Midterm PDF - Biology Past Paper
Document Details
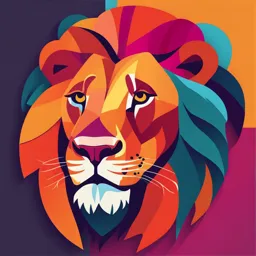
Uploaded by SelfSufficientSard8987
University of Regina
Tags
Related
Summary
This document examines fundamental principles of inheritance, focusing on Mendel's principles. It covers monohybrid and dihybrid crosses; probability rules; and the first and second laws of Mendelian genetics. It also includes topics on pedigree analysis, the chromosomal basis of Mendelism, and more.
Full Transcript
Topic 1 – Fundamental Principles of Inheritance Mendel’s controlled crosses - Monohybrid – crossing of two organisms that differ in one character (shape, colour), show the inheritance of a single trait - Dihybrid – crossing of two organisms that differ in two characters, helps in under...
Topic 1 – Fundamental Principles of Inheritance Mendel’s controlled crosses - Monohybrid – crossing of two organisms that differ in one character (shape, colour), show the inheritance of a single trait - Dihybrid – crossing of two organisms that differ in two characters, helps in understanding how the distribution of phenotypes of two traits segregates relative to each other. Allowed observation of individual assortment of alleles for different traits - Test Cross – crossing of an organism with a dominant allele with one with a recessive allele to determine the genotype of the organism with a dominant phenotype. Determines if it is RR or Rr Basic Probability Rules - Multiplicative rule o Calculates the probability of two individual events occurring together o P(A and B) = P(A) x P(B) o Example: calculating the probability of drawing an ace of hearts in a deck of card. You need to calculate the probability of drawing an ace, and in the hearts suit - Additive rule o Calculates the probability of either one or another of two mutually exclusive events o P(A or B) = P(A) + P(B) – P(A and B) o Example: calculating the probability of drawing either an ace or a king from a deck of cards First law of Mendelian Genetics - Evidence of dominance – phenomenon that one allele (dominant) can cover the expression of a different allele (recessive). Shown in monohybrid crosses. - Segregation of alleles – each individual has two alleles for every gene. They segregate during formation. Shows how genetic variation is maintained and inherited - Prediction of probabilities in crosses – predictions based on probability of different combinations of alleles coming together in fertilization - Overall shows the understanding of inheritance of traits and patterns Second law of Mendelian Genetics - Evidence of assortment in F2 progenies – evidence of independent assortment (independent segregation of alleles of different genes. Observed in dihybrid crosses or higher, where alleles of two or more segregate independently, leading to a variety of combinations - Identification and recombinant/parental phenotypes – allow for the identification of recombinant and parental phenotypes in the F2 progeny. Recombinant phenotypes are those that are new and come from the recombination of alleles. Parental phenotypes are those that resemble the parental combination - Prediction of probability in crosses – similar to first law. Predicts the probabilities in crosses that involves multiple genes - Derivation of the second law (segregation of multiple genes) – predictions can be done in a mathematical equation. Based on the principle that alleles of different genes segregate independently during formation - Overall shows the independent assortment of alleles, providing insight into inheritance patterns of multiple traits Chi-Square Test (c2) - Formulating null hypothesis o Prediction of distributions of phenotypes based on Mendelian ratios - Mathematical predictions based on Mendel’s Laws o Using Mendel’s laws to predict the number of progenies of each phenotype o Monohybrid crosses have an expected phenotypic ratio of 3:1, dihybrid is expected to be 9:3:3:1 - Degrees of freedom o df = number of phenotypic classes – 1 o Example: a dihybrid cross with 4 phenotypic classes, there degrees of freedom would be 3 - Interpreting c2 values o c2 = å (O – E)2 / E o O à observed number of progenies, E à expected number of progeny o Compare calculated value to the critical value to decide whether to accept or reject the hypothesis. If calculation is less than critical value it is accepted. Pedigree Analysis - Symbols represent the individuals, relationships, and genetic traits - Males are squares. Females are circles. Shaded means affected. Half shaded means they are a carrier Inheritance patterns - Autosomal dominant – at least one affected parent, trait appears in every generation - Autosomal recessive – can have unaffected parents, trait skips a generation - X-linked inheritance – genes on the X-chromosome. Males inherit it from their mothers but can skip generations for the females. Females are the carriers Calculating probability of each phenotype - Autosomal dominant – one affected parent = 50% chance the child is affected - Autosomal recessive – both parents are affected = 25% chance of child being affected - X-linked – if the mother is a carrier = son has 50% chance of being affected TOPIC 2 – Chromosomal Basis of Mendelism Chromosome behaviour in meiosis + second law of Mendel - Homologous chromosomes pair up and go through crossing over (genetic recombination). This randomly realigns the homologous chromosomes on the metaphase plate (during metaphase I). This results in independent assortment - The random orientation will produce different daughter cells, giving a random chance of every outcome. The randomness is shown in the F2 phenotypic ratios - The behaviour or chromosomes during meiosis dictates the inheritance pattern. Therefore, explaining the equal but random segregation of alleles that is in Mendelian ratios X-linkage + Chromosomal Theory of Inheritance - X-linkage – inheritance pattern of genes on the X-chromosome. Discovered by Thomas Hunt Morgan via drosophila. Found that certain traits are linked to the sex of the organism - Chromosomal Theory of Inheritance (CTI) – principle that states that’s genes are located on the chromosomes and that its behaviour during meiosis explains the pattern of inheritance. Provided a cytological explanation for Mendel’s ratios, and introduced the concept of sex-linkage, where genes are physically linked to sex chromosomes - The development of both established that chromosomes are actual units of segregation. This led to a paradigm shift in the understanding of genetics Non-disjunction as proof of the CTI - Non-disjunction – the failure of homologous chromosomes (or sister chromatids) to separate properly during cell division. Serves as evidence supporting the CTI - Non-disjunction events result in production of gametes with an incorrect number of chromosomes which leads to aneuploidy (abnormal number of chromosomes in haploid set) - Occurrence of non-disjunction events is evidence of CTI. It demonstrates the behaviour of chromosomes during meiosis influences the inheritance patterns. Defects in cell division that leads to abnormal numbers of chromosomes, like what is seen in non- disjunction - Non-disjunction serves as proof of CTI by highlighting the link between chromosomal behaviour during meiosis and the inheritance of genetic traits Sex as a phenotype (flies and humans) - Sex is considered a phenotype, an observable characteristic from one’s genetic makeup - Drosophila – sex determination is based on ratio of sex chromosomes to autosomes. Males are heterogametic (XY), females are homogametic (XX). Presence of Y chromosomes determines maleness, but sex determination is not solely dependent on the presence of it, but the balance of X chromosomes and autosomes - Humans – sex determination based on presence of chromosomes. Males are XY, females are XX. The presence of the SRY gene on the Y chromosome triggers the development of male reproductive structures (penis) Sex linked inheritance in humans - Refers to the inheritance patterns of genes located on the sex chromosome. This leads to distinct patterns of segregation in X-linked recessive/dominant diseases - Patterns of segregation o Genes causing the X-linked disorders are located on the X chromosome o X-linked recessive - Males more commonly affected because they only have one X chromosome. If it carries the recessive allele, they will express the disorder. Females are less frequently affected because they need to inherit two copies of the recessive allele to express the disorder o X-linked dominant – Both male and female can be affected but has different inheritance patterns. Males inherit it form their mothers; females can inherit it from either parent - Calculations of Probabilities o X-linked recessive – males have higher probability than females o X-linked dominant – probability of males and females expressing the disorder depends on the specific inheritance pattern and if it is inherited from the mother or father Cytogenetics - Focuses on the study of chromosomes and their role in inheritance - Karyotype – visual representation of complete set of chromosomes in an organism. Provides detailed picture of the number, size, and shape of chromosomes in a cell - Ploidy – number of sets of chromosomes in a cell. Example: humans are diploid, meaning they have two sets of chromosomes (2n). Some plants and animals can be polyploidy meaning two sets of chromosomes - Polyploidy – organism with more than two sets of chromosomes. Can result from errors in cell division or hybridization between species. Significant consequences include increased cell size, altered metabolism, and creation of new species - Aneuploidy – abnormal number of chromosomes usually due to non-disjunction during meiosis. Can lead to developmental disorders and genetic diseases. Examples: Trisomy (extra chromosome), Monosomy (missing a chromosome) - Gene dosage – monosomies and trisomies can lead to imbalances in gene dosage, affected the expression of genes. Trisomies of autosomes often result in embryonic lethality, while trisomy of chromosomes 21 (down syndrome) is a notable exception. Monosomy of autosomes is typically lethal, but monosomy of the X chromosomes (turners syndrome) is viable in humans - Chromosomal Rearrangements o Deletion – loss of a segment of a chromosome. This can lead to the loss of specific genes are result in a genetic disorder o Duplication – presence of extra copies of a chromosomal segment. This can lead to gene redundancy or altered gene expression o Translocations - occur when segments of chromosomes break and reattach to non-homologous chromosomes. This can lead to gene disruption or altered expression o Inversions – the reversal of a segment of a chromosome. This can lead to changes in gene expression and potential genetic disorders TOPIC 3 – Extensions of Mendelian Laws Mechanisms to explain recessiveness and dominance - Recessiveness o Haplosufficiency – recessive alleles are often associated with “loss of function” mutations, where a single function copy of the gene (in a heterozygote state) is sufficient to produce a wild-type phenotype. Genetic conditions that occur when one cell has an inactivated or deleted copy of a gene, the remaining is unable to produce enough of the gene’s product alone o Incomplete or Loss of Function – recessive alleles typically result in incomplete or loss of function of the gene product, this may not significantly affect the phenotype when one functional copy is present - Dominance o Haploinsufficiency – in some cases, a single functional copy of the gene is not sufficient enough to produce the wild-type phenotype. The presence of only one functional allele is not enough to compensate for the loss of function caused by the mutant allele o Dominant Negative Interactions – some dominant alleles produce abnormal gene products that interfere with the function of wild-type gene products. Dominant negative effect can lead to a dominant phenotype, even in the presence of a single copy of the mutant allele Allele interactions - Multiple alleles on a single locus – multiple alleles at a single locus creates an allelic series, which describes the dominance hierarchy of these alleles. Interactions between multiple alleles can lead to a wide range of phenotypic variations, influencing traits in a diverse way - Semi-Dominance – the heterozygous phenotype is the immediate between the two homozygous phenotypes. This ends up with a 1:2:1 phenotypic ratio in the F2 generation, instead of the 3:1 Mendelian ratio. Example: red flower (RR) and white flower (rr), are crossed and produce a pink flower (Rr). - Co-Dominance – both alleles at a locus are fully expressed in the heterozygote condition, leading to a distinct phenotype. F2 generation has a 1:2:1 phenotypic ratio. Example: Red flower (RR) is crossed with a white flower (rr) results in a flower with red and white petals (Rr) - Lethal Alleles – cause the death of an organism when present in a homozygous state, leading to a modified phenotypic ratio in the surviving offspring - Variable Penetrance – situation where individuals with the same genotype do not express the expected phenotype. This can lead to deviations from the predicted phenotypic ratios - Variable expressivity – range of phenotypic manifestations of a particular genotype. Can lead to variations in the severity or extent of the phenotype, contributing to deviations from expected ratios. Range of severity or degree that an individual expresses a gene - Pleiotropy Inheritance – single gene can influence multiple, unrelated phenotypic traits. Leads to unexpected phenotypic outcomes, and complicate predicted ratios - Polygenic Inheritance – many traits influenced by combined effects of genes. Interactions can lead to continuous variation in phenotypes, deviating from Mendelian ratios Sorting out allelism: completion tests - Completion tests – determines how many genes (known as completion groups) are involved in a given trait. Failure to complete indicates allelism (once locus involved), while completion indicated non-allelic mutants (more than one gene responsible for the same phenotype). Cross between organisms to see if their mutations are on the same gene or different ones Pathway analysis + biochemical supplementation - Organizing genes in a pathway based on mutant’s growth responses. Supplementation with specific biochemicals downstream form a block in the pathway can reveal groups of allelic mutations and provide insights into the functional organizations of genes in metabolic pathways o Precursor à B à C àD o Precursor à A àD o Mutant 3 synthesizes compound D in the presence of A but not B or C o Mutant 2 synthesizes compound D in the presence of C but not A or B Suppressor and enhancer mutations - Suppressor Mutation – suppresses effects of mutations - Enhancer Mutation – enhances effects of mutations - Synthetic Lethal Mutations – combination of two mutants leads to cell death, even though each mutation is viable when alone Gene interaction detected by changes in the expected F2 phenotypic ratios - Additive Gene Action – involves genes in independent pathways influencing the same phenotype. This can lead to a 9:3:3:1 phenotypic ratio. Multiple genes contribute equally to a phenotype - Complementary Gene Action – genes in the same linear pathway fully complement each other, leading to a 9:7 phenotypic ratio (9:3:3:1 ratio, but adding 3+3+1) - Duplication Gene Action – two (dominant) genes independently performing the same step in a pathway, leading to a 1:5:1 phenotypic ratio - Epistasis – recessive and dominant epistasis can modify the classical 9:3:3:1 ratio into 9:3:4 (recessive) and 1:2:3:1 (dominant) ratios TOPIC 4 – Linkage and Genetic Mapping Signatures of linkage in F2 and TC progenies - Signatures of linkage are shown by the proportions of parental and recombinant phenotypes. When linked, the rarity of crossing over predicts less combination than expected if the alleles were segregating independently. This leads to a decrease in frequency of recombinant phenotypes and more parental phenotypes. This deviates from Mendel’s predictions, and indicates that presence of linkage between genes. Linkage phases - Coupling – genes flowing down to F1 from a single P0 parental. Both recessive alleles are on one parent - Repulsion – genes segregate form different P0 parentals. One recessive allele from each parent Evidence of crossing over as the mechanism for recombination - No crossing over – outcome is 100% the parental gametes - Crossing over before DNA duplication – outcome is 100% recombinants. DNA exchanges before DNA replication is completed - Crossing over after meiosis I – outcome is 100% parental gametes. DNA exchange after segregation is completed - Crossing over during meiosis I – 50% parental, 50% recombinant. The actual way it is done. Maximum recombinant percentage can only be 50% Multiple crosses on loci - Very, very rare - Effect does not always change the phenotypic ratio - Even numbers of crossing over will recoup the parental information and become undetectable as a recombinant. The shuffling cancels it out, and alleles remain as the original chromatid - Leads to a general underestimation of recombination frequency Recombination frequency - RF = (# of total recombinant phenotypes) / (total # of progeny) - In a test cross the tester is recessive - F2 progeny will show the frequency of recombinant gametes in the hybrid - Test cross example: test cross results in 400 dominant phenotype, and 100 progenies with recessive. RF = 100/500 = 0.2. 20% will have the recombinant phenotype. - F2 cross example: cross results in 300 double dominants, 100 with double recessive, 50 with one dominant and one recessive, and 50 with the other dominant and recessive. RF = (50 + 50) / 500 = 0.2. 20% will be a recombinant Genetic mapping and RFs - RF shows the percent of recombinant progenies from a cross - Map distance (centimorgans, or map units) represent the genetic distance between two loci - These two directly correlate. As the map distance between two genes increases, so does the RF. These correlate to a certain point (around 7 m.u, or 7% recombinant). Past that, the RF begins to underestimate the map distance Physical and genetic maps - Physical maps o Represents the physical position of the genes, DNA markers, and other features on the chromosome/genome o Based on physical distances measures in base pairs (bp) or kilobases (kb) o Distances can be affected by recombination hotspots (high recombination rates) and cold spots (low recombination rates) - Genetic map o Also known as a linkage map o Represent the relative positions of genes on a chromosome/genome based on RF and map distances o Based on the genetic distances measured in cM or m.u. - The shorter the distance, the more linear the correlation is - Genetic maps give an indirect estimation of physical distance - Distance between two points on a genetic map of a chromosome is the average number of crossovers between them - Linked genes can be mapped relative to each other on a chromosome by studying how often their alleles recombine Building maps with two-point crosses - Used to determine the recombination frequencies between pairs of genes. Crosses between individuals heterozygous for two genes of interest 1. Establish individual distances – calculate RF of each pair of genes based on progeny phenotype. Convert RF to map units 2. Integration of distances – when individual distances are established, integrate the distances on a genetic map for three or more genes. Consider the relative order and distance between each pair of genes to construct a genetic map that reflects the arrangement of genes 3. Correcting for interference and multiple crossing over – crossover interference can affect the accuracy of genetic distances. Use mapping functions to adjust for unseen extra crossing over 4. Using mapping functions – Haldane’s mapping function Building maps with three-point crosses 1. RF from trihybrid test cross – analyze results from test cross to determine the RF between the three genes. Calculate the RF 2. Identifying parental, recombinants, and double recombinants – identify and classify the progenies into distinct categories to differentiate them from parentals, recombinants, and double recombinants 3. Identify the middle gene – analyze the double recombinants. The gene that switches between the double and parental is classified as the middle gene 4. Correcting RFs for the doubles 5. Integrating map distances – when individual RFs are correct, integrate the map distances. Consider the relate order and distance between each pair, as well as the middle gene Limitations of genetic mapping - Underestimation of crossing over events o As the distance between loci increase, the higher the occurrence of crossing over is o Mapping functions are applied to correct for the underestimation. Helps readjust the RFs to account for the complexities - Loss of linear correlation o Genetic mappings shows loss of linear correlation around 7-8 cM o This leads to challenges in estimating the physical distances between genes - Low-quality genetic maps o Genetic mapping may yield low-quality maps where genes are poorly characterized o Creates challenges in accurately telling the relative positions and distances between genes, leading to less reliable maps Measuring distances by integrating maps past 50 cM - Infer distances based on linked markers o Infer distance within the 50 cM. these can serve as a reference point - Intermediate markers o Markers in the 50 cM can bridge the gap and infer the genetic distances past 50 - Consider multiple crosses o Use multiple crosses and analyze the inferred genetic distances. From this it is possible to estimate - Complex mapping o Mapping over 50cM becomes more complex