-phenylcinnamic acid trans-stilbene cis-stilbene upon UV irradiation gets converted to tans-stilbene, a classic example of a photochemical reaction involving trans-cis isomerization Ullmann Reaction The Ullmann reaction (also known as Ullmann coupling) is an organic named reaction that involves the coupling of two aryl halides in the presence of copper to yield a biaryl as the product. This coupling reaction is named after the German chemist Fritz Ullmann. The general format of the Ullmann reaction is illustrated below. An illustration of the Ullmann reaction undergone by ortho-chloronitrobenzene in the presence of a copper-bronze alloy at a temperature of approximately 500 K to form 2,2’-dinitrobiphenyl 25 26 26