Microbiology Final Study Guide PDF
Document Details
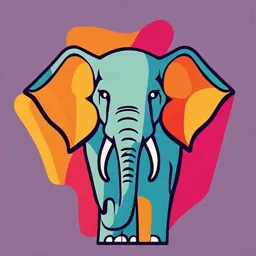
Uploaded by HarmoniousDenouement
University of Nebraska Omaha
Tags
Summary
This study guide is for a microbiology final exam and focuses on physical and chemical control methods for microbes. It includes definitions of key terms like sterilization, disinfection, and antisepsis, and discusses factors impacting the effectiveness of microbial control agents. The guide also explores various physical methods such as heat, filtration, and radiation.
Full Transcript
2440 – Final Exam – Study Guide (new material portion only) Chapter 7 – Physical and Chemical Control of Microbes 1. What is the germ theory of disease? The germ theory of disease states that microorganisms, also known as germs, are the cause of many diseases...
2440 – Final Exam – Study Guide (new material portion only) Chapter 7 – Physical and Chemical Control of Microbes 1. What is the germ theory of disease? The germ theory of disease states that microorganisms, also known as germs, are the cause of many diseases. 2. Be able to define sterilization. When would it be used? Sterilization is the process of completely eliminating all forms of microbial life, including bacteria, viruses, fungi, and spores, from an object or surface. It is used in situations where the highest level of microbial control is needed, such as in medical and surgical instruments, laboratory equipment, and food production facilities. 3. What is commercial sterilization and why is it used? Commercial sterilization is a process used in food preservation, particularly in canning, where foods are treated to kill or inactivate harmful microorganisms, including spores of Clostridium botulinum. It doesn't necessarily kill all microorganisms, but it ensures the food is safe for consumption by targeting pathogens that could cause foodborne illness. 4. Be able to define disinfection. When would it be used? What is a disinfectant? Disinfection is the process of reducing the number of viable microorganisms to a level that is not harmful to health. Disinfection is typically used on inanimate objects or surfaces, such as countertops, medical equipment, or floors, where the goal is to eliminate or reduce harmful pathogens. A disinfectant is a chemical agent used to carry out disinfection, often used on surfaces that cannot tolerate the intensity of sterilization. 5. Be able to define antisepsis. When would it be used? What is an antiseptic agent? Antisepsis refers to the process of eliminating or inhibiting the growth of microorganisms on living tissue, such as skin or mucous membranes. It is used to prevent infections, especially before surgical procedures or after an injury. An antiseptic agent is a chemical substance that prevents or inhibits the growth of microorganisms on living tissue. Examples include alcohol, iodine, and hydrogen peroxide. 6. Be able to define decontamination. When would it be used? Decontamination refers to the process of removing or neutralizing harmful substances, including microorganisms, from a surface or object. It is a broader term that includes cleaning, disinfection, and sterilization and is used when handling potentially contaminated materials, such as laboratory equipment, clothing, or waste from healthcare environments. 7. Be able to define de-germing. When would it be used? De-germing refers to the physical removal or reduction of the number of microorganisms from the skin, typically by scrubbing or washing with soap or other cleaning agents. It is commonly used in healthcare settings before procedures such as injections or surgeries to minimize the risk of infection. 8. Be able to define sanitation. When would it be used? Sanitation is the process of cleaning and reducing the number of microorganisms to a safe level, typically in food handling, water treatment, or public health contexts. It aims to meet health standards and prevent the spread of diseases. It is used in restaurants, food production facilities, and public restrooms to ensure hygiene. 9. What does the suffix “-cide” mean? The suffix “-cide” refers to the killing of a particular type of microorganism. For example, bactericide refers to a substance that kills bacteria, and fungicide refers to a substance that kills fungi. 10. What does the suffix “-static” mean? The suffix “-static” refers to the inhibition or slowing down of microbial growth, rather than killing the microorganisms. For example, bacteriostatic refers to an agent that inhibits the growth of bacteria, and fungistatic refers to an agent that inhibits the growth of fungi. 11. What are fomites? Fomites are inanimate objects or surfaces that can carry and transfer infectious microorganisms. Examples include doorknobs, countertops, medical instruments, or towels. These objects can harbor pathogens and contribute to the spread of infections if they come into contact with humans. 12. What are the factors that influence microbial agent effectiveness? The effectiveness of a microbial control agent is influenced by several factors: Concentration of the agent: Higher concentrations of a disinfectant or antiseptic tend to be more effective. Temperature: Higher temperatures can enhance the activity of antimicrobial agents. Time of exposure: The longer the exposure to a control agent, the more effective it generally is. Microbial characteristics: Some microorganisms are more resistant to control agents (e.g., bacterial spores, mycobacteria). Presence of organic matter: Organic materials like blood, feces, or dirt can protect microorganisms from the effects of the antimicrobial agent. 13. Be able to describe the 4 main ways that microbial control agents kill or inhibit microbes (altering cell membrane permeability, disrupting cell walls, damaging proteins, damaging nucleic acids). Altering Cell Membrane Permeability: Microbial control agents can disrupt the lipid bilayer of the cell membrane, leading to leakage of vital intracellular substances, ultimately causing cell death. Examples include surfactants like detergents. Disrupting Cell Walls: Certain agents (e.g., penicillin) inhibit the formation of the cell wall, leading to osmotic pressure changes and cell lysis. Bacteria without cell walls (e.g., Mycoplasma) are less susceptible to this method. Damaging Proteins: Microbial control agents, such as heat or chemicals, can denature proteins, making them lose their functional structure, thus disrupting cellular processes. This can occur through the breaking of bonds in protein molecules, rendering them nonfunctional. Damaging Nucleic Acids: Chemicals like radiation or certain chemicals (e.g., formaldehyde) can damage DNA or RNA, preventing replication or transcription, which ultimately halts cell division and can lead to cell death. 14. What are the physical methods of microbial control that we discussed in class? The main physical methods of microbial control include: Heat (both dry and moist) Filtration (for air and liquids) Radiation (e.g., UV light, gamma rays) Desiccation (drying out microorganisms) Low temperatures (refrigeration and freezing) 15. Be able to describe how heat controls microorganism growth. Heat kills microorganisms primarily by denaturing their proteins, disrupting their cell membranes, and damaging nucleic acids. The effectiveness of heat is temperature-dependent, with higher temperatures being more lethal. It can also cause the destruction of enzymes, which are crucial for cellular processes. 16. What is the difference between dry-heat and moist-heat? Dry Heat: Involves higher temperatures (e.g., in ovens or incinerators) and works by oxidizing cellular components and denaturing proteins. It requires higher temperatures and longer exposure times compared to moist heat. Examples include hot-air sterilization. Moist Heat: Involves the use of steam or water vapor, which transfers heat more efficiently than dry heat. Moist heat is typically more effective at lower temperatures and shorter exposure times. Examples include boiling, autoclaving, and pasteurization. 17. Be able to describe pasteurization and why it is used in place of other heating methods. Pasteurization is a process that uses moderate heat (usually between 60–85°C) to kill pathogens and reduce the number of spoilage microorganisms in food and beverages without affecting their taste or quality. It is commonly used in dairy products, juices, and wines. Unlike sterilization, which kills all microorganisms, pasteurization only reduces microbial numbers to a safe level and does not affect the nutritional content or flavor of the product. 18. Be able to describe how high-pressure controls microorganism growth. High pressure, typically applied in the range of 100 to 800 MPa, can be used to kill or inhibit the growth of microorganisms. This process, known as high-pressure processing (HPP), affects the integrity of microbial cell membranes, enzymes, and DNA, leading to inactivation. It is commonly used in food preservation, particularly for products like juices and ready-to-eat meals, as it can be applied without the need for high temperatures, thus preserving the food’s taste and texture. 19. What is an autoclave? In general, how does it work? An autoclave is a device used for sterilizing equipment and materials by applying high-pressure steam. It works by raising the temperature of water to 121°C (250°F) under pressure, which kills bacteria, viruses, and spores. Autoclaves are commonly used in medical and laboratory settings to sterilize surgical instruments, glassware, and other equipment. 20. Be able to describe how low temperature controls microorganism growth. Low temperatures, such as refrigeration (around 4°C or 39°F) and freezing (below 0°C or 32°F), slow down or inhibit the growth of microorganisms. While freezing can kill some microbes, most pathogens simply become dormant and will not grow. Refrigeration slows the metabolic rate of microorganisms, thus extending the shelf life of perishable items by preventing rapid microbial reproduction. 21. What is desiccation? Be able to describe how desiccation controls microorganism growth. Desiccation is the process of drying out, which removes the water necessary for the growth and reproduction of microorganisms. Without sufficient water, most bacteria, fungi, and other microorganisms are unable to function or replicate, effectively halting their growth. This can be used as a method of preservation for food, biological samples, or other materials. In desiccation, microorganisms may not necessarily die, but their metabolic processes are slowed or completely stopped. 22. How does high salt and high sugar contribute to desiccation? High concentrations of salt or sugar create a hypertonic environment, where the concentration of solutes outside the microorganism is higher than inside the cell. This causes water to leave the cell through osmosis in an attempt to balance the solute concentrations. As the water leaves the microorganism, it becomes dehydrated, which can inhibit metabolic functions and lead to the death of the organism. This is why high salt and sugar concentrations are often used in food preservation, such as in curing meats or making jams. 23. Be able to define filtration. - Be able to describe how filtration controls microorganism growth. Filtration is a physical method used to remove microorganisms from liquids and air by passing them through a filter with pores small enough to trap them. It is especially useful for sterilizing heat-sensitive liquids, such as vaccines or culture media, where heat sterilization (like autoclaving) would damage the substance. Filtration works by physically removing the microbial cells from the medium, thus preventing their growth or further contamination. 24. What is a high efficiency particulate air (HEPA) filter? A HEPA filter is a type of air filter that is designed to trap very fine particles, including microorganisms such as bacteria, viruses, and spores. These filters are capable of capturing particles as small as 0.3 microns with an efficiency of 99.97%. HEPA filters are used in environments like hospitals, laboratories, and clean rooms to control airborne contamination and protect both personnel and the environment from microorganisms. 25. What is ionizing radiation? Be able to describe how it controls microorganism growth (including what it does to the DNA). Ionizing radiation refers to high-energy radiation (e.g., gamma rays, X-rays, or electron beams) that has enough energy to remove tightly bound electrons from atoms, creating ions. This radiation can damage the DNA of microorganisms by breaking the chemical bonds between the bases of the DNA molecule or causing single- and double-strand breaks. This DNA damage can lead to mutations, and in many cases, the microorganism becomes nonviable and is unable to replicate. Ionizing radiation is used in sterilization processes for medical equipment, food preservation, and sterilizing biological materials. 26. What is nonionizing radiation? Be able to describe how it controls microorganism growth (including what it does to the DNA). Nonionizing radiation includes ultraviolet (UV) light, which has less energy than ionizing radiation. While it doesn’t remove electrons from atoms, it can still cause significant damage to microbial DNA. Specifically, UV light causes thymine dimers to form, where adjacent thymine bases in the DNA strand bond with each other, rather than pairing with adenine. This distortion of the DNA prevents proper replication and transcription, leading to mutations and cell death if the damage is severe. Nonionizing radiation is commonly used to disinfect air, water, and surfaces in areas like hospitals and laboratories. 27. What are important things to consider for chemical disinfectants? When selecting and using chemical disinfectants, several factors should be considered: Spectrum of Activity: The disinfectant should be effective against a broad range of microorganisms, including bacteria, viruses, fungi, and spores. Concentration: The concentration of the disinfectant must be sufficient to kill microorganisms, but not so high as to be harmful to surfaces or users. Contact Time: The disinfectant must be in contact with the microorganisms for a sufficient period of time to be effective. Toxicity: The potential toxicity to humans and the environment should be assessed. Temperature and pH: Some disinfectants may require specific temperatures or pH levels to work effectively. Corrosiveness: The disinfectant should not be overly corrosive to surfaces, especially in environments where materials are sensitive. 28. What are the methods mentioned in class to evaluate the effectiveness of a disinfection? Common methods to evaluate the effectiveness of disinfectants include: Disk Diffusion Method: This method involves placing a disinfectant-soaked paper disk on an agar plate inoculated with microorganisms. The zone of inhibition (clear area) around the disk indicates the effectiveness of the disinfectant in killing or inhibiting microbial growth. Minimum Inhibitory Concentration (MIC): This measures the lowest concentration of a disinfectant that inhibits microbial growth in a liquid culture. Minimum Bactericidal Concentration (MBC): This is the lowest concentration of a disinfectant that kills the microorganism, rather than just inhibiting its growth. Time-Kill Test: This test assesses the effectiveness of a disinfectant by measuring the number of surviving microorganisms after a given period of exposure. 29. What are the main types of chemical disinfectants discussed in class? Some of the main types of chemical disinfectants include: Alcohols (e.g., ethanol, isopropanol): Used to disinfect surfaces and skin. They are effective against bacteria and enveloped viruses. Aldehydes (e.g., formaldehyde, glutaraldehyde): Strong disinfectants used in sterilization, especially in medical settings. Halogens (e.g., chlorine, iodine): Used in water treatment, surface disinfection, and wound care. Phenols (e.g., lysol): Used to disinfect surfaces and sometimes in antiseptics. Quaternary Ammonium Compounds (Quats): Commonly used in surface disinfection in hospitals and food industries. Peroxides (e.g., hydrogen peroxide): Used for surface and wound disinfection and sterilization. 30. What are surfactants? Be able to describe how they control microorganism growth. Surfactants are chemical compounds that reduce the surface tension of water, allowing it to more easily spread and penetrate surfaces. They typically have both hydrophilic (water-attracting) and hydrophobic (water-repelling) parts. Surfactants work to control microorganism growth by disrupting the cell membranes of bacteria, fungi, and other microorganisms, causing leakage of cellular contents and ultimately cell death. Surfactants can also function as detergents, removing dirt and organic material, which may otherwise shield microorganisms from disinfectants. Examples include soaps, detergents, and some disinfectant cleaners. 31. What are detergents? What is the difference between cationic and anionic detergents? Detergents are surfactants that reduce the surface tension of water, making it easier to remove dirt, oil, and microorganisms from surfaces. They are commonly used in cleaning and disinfecting applications. Detergents consist of molecules with hydrophilic (water-loving) and hydrophobic (water-repelling) regions, which help them to break up oils and dirt and facilitate their removal Cationic detergents: These detergents have a positively charged hydrophilic head. They are often more effective as disinfectants due to their ability to disrupt the cell membranes of microorganisms, leading to cell death. They are commonly used as antiseptics and disinfectants in hospitals. An example is quaternary ammonium compounds (quats). Anionic detergents: These detergents have a negatively charged hydrophilic head. They are primarily used for cleaning purposes, as they emulsify oils and suspend dirt, but they are generally less effective at killing microorganisms. They are commonly found in household soaps and detergents, such as sodium lauryl sulfate. 32. Be able to describe the use of acid anionic sanitizers in controlling microorganisms (how do they work?) What are the advantages/disadvantages? Acid anionic sanitizers are disinfectants that contain negatively charged ions (anions) and an acidic solution. They are commonly used in food-processing environments and for sanitizing surfaces. The mechanism by which acid anionic sanitizers work involves disrupting the microbial cell membrane, which leads to leakage of cellular contents, ultimately causing cell death How they work: Acid anionic sanitizers are effective against a wide range of microorganisms, especially Gram-positive bacteria. They do not penetrate the cell membrane as effectively as some other disinfectants but can still be effective in removing surface contaminants. Advantages: a. They are non-corrosive and can be safely used on various surfaces, including food-contact surfaces. b. They are relatively safe for use in food processing and are effective at low concentrations. Disadvantages: c. They are not as effective against all types of bacteria, particularly Gram-negative bacteria. d. Their effectiveness may be reduced in the presence of organic material (e.g., food residues). 33. Be able to describe the use of phenolics in controlling microorganisms? (What are they? how do they work? What are the advantages/disadvantages? Phenolics are a class of chemical compounds derived from phenol (carbolic acid) and are widely used as disinfectants. They are effective against a broad spectrum of microorganisms, including bacteria, fungi, and viruses. How they work: Phenolics disrupt the cell membrane of microorganisms and denature proteins, leading to cell leakage and cell death. They also inactivate enzymes necessary for microbial metabolism. Advantages: a. Phenolics are effective against a broad range of microorganisms, including some that are resistant to other disinfectants (e.g., tuberculosis bacteria). b. They remain active on surfaces for extended periods, providing residual antimicrobial effects. Disadvantages: c. Phenolics can be toxic to humans and animals if ingested or inhaled, and they can be irritating to the skin and mucous membranes. d. They have a strong, unpleasant odor. e. They can be corrosive to some metals and plastics. 34. Be able to describe how heavy metals control microorganism growth. Heavy metals such as mercury, silver, and copper can inhibit microbial growth by binding to proteins and enzymes within the microorganism, leading to denaturation of these proteins and disrupting cellular processes. The metal ions may also interfere with the microorganism’s ability to transport substances across its cell membrane. How they work: Heavy metals inactivate enzymes and can bind to sulfur-containing groups in proteins, resulting in loss of protein function. This damages the microorganism's metabolism, leading to its death. 35. What were the examples given for the use of mercury, silver, and copper? Mercury: Historically used in the form of mercurochrome and other mercury-based compounds for antiseptic purposes, but it is now largely avoided due to its toxicity and environmental impact. Silver: Used in wound dressings, catheters, and some medical devices due to its antimicrobial properties. Silver ions can kill bacteria by binding to their cellular components. Copper: Used in antimicrobial surfaces, such as copper-based alloys in hospitals, as copper ions can damage bacterial cell membranes and proteins. Copper is also used in water treatment systems. 36. What are the 2 halogens discussed in class? The two halogens most commonly discussed in microbiology for antimicrobial purposes are: Chlorine Iodine 37. Be able to describe how iodine is used to control microbes. What is it? How does it work? What the advantages/disadvantages of using iodine? Iodine is a halogen used as an antiseptic and disinfectant. It is typically used in the form of iodine tinctures or iodine-based compounds like povidone-iodine. How it works: Iodine works by penetrating the microbial cell and binding to the amino acids and proteins, disrupting their function and structure. It also inactivates enzymes, leading to microbial death. Advantages: a. Effective against a wide range of microorganisms, including bacteria, viruses, and fungi. b. Relatively safe for use in wounds and for skin disinfection. Disadvantages: c. It can cause skin irritation or allergic reactions in some individuals. d. It may stain clothing and skin. e. It is less effective in the presence of organic matter. 38. How is chlorine used to control microbial growth? Chlorine is used to disinfect drinking water, swimming pools, and surfaces in food-processing environments. It is commonly used in the form of sodium hypochlorite (bleach) or chlorine gas. How it works: Chlorine works by releasing chlorine gas (Cl₂) or hypochlorite ions (OCl⁻), which are highly reactive and can inactivate enzymes and proteins in microorganisms, leading to cell death. Chlorine is particularly effective at killing bacteria and viruses in water. Advantages: a. It is an effective disinfectant against a wide range of microorganisms, including bacteria, viruses, and protozoa. b. It is widely used for water treatment and surface disinfection. Disadvantages: c. It can be toxic if ingested or inhaled in high concentrations. d. It may react with organic matter in water, forming harmful by-products such as trihalomethanes (THMs). e. Chlorine can be corrosive to certain materials. 39. Be able to describe how alcohols are used to control microbial growth. What are they? How do they work? What are the advantages/disadvantages of using alcohols? Alcohols (commonly ethanol and isopropanol) are widely used as disinfectants for skin, surfaces, and in laboratory settings. How they work: Alcohols work by denaturing proteins and dissolving lipids, effectively disrupting cell membranes and leading to cell leakage and death. Alcohols also have limited ability to penetrate the cell, so they are more effective against the outer layers of microorganisms. Advantages: a. Alcohols are effective against a broad range of microorganisms, including bacteria, fungi, and some viruses. b. They dry quickly and leave minimal residue. Disadvantages: c. Alcohols are not effective against bacterial spores. d. They can be drying or irritating to the skin with prolonged use. e. Alcohols are flammable, which can pose a safety risk. 40. Be able to describe the use of essential oils to control microbial growth. Essential oils are plant-derived compounds that have natural antimicrobial properties. Some common examples include tea tree oil, eucalyptus oil, and lavender oil. How they work: Essential oils contain compounds such as terpenes, phenols, and aldehydes, which can disrupt cell membranes, inhibit enzyme function, and alter the structure of proteins within microorganisms, leading to their death. Advantages: a. They are natural and often considered safer for use in the environment compared to synthetic chemicals. b. Many essential oils have broad-spectrum antimicrobial activity, effective against bacteria, fungi, and viruses. Disadvantages: c. Their effectiveness can vary widely depending on the type of oil and microorganism. d. Essential oils can be toxic to humans or animals if ingested or applied in high concentrations. e. They are generally less potent than chemical disinfectants and may require longer exposure times to be effective. 41. Be able to describe the use of aldehydes to control microbial growth. Aldehydes are highly effective chemical disinfectants used to control microbial growth. Examples include formaldehydeand glutaraldehyde. How they work: Aldehydes are highly reactive compounds that can cross-link the amino and sulfhydryl groups in proteins, nucleic acids, and other macromolecules in microbial cells. This leads to the inactivation of enzymes and the structural breakdown of cellular components, preventing the microorganism from growing or replicating. Formaldehyde is used in sterilizing medical equipment, while glutaraldehyde is often used as a high-level disinfectant. Advantages: a. Highly effective against bacteria, viruses, fungi, and spores. b. Can be used for disinfecting and sterilizing equipment that cannot withstand heat. Disadvantages: c. Toxic and potentially carcinogenic (e.g., formaldehyde). d. Irritating to the skin, eyes, and respiratory system. e. Requires proper handling and disposal. 42. Be able to describe the use of chemical food preservatives to control microbial growth. Chemical food preservatives are substances added to food to prevent spoilage and extend shelf life by inhibiting microbial growth. Examples: a. Sodium nitrate/nitrite: Prevents the growth of Clostridium botulinum and is used in curing meats. b. Sorbic acid: Used to inhibit mold and yeast growth in baked goods. c. Benzoic acid: Common in soft drinks and fruit juices, effective against bacteria and fungi. d. Calcium propionate: Prevents mold growth in baked goods. How they work: Chemical preservatives either lower the pH of the food, interfere with the metabolism of microorganisms, or disrupt their cell membranes. Some also prevent the microbial enzymes from functioning, thus stopping growth or reproduction. Advantages: e. Effective in controlling microbial growth, thereby increasing shelf life. f. Generally regarded as safe for consumption within regulated limits. Disadvantages: g. Some consumers may be sensitive or allergic to certain preservatives. h. Long-term use of certain preservatives may lead to health concerns (e.g., nitrites being linked to carcinogenic effects in large amounts). 43. Be able to describe how peroxygens are used to control microbial growth. What are they? How do they work? What are advantages/disadvantages of using peroxygens? - What are antimicrobial drugs? Peroxygens are a group of chemical compounds that release oxygen upon breakdown and are used as disinfectants and antiseptics. Examples include hydrogen peroxide and peracetic acid. How they work: Peroxygens release oxygen radicals (O₂⁻), which are highly reactive and can damage microbial DNA, proteins, and lipids, leading to cell death. They are particularly effective against anaerobic bacteria, viruses, and fungi. Advantages: a. Hydrogen peroxide is an effective disinfectant for both surfaces and wounds. b. They are biodegradable and generally considered environmentally friendly. c. Peracetic acid is used as a sterilant in food processing and medical equipment. Disadvantages: d. They can be corrosive to some surfaces and tissues. e. Hydrogen peroxide can be toxic in high concentrations. f. Effectiveness can be reduced in the presence of organic material. Antimicrobial drugs are substances used to kill or inhibit the growth of microorganisms (bacteria, viruses, fungi, and parasites). These drugs include antibiotics, antivirals, antifungals, and antiparasitic drugs. How they work: Antimicrobial drugs can work by targeting specific structures or processes in the microorganism, such as cell wall synthesis, protein synthesis, or DNA replication, disrupting the organism’s ability to grow or reproduce. 44. Be able to describe the 5 modes of action of antimicrobial drugs. The five modes of action of antimicrobial drugs are: Inhibition of cell wall synthesis: Drugs like penicillin prevent bacteria from synthesizing their cell walls, which weakens the cell and causes it to burst due to osmotic pressure. Inhibition of protein synthesis: Drugs like tetracyclines and aminoglycosides bind to bacterial ribosomes and prevent the synthesis of proteins, essential for the microorganism's growth and function. Disruption of cell membrane function: Drugs like polymyxins bind to and disrupt the cell membrane of bacteria, making the membrane leaky and leading to cell death. Inhibition of nucleic acid synthesis: Drugs like rifampin and quinolones block the synthesis of DNA or RNA, inhibiting replication and transcription, which are essential for microbial growth. Inhibition of metabolic pathways: Drugs like sulfonamides block enzymes involved in the synthesis of folic acid, which is necessary for the synthesis of nucleic acids and proteins. 45. Be able to define antibiotic. An antibiotic is a type of antimicrobial drug that is derived from natural sources (such as fungi or bacteria) or synthesized in the lab and is used specifically to treat bacterial infections. Antibiotics work by inhibiting the growth of bacteria or killing them directly. 46. How does antibiotic resistance develop? Antibiotic resistance develops when microorganisms (usually bacteria) evolve mechanisms to resist the effects of drugs that once killed them or inhibited their growth. This resistance can occur through: Mutation: Random mutations in bacterial DNA can result in changes that make the bacteria less susceptible or resistant to an antibiotic. Gene transfer: Bacteria can acquire resistance genes from other bacteria through processes such as conjugation, transduction, or transformation. Selective pressure: Overuse or misuse of antibiotics (such as incomplete courses of treatment or using antibiotics for viral infections) creates selective pressure, allowing resistant bacteria to thrive and multiply. 47. How can bacterial cells pass on antibiotic resistance to other bacterial cells? Bacterial cells can pass on antibiotic resistance through several mechanisms: 1. Conjugation: Direct transfer of DNA, often in the form of plasmids, between two bacteria through a pilus. 2. Transformation: The uptake of free DNA from the environment, which may contain antibiotic resistance genes. 3. Transduction: The transfer of resistance genes from one bacterium to another by a bacteriophage (a virus that infects bacteria). 48. Be able to define the following key terms related to microbial control: sterilization, disinfection, antisepsis, degerming, sanitization, biocide, germicide, bacteriostasis, and asepsis. Sterilization: The complete destruction of all forms of microbial life, including bacterial spores, typically using heat, chemicals, or radiation. Disinfection: The process of eliminating most or all pathogenic microorganisms on a surface or object (not necessarily spores) using chemicals or heat. Antisepsis: The reduction of microbial load on living tissues, usually through the application of antimicrobial agents. Degerming: The removal of microorganisms from a surface, usually through mechanical action (like scrubbing) or the application of antiseptic agents (e.g., alcohol). Sanitization: The reduction of microbial populations to safe levels, typically for public health standards (e.g., in food establishments). Biocide: A broad term for any chemical agent that can kill microorganisms. Germicide: A substance or agent that kills microorganisms. Bacteriostasis: The inhibition of bacterial growth without killing the bacteria, often used to prevent spoilage or infection in non-harmful concentrations. Asepsis: The absence of pathogens or microorganisms, particularly in medical and surgical settings. 49. Be able to describe the patterns of microbial death caused by treatments with microbial control agents. The patterns of microbial death generally follow a logarithmic curve. Initially, the number of viable microorganisms decreases rapidly, and over time, the rate of microbial death slows. This logarithmic death rate suggests that microbial control agents are more effective at higher concentrations or with longer exposure times. The pattern depends on the type of microorganism, the concentration of the agent, and environmental conditions. 50. Be able to describe the effects of microbial control agents on cellular structures. Microbial control agents can affect different cellular structures in various ways, including: Cell wall: Disruption of the cell wall weakens the cell, leading to lysis or inability to regulate its internal environment. Cell membrane: Disruption of the cell membrane causes leakage of essential cellular contents, leading to cell death. Proteins: Denaturation of proteins by heat or chemicals inactivates enzymes and other essential proteins, impairing cellular functions. Nucleic acids: Damage to DNA or RNA prevents replication and transcription, which halts microbial reproduction. 51. Be able to compare the effectiveness of moist heat (boiling, autoclaving, pasteurization) and dry heat. Moist heat and dry heat are both effective methods for controlling microbial growth, but they work differently and have varying effectiveness. Moist Heat: ○ Boiling: Boiling water (100°C) is an effective way to kill most bacteria, viruses, and fungi, but it does not kill spores. Boiling for 10-30 minutes can kill most vegetative forms, but resistant spores (e.g., Clostridium botulinum spores) may survive. ○ Autoclaving: Autoclaving uses steam under pressure at temperatures of 121°C or higher, which is much more effective than boiling or pasteurization. It can kill bacteria, viruses, and spores. The high pressure increases the boiling point of water, allowing for more effective sterilization. Autoclaving is used for sterilizing medical instruments and laboratory equipment. ○ Pasteurization: This involves heating liquids (e.g., milk, juices) to temperatures of 60°C to 85°C for a specific period, followed by rapid cooling. Pasteurization reduces microbial load but does not sterilize. It effectively kills pathogens like Salmonella and Listeria, but spores may survive. There are two main types: HTST (High-Temperature Short Time) and UHT (Ultra-High Temperature). Dry Heat: ○ Dry heat sterilization involves hot air at temperatures ranging from 160°C to 170°C for 2 to 3 hours. It kills microorganisms by oxidizing cellular components, like proteins and lipids, and dehydrating the cell. ○ Dry heat is less efficient than moist heat because it requires higher temperatures and longer exposure times to achieve the same effect. It is commonly used for sterilizing items that cannot tolerate moisture, such as glassware and metal surgical instruments. Comparison: ○ Moist heat is generally more effective than dry heat at lower temperatures because it is more efficient at transferring heat to the microorganisms. Autoclaving is the most effective method for sterilization among the options, as it can kill spores. ○ Dry heat requires higher temperatures and longer exposure times but is effective for sterilizing items that are heat-sensitive to moisture. 52. Be able to describe how filtration, low temperatures, high pressure, desiccation, and osmotic pressure suppress microbial growth. Filtration: Filtration physically removes microorganisms from liquids and air. It works by passing the fluid through a filter with pores small enough to trap microorganisms. It is commonly used to sterilize heat-sensitive liquids, like vaccines, and to purify air using HEPA filters. Low Temperatures: Refrigeration (4°C) and freezing (−20°C) slow down the growth and reproduction of microorganisms by reducing the metabolic rate. While freezing may kill some microorganisms, psychrophiles (cold-loving bacteria) may still grow at low temperatures. Freezing also helps preserve food by preventing spoilage. High Pressure: High-pressure processing (HPP) uses pressures up to 1000 MPa to disrupt cellular structures like membranes and proteins. It can kill microorganisms in food without using high heat, which preserves flavor and nutrients. This method is often used in the food industry for juice pasteurization and meat processing. Desiccation: Drying or desiccation removes water from cells, which inhibits microbial growth because water is essential for metabolic processes. Lyophilization (freeze-drying) is a method often used for preserving microorganisms for research. However, desiccation does not always kill all microbes—some can survive in a dormant state. Osmotic Pressure: By applying high concentrations of solutes (e.g., salt or sugar), osmotic pressure can draw water out of microbial cells, leading to plasmolysis (shrinking of the cell due to loss of water). This prevents the cells from growing or reproducing. This principle is used in food preservation, such as curing meats or preserving fruits in syrup. 53. Be able to explain how radiation kills cells. Radiation kills cells by damaging their DNA through either ionizing or non-ionizing radiation: Ionizing radiation (e.g., X-rays, gamma rays) has enough energy to remove electrons from atoms, forming free radicals that damage DNA and other cellular structures. This leads to mutations, breakage of DNA strands, and ultimately cell death. Ionizing radiation is used for sterilizing medical equipment and food products. Non-ionizing radiation (e.g., UV light) has lower energy and cannot ionize atoms but can excite electrons in molecules. UV radiation damages DNA by causing thymine dimers, which can lead to mutations if not repaired. UV is effective for surface disinfection but cannot penetrate materials or liquids. 54. Be able to interpret the results of use-dilution tests and the disk diffusion method. Use-dilution test: This test evaluates the effectiveness of a disinfectant by exposing metal or glass cylinders to bacterial cultures and then placing them in different concentrations of disinfectants. After a set period, the cylinders are placed in fresh media to see if bacterial growth occurs. A high concentration of disinfectant that prevents growth indicates effectiveness. The results show whether the disinfectant can kill bacteria at the concentration used. Disk diffusion method (also known as the Kirby-Bauer test): In this method, paper disks soaked in different antimicrobial agents are placed on an agar plate inoculated with the target microorganism. The zone of inhibition around each disk indicates the effectiveness of the antimicrobial agent. A larger zone means the agent is more effective against the microorganism. 55. Be able to identify the methods of action and preferred uses of chemical disinfectants. Halogens (e.g., chlorine, iodine): Action: They work by oxidizing microbial proteins and DNA, leading to cell death. Preferred uses: Chlorine is used for water disinfection, and iodine is used for skin antisepsis (e.g., tinctures, betadine). Alcohols (e.g., ethanol, isopropanol): Action: Alcohols denature proteins and dissolve lipids in cell membranes. Preferred uses: Disinfection of skin and medical instruments. They are often used as hand sanitizers. Phenolics (e.g., phenol, bisphenols): Action: They denature proteins and disrupt cell membranes. Preferred uses: Used in hospital disinfectants, as they are effective against bacteria and fungi. Quaternary ammonium compounds (quats): Action: They disrupt cell membranes, leading to leakage of cellular contents. Preferred uses: Used for household cleaning, in hospitals, and in cosmetic products. 56. Be able to differentiate halogens used as antiseptics from halogens used as disinfectants. Antiseptics (e.g., iodine): Used on living tissues to reduce the number of microorganisms. Iodine tinctures are common antiseptics for cleaning wounds. Disinfectants (e.g., chlorine): Used on inanimate surfaces to kill or inhibit microorganisms. Chlorine is often used to disinfect water, swimming pools, and surfaces in food processing. 57. Be able to identify the appropriate uses for surface-active agents. Surface-active agents (surfactants) lower the surface tension of water and help detergents, soaps, and other cleaning products to penetrate surfaces, removing dirt, oils, and microorganisms. Soaps: Used for degerming (removing oils and dirt from the skin). Detergents: Used for cleaning and disinfection (e.g., quaternary ammonium compounds used in hospitals). Emulsifiers: Used to suspend oils and fats in solutions. 58. Be able to list the advantages of glutaraldehyde over other chemical disinfectants. Effectiveness: Glutaraldehyde is a high-level disinfectant and can kill most microorganisms, including bacterial spores, unlike many other disinfectants. Non-corrosive: It is less corrosive compared to other disinfectants like formaldehyde, making it safer to use on medical instruments. Broad-spectrum: It works well against bacteria, fungi, and viruses. Long-lasting: Glutaraldehyde can maintain its effectiveness for extended periods without losing potency. 59. Be able to explain how the type of microbe affects the control of microbial growth. Different types of microorganisms vary in their resistance to microbial control agents: Bacterial spores (e.g., Clostridium, Bacillus) are highly resistant to many disinfectants and require more intense treatments, such as autoclaving or high concentrations of disinfectants. Vegetative bacteria are generally easier to kill but may vary in their resistance depending on the species (e.g., Gram-positive bacteria are often more susceptible than Gram-negative bacteria). Viruses: Enveloped viruses (e.g., influenza, HIV) are more Chapter 8 – Microbial Genetics - What microbial traits are controlled by DNA? Be able to describe. DNA controls almost all microbial traits by encoding the information necessary to produce proteins and RNA molecules. These traits include: Metabolic processes: Enzymes that break down nutrients or synthesize cellular components. Structural components: Proteins that make up cell structures like the cell wall, membrane, and ribosomes. Reproduction: Genes involved in DNA replication and cell division. Virulence factors: Genes coding for toxins or structures (e.g., pili, flagella) that help the microbe infect the host. Antibiotic resistance: Genes that confer resistance to antimicrobial agents. These traits are encoded in the organism’s genome and expressed through the processes of gene expression (transcription and translation). - What is the difference genotype and phenotype? Be able to describe. - Genotype: The genetic makeup of an organism; it refers to the actual sequence of nucleotides in the DNA. The genotype determines the potential for traits to be expressed. - Phenotype: The observable characteristics or traits of an organism, such as its morphology, behavior, or metabolic capabilities. The phenotype results from the expression of the genotype (i.e., the proteins and RNAs produced from the genetic information). In summary, the genotype is the genetic code, while the phenotype is the physical manifestation of that code. - What is the central dogma? What are the steps in the central dogma? Be able to describe. The central dogma of molecular biology describes the flow of genetic information in cells: DNA → RNA → Protein. DNA replication: Copies the DNA, ensuring that genetic information is transmitted to offspring. Transcription: The process by which an RNA molecule is synthesized from a DNA template. This results in messenger RNA (mRNA) that carries the genetic code from DNA to the ribosome. Translation: The process by which the mRNA sequence is used to synthesize a protein at the ribosome, using transfer RNA (tRNA) to match codons in the mRNA with amino acids. Steps: 1. DNA Replication: Makes a copy of the DNA molecule for cell division. 2. Transcription: DNA is transcribed to produce RNA (specifically mRNA). 3. Translation: mRNA is translated into a polypeptide chain (protein). - Be able to describe DNA replication and gene expression (both transcription and translation). DNA Replication: The process by which a cell makes an identical copy of its DNA. It occurs in the S phase of the cell cycle and involves several key enzymes: Helicase unwinds the double helix. Primase synthesizes short RNA primers. DNA polymerase adds new nucleotides to the growing DNA strand. Ligase seals nicks in the DNA backbone. Transcription: The first step of gene expression where a segment of DNA is copied into mRNA. The process involves: RNA polymerase binds to the promoter region of a gene. The enzyme synthesizes a complementary mRNA strand from the DNA template strand. The mRNA is processed (in eukaryotes) to remove introns and join exons together. Translation: The second step of gene expression, where the mRNA is translated into a protein. It occurs at the ribosome and involves: Ribosome attaches to the mRNA. tRNA molecules bring amino acids corresponding to the mRNA codons. Amino acids are linked together to form a polypeptide chain. - Where do replication, transcription, and translation occur in a prokaryotic cell vs in a eukaryotic cell? Prokaryotic Cells (e.g., bacteria): DNA Replication: Occurs in the cytoplasm (since prokaryotes lack a nucleus). Transcription: Occurs in the cytoplasm as well, near the site of DNA. Translation: Occurs simultaneously with transcription in the cytoplasm, where ribosomes are located. Eukaryotic Cells (e.g., plants, animals, fungi): DNA Replication: Occurs in the nucleus during the S phase of the cell cycle. Transcription: Occurs in the nucleus, where mRNA is synthesized and processed (e.g., splicing of introns). Translation: Occurs in the cytoplasm, at the ribosomes (either free in the cytoplasm or on the rough endoplasmic reticulum). - Regarding the flow of genetic information, be able to describe gene expression, recombination, and DNA replication? - Gene Expression: The process by which genetic information is used to synthesize proteins, which ultimately leads to the manifestation of phenotypic traits. This involves transcription (DNA → RNA) and translation (RNA → Protein). - Recombination: The process by which genetic material is rearranged, either during sexual reproduction (e.g., crossing over in meiosis) or in prokaryotes (e.g., horizontal gene transfer via transformation, conjugation, or transduction). Recombination increases genetic diversity. - DNA Replication: The process of copying DNA so that each daughter cell receives an identical copy. This is crucial during cell division (mitosis or binary fission). - - Be able to describe/identify the general structure of nucleotides and the know the location of the 1’, 2’, 3’, 4’, and 5’ carbons of the associated sugar. A nucleotide consists of: A phosphate group (PO₄). A sugar molecule: In DNA, it’s deoxyribose; in RNA, it’s ribose. A nitrogenous base: Either a purine (adenine [A] or guanine [G]) or a pyrimidine (cytosine [C], thymine [T] in DNA, or uracil [U] in RNA). The sugar has five carbon atoms, labeled 1’ through 5’: 1' Carbon: Attached to the nitrogenous base. 2' Carbon: In ribose, this carbon has a hydroxyl group (-OH), while in deoxyribose, it has a hydrogen (H) (which distinguishes DNA from RNA). 3' Carbon: Has a hydroxyl group (-OH) and is involved in forming the phosphodiester bond with the phosphate group of another nucleotide. 4' Carbon: Forms part of the ring structure and is bonded to the 5' carbon. 5' Carbon: Attached to the phosphate group. - What is the bond formed between nucleotides? Know the location of the bond with relation to one nucleotide attaching to another. The bond formed between nucleotides is a phosphodiester bond. This bond occurs between the 3' hydroxyl group (-OH) of the sugar of one nucleotide and the 5' phosphate group of the next nucleotide. This linkage forms the backbone of the nucleic acid (DNA or RNA). Each nucleotide is connected to the next by this bond, creating a sugar-phosphate backbone, with the nitrogenous bases sticking out to form base pairs. - Why are DNA strands antiparallel, and what does antiparallel mean? - DNA strands are antiparallel because the two strands of the double helix run in opposite directions. This means that one strand runs from the 5' to 3' direction, while the complementary strand runs from 3' to 5'. The directionality of the strands is crucial for the proper functioning of DNA replication and transcription because enzymes involved in these processes (like DNA polymerase) can only synthesize DNA in the 5' to 3' direction. - Antiparallel refers to the orientation of the two strands being opposite, with one strand oriented in the 5' to 3' direction and the other in the 3' to 5' direction. This complementary orientation allows the nitrogenous bases to pair correctly and ensures that the DNA can be copied and transcribed efficiently. - Be able to describe the rules of base-pairing (which nucleotide bases bond to one another between two strands of DNA). The base-pairing rules in DNA are: Adenine (A) pairs with Thymine (T) (via two hydrogen bonds). Cytosine (C) pairs with Guanine (G) (via three hydrogen bonds). These specific pairings allow for the double-stranded structure of DNA. The base-pairing ensures that the DNA is a double helix with complementary strands. In RNA, A pairs with Uracil (U) instead of thymine. - Be able to describe how the 5’ end and the 3’ end of a DNA strand are determined. The 5’ and 3’ ends of a DNA strand are determined by the orientation of the sugar molecule (deoxyribose) in each nucleotide. The numbering of the carbons in the sugar molecule defines these ends: The 5' end has a phosphate group attached to the 5' carbon of the sugar. The 3' end has a hydroxyl group (-OH) attached to the 3' carbon of the sugar. DNA strands are always synthesized in the 5' to 3' direction, meaning the strand grows by adding nucleotides to the 3' end. - What is meant by semi-discontinuous DNA replication? Semi-discontinuous DNA replication refers to the fact that one strand of the DNA is replicated continuously, while the other strand is replicated discontinuously in fragments. This happens due to the antiparallel nature of the DNA strands: The leading strand is synthesized continuously in the 5' to 3' direction, following the replication fork as it opens. The lagging strand is synthesized discontinuously in small fragments (Okazaki fragments) because it runs in the 3' to 5' direction, opposite to the direction of the replication fork. These fragments are later joined together by DNA ligase. - During DNA replication, in which direction is the template read? In which direction is the new strand made (synthesized)? During DNA replication: The template strand is read in the 3' to 5' direction. The new strand is synthesized in the 5' to 3' direction. DNA polymerase can only add nucleotides to the 3' end of the growing strand, so it must read the template in the opposite direction (3' to 5'). - What is the replication fork? Be able to describe. The replication fork is the Y-shaped region where the DNA double helix is unwound and separated into two single strands during DNA replication. It is the point at which the two strands of DNA are being separated to serve as templates for the synthesis of new complementary strands. The replication fork consists of several key enzymes: Helicase unwinds the DNA. Single-strand binding proteins (SSBs) prevent the strands from re-annealing. Primase lays down RNA primers to start the synthesis of new DNA strands. At the replication fork, the DNA is being replicated in both directions on the two strands: continuously on the leading strand and discontinuously on the lagging strand. - During replication how are the leading strand and a lagging strand made differently? - The leading strand is synthesized continuously in the 5' to 3' direction, following the direction of the replication fork as it opens. - The lagging strand is synthesized discontinuously in the opposite direction (3' to 5' relative to the fork). Because DNA polymerase can only synthesize in the 5' to 3' direction, small segments called Okazaki fragments are formed on the lagging strand, each starting with a short RNA primer. These fragments are later joined together. - Be able to describe the leading strand and the lagging strands during DNA replication. Leading Strand: The leading strand is synthesized continuously toward the replication fork in the 5' to 3' direction. It is the strand that is in the same direction as the movement of the replication fork, so only one RNA primer is needed to start synthesis. Lagging Strand: The lagging strand is synthesized discontinuously away from the replication fork, in the 3' to 5' direction. It is synthesized in short fragments known as Okazaki fragments, with each fragment requiring a new RNA primer. The RNA primers are later removed, and the fragments are joined together by DNA ligase. - What are Okazaki fragments? Be able to describe. Okazaki fragments are short DNA fragments that are synthesized on the lagging strand during DNA replication. Because the lagging strand runs in the opposite direction to the replication fork, DNA synthesis cannot proceed continuously. Instead, DNA polymerase synthesizes small segments (Okazaki fragments) in the 5' to 3' direction, starting from a newly laid RNA primer. After the fragments are formed, the RNA primers are removed and replaced with DNA, and the fragments are joined together by the enzyme DNA ligase to form a continuous strand. - What are primers? Be able to describe. Why are they necessary? - Primers are short, single-stranded sequences of RNA that are required to initiate DNA replication. They are synthesized by the enzyme primase. Primers are necessary because DNA polymerase, the enzyme that synthesizes new DNA, cannot begin a new strand on its own; it can only add nucleotides to an existing strand. Therefore, a primer provides the free 3' hydroxyl group (–OH) needed for DNA polymerase to begin adding nucleotides. - Primers are eventually removed and replaced with DNA during replication, but they are crucial for the initiation of replication on both the leading and lagging strands. - Be able to describe the events (in order) that happen at the DNA replication fork during DNA replication, including the components (proteins/enzymes) involved. The DNA replication fork is the site where the two DNA strands are unwound and new strands are synthesized. Here’s the sequence of events at the fork: 1. Helicase unwinds the DNA double helix, creating single-stranded regions known as the replication bubble. 2. Single-strand binding proteins (SSBs) bind to the single-stranded DNA to prevent the strands from re-annealing. 3. Topoisomerase (or gyrase) alleviates the torsional strain ahead of the replication fork caused by the unwinding of the DNA. 4. Primase synthesizes short RNA primers to provide a 3' hydroxyl group for DNA polymerase to begin elongation. 5. DNA polymerase III adds new nucleotides to the 3' end of the primer, extending the new DNA strand in the 5' to 3' direction. 6. On the lagging strand, Okazaki fragments are created, each starting with an RNA primer and synthesized discontinuously. 7. DNA polymerase I removes RNA primers and replaces them with DNA. 8. DNA ligase seals the nicks between adjacent Okazaki fragments, creating a continuous strand. This process is continuous on the leading strand and discontinuous on the lagging strand. - Be able to describe the enzymes involved in DNA replication, and be able to describe what each enzyme does during DNA replication. Several key enzymes are involved in DNA replication, each with a specific role: Helicase: Unwinds the double-stranded DNA helix to create single-stranded regions. Single-strand binding proteins (SSBs): Bind to the single-stranded DNA and prevent it from re-annealing. Topoisomerase (Gyrase): Relieves tension and supercoiling ahead of the replication fork caused by the unwinding of the DNA. Primase: Synthesizes short RNA primers that are required to initiate DNA synthesis. DNA polymerase III: Main enzyme that adds nucleotides to the 3' end of the primer, synthesizing the new DNA strand in the 5' to 3' direction. DNA polymerase I: Removes the RNA primers and replaces them with DNA nucleotides. DNA ligase: Joins the sugar-phosphate backbone of adjacent Okazaki fragments on the lagging strand by forming phosphodiester bonds. - What is an origin of replication? Be able to describe. The origin of replication is the specific site on a DNA molecule where replication begins. In both prokaryotes and eukaryotes, replication starts at particular sequences called origins. In prokaryotes, there is typically a single origin of replication, whereas in eukaryotes, there are multiple origins of replication along each chromosome. The origin of replication is recognized by initiator proteins that bind to the origin, causing the DNA to unwind and form the replication bubble. This is where the replication machinery assembles to begin copying the DNA. - What is bidirectional replication? Be able to describe. Bidirectional replication refers to the process where DNA replication occurs in two directions simultaneously from the origin of replication. In this process: Two replication forks are formed at the origin, each moving away from the origin in opposite directions. On both strands, replication occurs in the 5' to 3' direction. On the leading strand, replication is continuous, while on the lagging strand, replication occurs in short fragments (Okazaki fragments). Bidirectional replication ensures that the entire DNA molecule is replicated efficiently and simultaneously from a single origin. - Be able to describe vertical gene transfer and horizontal gene transfer. - Vertical Gene Transfer: This is the transmission of genetic material from parent to offspring during reproduction. This is the typical mode of inheritance in sexually and asexually reproducing organisms. For example, in bacteria, vertical gene transfer occurs when a bacterial cell divides, passing its genetic information to the daughter cells. - Horizontal Gene Transfer: This is the transfer of genetic material between organisms, often of the same generation, without sexual reproduction. It is common in bacteria and can occur via several mechanisms: – Transformation: The uptake of naked DNA from the environment. – Conjugation: The direct transfer of DNA between two bacterial cells through a conjugation pilus. – Transduction: The transfer of genetic material via a virus (bacteriophage). - Be able to describe transformation and the steps involved. Transformation is the process by which bacteria take up foreign DNA from their environment and incorporate it into their own genome. The steps involved in transformation are: 1. DNA release: A donor bacterium dies and releases its DNA into the environment. 2. Uptake of DNA: A recipient bacterium binds and takes up the free DNA from the environment. This typically requires the bacterium to be in a "competent" state, which is a physiological condition that allows DNA uptake. 3. Integration: The foreign DNA integrates into the recipient's genome by recombination. 4. Expression: The recipient bacterium expresses the new genes acquired through transformation. - Be able to describe conjugation and the steps involved. Conjugation is a type of horizontal gene transfer in which genetic material is transferred directly between two bacterial cells through a conjugation pilus. The steps of conjugation are: 1. Formation of conjugation pilus: A donor bacterium with a plasmid (often containing genes like antibiotic resistance genes) extends a conjugation pilus to the recipient bacterium. 2. DNA transfer: The pilus brings the two cells together and creates a direct channel for DNA transfer. Typically, a plasmid is transferred from the donor to the recipient. 3. Plasmid replication: The plasmid is replicated in the donor cell, ensuring that both cells maintain copies of the plasmid. 4. Recipient incorporation: The recipient bacterium may incorporate the transferred plasmid into its own genome or keep it as an extra-chromosomal element. - What is the template for transcription? Be able to describe. The template for transcription is one strand of the DNA, known as the coding strand or non-template strand. During transcription: The RNA polymerase reads the non-coding (template) strand in the 3' to 5' direction to synthesize a complementary RNA strand. The newly synthesized RNA will have the same sequence as the coding strand, except that thymine (T) is replaced by uracil (U) in RNA. - Be able to compare and contrast RNA and DNA polymers. Structure: DNA: A double-stranded molecule composed of deoxyribonucleic acid, with a sugar backbone made of deoxyribose and nucleotides (A, T, C, G). It is stable and stores genetic information. RNA: A single-stranded molecule made of ribonucleic acid, with a sugar backbone made of ribose. It contains the bases A, U (uracil), C, and G. RNA is involved in protein synthesis and gene regulation. Function: DNA: Stores genetic information and is responsible for long-term information storage. RNA: Serves as a template for protein synthesis (mRNA), is involved in the catalysis of biochemical reactions (ribozymes), and plays roles in gene regulation (e.g., miRNA, siRNA). Stability: DNA: Stable, double-stranded, and resistant to degradation. RNA: More unstable due to its single-stranded structure and the presence of the 2'-hydroxyl group, which makes it more prone to hydrolysis. - What is ribosomal RNA (rRNA)? Be able to describe. - Ribosomal RNA (rRNA) is a type of RNA that, along with proteins, makes up the ribosomes, which are the molecular machines responsible for protein synthesis in cells. rRNA plays a crucial role in the structure and function of the ribosome by facilitating the binding of mRNA and tRNA and catalyzing the formation of peptide bonds between amino acids. Ribosomes consist of two subunits: a larger subunit and a smaller subunit, each composed of specific rRNA molecules and proteins. In prokaryotes, rRNA is synthesized in the nucleoid region, while in eukaryotes, it is synthesized in the nucleolus of the nucleus. - What is messenger RNA (mRNA)? Be able to describe. - Messenger RNA (mRNA) is a type of RNA that carries genetic information from the DNA in the nucleus (in eukaryotes) or the cytoplasm (in prokaryotes) to the ribosomes, where protein synthesis occurs. mRNA is synthesized through transcription from a DNA template and serves as a blueprint for building proteins during translation. mRNA is a single-stranded molecule that is complementary to the DNA strand from which it is transcribed. In eukaryotes, mRNA undergoes processing before leaving the nucleus, including splicing (removal of non-coding regions called introns) and the addition of a 5' cap and a poly-A tail. - What is transfer RNA (tRNA)? Be able to describe. Transfer RNA (tRNA) is a type of RNA that plays a key role in protein synthesis by bringing amino acids to the ribosome during translation. Each tRNA molecule has a specific amino acid attached at one end and an anticodon at the other end, which is complementary to the mRNA codon. The anticodon enables the tRNA to recognize and bind to the corresponding codon on the mRNA, ensuring that the correct amino acid is added to the growing polypeptide chain. tRNA acts as an adaptor, matching mRNA codons with their respective amino acids during translation. - What is an RNA polymerase? Be able to describe. RNA polymerase is the enzyme responsible for synthesizing RNA from a DNA template during transcription. It binds to the promoter region of a gene and catalyzes the formation of a complementary RNA strand. RNA polymerase moves along the DNA template strand in the 3' to 5' direction, synthesizing the RNA strand in the 5' to 3' direction. In prokaryotes, RNA polymerase is a single enzyme, whereas in eukaryotes, several forms of RNA polymerase exist, each responsible for transcribing different types of RNA (e.g., mRNA, rRNA, and tRNA). - What is the promoter? Be able to describe. The promoter is a specific sequence of DNA located near the beginning of a gene. It acts as the binding site for RNA polymerase and other transcription factors that are necessary to initiate transcription. The promoter is typically located upstream (before) the coding region of the gene and defines the direction of transcription. In prokaryotes, the promoter usually contains consensus sequences such as the -35 and -10 regions (named for their approximate distance from the transcription start site), while in eukaryotes, the promoter often contains the TATA box, a similar conserved sequence. - What is the terminator? Be able to describe. - The terminator is a specific DNA sequence that signals the end of transcription. Once RNA polymerase reaches the terminator sequence, it stops synthesizing RNA and detaches from the DNA template. In prokaryotes, terminators can be intrinsic (self-terminating) or involve the assistance of a protein called Rho. In eukaryotes, termination is more complex and often involves the cleaving of the newly synthesized RNA transcript and the addition of a poly-A tail. - What are the three stages of transcription? What occurs during each stage? The three stages of transcription are: 1. Initiation: ○ RNA polymerase binds to the promoter region of the gene. ○ Transcription factors help RNA polymerase locate the promoter (in eukaryotes). ○ The DNA strands are unwound, and the RNA polymerase begins synthesizing RNA at the +1 transcription start site. 2. Elongation: ○ RNA polymerase moves along the DNA template strand in the 3' to 5' direction. ○ It synthesizes a complementary RNA strand in the 5' to 3' direction. ○ As RNA polymerase moves along, the DNA is unwound ahead of it, and the RNA is elongated by adding nucleotides. 3. Termination: ○ RNA polymerase reaches the terminator sequence and detaches from the DNA. ○ In prokaryotes, this may involve a hairpin loop or the action of the Rho protein. ○ In eukaryotes, termination often involves the cleavage of the RNA and the addition of a poly-A tail. - During transcription, in which direction is the DNA template read? In which direction is the RNA strand made (synthesized)? During transcription: The DNA template strand is read by RNA polymerase in the 3' to 5' direction. The RNA strand is synthesized in the 5' to 3' direction. This is because RNA polymerase adds nucleotides to the growing RNA chain at the 3' end. - What is RNA processing, and why is it done in eukaryotes? RNA processing refers to the modifications made to the primary RNA transcript (pre-mRNA) before it is transported out of the nucleus in eukaryotic cells. These modifications include: 5' capping: A 7-methylguanosine cap is added to the 5' end of the mRNA. This cap protects the mRNA from degradation, helps with ribosome binding during translation, and facilitates mRNA export from the nucleus. Splicing: Non-coding regions called introns are removed, and the remaining exons are joined together to form a continuous coding sequence. Polyadenylation: A poly-A tail is added to the 3' end of the mRNA, which aids in stability, transport, and translation initiation. RNA processing is necessary in eukaryotes because they have introns in their genes, and mRNA must be properly modified before it can be translated into a protein. Prokaryotes do not have introns and typically do not require such extensive RNA processing. - What are exons? Be able to describe. Exons are the coding regions of a gene that are transcribed into mRNA and eventually expressed as protein. Exons are spliced together after the removal of non-coding regions called introns during RNA processing in eukaryotes. Exons contain the information that will be used to synthesize the protein in the process of translation. In a mature eukaryotic mRNA molecule, the exons are connected by a continuous coding sequence after introns have been excised. - What are introns? Be able to describe. - Introns are non-coding regions of a gene that are transcribed into RNA but are not used for protein synthesis. They are removed from the pre-mRNA during RNA splicing before the mRNA is translated into a protein. In eukaryotes, genes typically consist of both exons (coding regions) and introns (non-coding regions). Introns are removed through a process called splicing, and the exons are joined together to form the mature mRNA that will be translated into protein. - Introns contribute to the complexity of gene expression regulation and can influence mRNA stability and alternative splicing, which allows one gene to code for multiple protein isoforms. - What is the 5’ cap and 3’ poly A tail? Be able to describe. The 5’ cap and 3’ poly-A tail are modifications made to eukaryotic mRNA during RNA processing to protect the mRNA and assist in its translation. · 5’ Cap: A modified guanine nucleotide (7-methylguanosine) is added to the 5' end of the mRNA. This cap protects the mRNA from degradation, helps with mRNA stability, facilitates the export of the mRNA from the nucleus, and aids in the binding of the mRNA to the ribosome for translation. · 3’ Poly-A Tail: A string of adenine nucleotides (about 100-250 A’s) is added to the 3' end of the mRNA. This tail helps stabilize the mRNA, aids in the export of mRNA from the nucleus, and plays a role in translation initiation. It also contributes to the mRNA's longevity in the cytoplasm. - What are amino acids, and what is the generalized structure of amino acids? Be able to describe. Amino acids are the building blocks of proteins. Each amino acid consists of: 1. A central carbon atom (α-carbon). 2. A hydrogen atom (attached to the α-carbon). 3. A carboxyl group (–COOH): This is the functional group that makes the molecule acidic. 4. An amino group (–NH₂): This is the functional group that makes the molecule basic. 5. A variable side chain (R group): This is what distinguishes different amino acids. The side chain can be simple (e.g., hydrogen) or complex (e.g., a ring structure). The R group determines the chemical properties of the amino acid (e.g., polar, non-polar, acidic, basic). - What type of bond forms between amino acids? Be able to describe. The bond that forms between amino acids is called a peptide bond. It is a covalent bond formed between the carboxyl group of one amino acid and the amino group of another amino acid. During this process, a molecule of water (H₂O) is released in a dehydration synthesis reaction (also known as a condensation reaction). This bond links the amino acids together into a polypeptide chain, which eventually folds into a functional protein. - What are codons? What is their purpose? Where do we find codons? - Codons are sequences of three nucleotides in mRNA that code for a specific amino acid during protein synthesis. Each codon corresponds to one of the 20 amino acids or to a stop signal that ends protein synthesis. Codons are found in mRNA during the process of translation. - The purpose of codons is to provide the genetic instructions for assembling a sequence of amino acids to form a protein. The sequence of codons in mRNA is translated into an amino acid sequence by ribosomes and tRNA during protein synthesis. - Be able to describe sense codons and nonsense codons. - Sense Codons: These are codons that code for specific amino acids. There are 61 sense codons, each corresponding to an amino acid. - Nonsense Codons: These are codons that do not code for any amino acid and instead signal the termination of translation. There are 3 nonsense codons (stop codons): UAA, UAG, and UGA. These codons are used to signal the end of the protein synthesis process. - How many possible codons are there? There are 64 possible codons in the genetic code. This is because there are four nucleotide bases (A, U, C, G), and codons are made up of three nucleotide bases, so the number of possible combinations is 4³ = 64. Out of these 64 codons: 61 codons specify amino acids. 3 codons are stop codons (nonsense codons). - Why do some codons “code” for the same amino acids as other codons? - The redundancy of the genetic code, where multiple codons code for the same amino acid, is known as degeneracy. This redundancy occurs because the third base of a codon (the "wobble" position) can often vary without changing the amino acid it codes for. For example, the codons GCU, GCC, GCA, and GCG all code for the same amino acid, alanine. This redundancy helps minimize the effects of mutations, as a change in the third position may still code for the same amino acid, thus preventing any change to the protein sequence. - What are the 3 nonsense codons? The three nonsense codons (also known as stop codons) are: UAA UAG UGA These codons signal the end of translation, causing the ribosome to detach from the mRNA and release the newly synthesized polypeptide chain. - What is the start codon and what amino acid does it encode for? The start codon is AUG, which is the first codon of mRNA that is recognized by the ribosome to begin translation. AUGcodes for the amino acid methionine in eukaryotes (and formylmethionine in prokaryotes). Methionine is always the first amino acid in a newly synthesized protein, although it may be removed later in the process. - Be able to interpret the codon table (tell which amino acid a given codon “codes” for when using the table) The codon table maps each of the 64 codons to their corresponding amino acid. For example: The codon AUG codes for methionine. The codon UUU codes for phenylalanine. The codon UAA, UAG, and UGA are stop codons. To interpret a codon from the table, match the first base of the codon to the left-most column, the second base to the top row, and the third base to the right-most column. This will give you the corresponding amino acid. - What are ribosomes? What role do ribosomes play during the translation step of gene expression? - Ribosomes are large molecular machines composed of rRNA and proteins that facilitate protein synthesis. Ribosomes consist of two subunits: the large subunit and the small subunit. They are found in the cytoplasm (in both prokaryotes and eukaryotes) and are essential for translating mRNA into a polypeptide chain. - During translation, the ribosome reads the mRNA codons and matches them with the appropriate tRNA molecules that carry the corresponding amino acids. As the ribosome moves along the mRNA, it catalyzes the formation of peptide bonds between amino acids, elongating the polypeptide chain. - What are anticodons? Be able to describe. Anticodons are sequences of three nucleotides found on tRNA molecules. The anticodon is complementary to the codon on the mRNA. Each tRNA molecule has an anticodon that recognizes and pairs with its corresponding mRNA codon during translation. The purpose of the anticodon is to ensure that the correct amino acid is added to the growing polypeptide chain by matching with the correct codon in the mRNA sequence. - In which direction is the mRNA template read during translation? Be able to describe. During translation, the mRNA template is read in the 5' to 3' direction. The ribosome moves along the mRNA strand from the 5' end towards the 3' end. Each codon, which consists of three nucleotides, is read sequentially by the ribosome. The corresponding tRNA molecules bring the correct amino acids, which are then added to the growing polypeptide chain. This process continues until a stop codon is reached. - What is the A, E, and P site on a ribosome? Be able to describe. The ribosome has three important sites where tRNA molecules interact during translation: · A site (Aminoacyl site): This is the site where a tRNA carrying an amino acid binds to the mRNA codon. The A site is the first stop for each incoming tRNA molecule, where the correct amino acid is added to the growing polypeptide chain. · P site (Peptidyl site): This is the site where the tRNA carrying the growing polypeptide chain is located. The P site holds the tRNA that has the polypeptide attached, and it is where the peptide bond is formed between the amino acid from the A site and the growing polypeptide chain. · E site (Exit site): This is the site where the empty tRNA (having already delivered its amino acid) exits the ribosome after the peptide bond has been formed. Once the tRNA has released its amino acid, it moves to the E site before leaving the ribosome. - Be able to describe (in order) the steps in translation and the components involved. Don’t forget things like the E, P, and A site. The steps of translation occur in three main phases: initiation, elongation, and termination. Initiation: 1. The small ribosomal subunit binds to the mRNA at the start codon (AUG). 2. The initiator tRNA (carrying methionine in eukaryotes) binds to the start codon in the P site. 3. The large ribosomal subunit binds to the small subunit, forming the functional ribosome. Now the A, P, and E sites are fully formed. Elongation: 1. A tRNA carrying the appropriate amino acid enters the A site and binds to the next mRNA codon. 2. A peptide bond is formed between the amino acid in the A site and the polypeptide chain in the P site. 3. The ribosome moves along the mRNA, a process called translocation. The tRNA in the P site moves to the E site, where it exits, and the tRNA in the A site moves to the P site. 4. A new tRNA enters the A site and the process repeats. Termination: 1. The ribosome reaches a stop codon (UAA, UAG, or UGA) on the mRNA. 2. The release factor binds to the stop codon in the A site, which causes the ribosome to release the newly synthesized polypeptide chain. 3. The ribosomal subunits dissociate from the mRNA, and the translation process ends. Components involved: mRNA: Provides the genetic code to be translated into a protein. tRNA: Brings amino acids to the ribosome based on codon-anticodon matching. Ribosomes: Composed of rRNA and proteins, facilitate translation by binding mRNA and tRNA. Amino acids: The building blocks of proteins, brought to the ribosome by tRNA. - What is meant by the coupling of transcription and translation? Be able to describe. Coupling of transcription and translation occurs primarily in prokaryotic cells, where both processes happen in the cytoplasm and can occur simultaneously. As the mRNA is being transcribed from DNA by RNA polymerase, the ribosomes begin translating the mRNA into protein. This coupling is efficient because there is no nuclear membrane to separate transcription from translation in prokaryotes. In eukaryotes, transcription occurs in the nucleus, and translation occurs in the cytoplasm, so the processes are not coupled. - Be able to define mutation, mutagens, and spontaneous mutation. - Mutation: A mutation is a permanent change in the DNA sequence of an organism's genome. Mutations can lead to changes in the structure and function of proteins. - Mutagens: Mutagens are physical or chemical agents that increase the rate of mutation. Examples include radiation (X-rays, UV light) and chemicals (such as those in tobacco smoke). - Spontaneous Mutation: A spontaneous mutation is a mutation that occurs naturally without external influences. These mutations can result from errors during DNA replication or natural chemical changes in the DNA. - What are base substitution mutations? Be able to describe. Base substitution mutations (also known as point mutations) involve the replacement of one nucleotide with another in the DNA sequence. There are three types: Silent Mutation: The change in the base does not affect the amino acid sequence (due to the redundancy of the genetic code). Missense Mutation: The change results in a different amino acid being incorporated into the protein, potentially altering its function. Nonsense Mutation: The change results in a stop codon, causing premature termination of the protein, which often results in a nonfunctional protein. - What are missense and nonsense mutations? Be able to describe. - Missense Mutation: This type of mutation results in the substitution of one amino acid for another in the protein sequence. This may or may not affect the protein's function, depending on the location and properties of the new amino acid. - Nonsense Mutation: A nonsense mutation changes a codon that codes for an amino acid into a stop codon. This causes premature termination of the polypeptide chain, often resulting in a nonfunctional protein. - What are frameshift mutations? Be able to describe. Frameshift mutations occur when nucleotides are inserted or deleted in a number that is not a multiple of three, disrupting the reading frame of the codons. This causes a shift in how the sequence of codons is read, leading to an incorrect sequence of amino acids in the resulting protein. Frameshift mutations often result in completely nonfunctional proteins. - What is a benefit of mutations? - Mutations are a source of genetic variation, which is essential for evolution. Beneficial mutations can give an organism an advantage in its environment, such as increased resistance to a disease, better adaptability to environmental changes, or enhanced survival traits. These beneficial mutations may be passed on to future generations, contributing to the species' evolutionary development. - Be able to define genetics, genome, chromosome, gene, genetic code, genotype, phenotype, and genomics. - Genetics: The study of genes, inheritance, and genetic variation in organisms. - Genome: The complete set of genetic material (DNA) in an organism, including all its genes. - Chromosome: A structure made of DNA and proteins that contains many genes. Organisms have a specific number of chromosomes in their cells (e.g., humans have 46). - Gene: A segment of DNA that contains the instructions for making a specific protein or RNA molecule. - Genetic Code: The set of rules by which information encoded in mRNA is translated into proteins. It is based on codons, where each three-nucleotide codon specifies an amino acid or a stop signal. - Genotype: The genetic makeup of an organism, specifically the alleles it carries. - Phenotype: The observable characteristics or traits of an organism, which result from the interaction between its genotype and the environment. - Genomics: The study of an organism's genome, including the sequencing, analysis, and comparison of its complete DNA sequence. - Be able to describe how DNA serves as genetic information. DNA (Deoxyribonucleic acid) serves as genetic information by encoding the instructions necessary for the development, functioning, and reproduction of all living organisms. The sequence of nucleotides (adenine, thymine, cytosine, and guanine) in DNA forms genes, which are functional units that code for proteins. Each gene contains specific instructions for the synthesis of a particular protein, which in turn determines the structure and function of cells, tissues, and organs. The flow of genetic information follows the central dogma of molecular biology: DNA → RNA → Protein. DNA replication ensures the genetic information is passed on to offspring during cell division. - Describe protein synthesis, including transcription and translation. Protein synthesis consists of two main stages: transcription and translation. Transcription (DNA → mRNA): 1. Initiation: RNA polymerase binds to a specific region of the gene called the promoter on the DNA template strand. 2. Elongation: RNA polymerase synthesizes a complementary strand of mRNA by reading the DNA template strand in the 3’ to 5’ direction and adding nucleotides to the growing RNA chain in the 5’ to 3’ direction. 3. Termination: When RNA polymerase reaches a terminator sequence, transcription stops, and the mRNA is released. Translation (mRNA → Protein): 1. Initiation: The small ribosomal subunit binds to the mRNA at the start codon (AUG). The corresponding tRNA carrying methionine binds to the start codon in the P site of the ribosome. 2. Elongation: The ribosome moves along the mRNA, reading the codons, and tRNA molecules bring the corresponding amino acids to the ribosome. Amino acids are linked together by peptide bonds to form a polypeptide. 3. Termination: The ribosome reaches a stop codon (UAA, UAG, UGA), and the newly synthesized protein is released. - Compare protein synthesis in prokaryotes and eukaryotes. Prokaryotes: Transcription and translation occur simultaneously because both processes happen in the cytoplasm (no nuclear membrane). mRNA is typically not processed (no 5' cap, no poly-A tail). No introns: Prokaryotic genes do not have introns, so transcription directly produces mature mRNA. Eukaryotes: Transcription occurs in the nucleus, and translation occurs in the cytoplasm. mRNA processing includes adding a 5' cap, a poly-A tail, and splicing out introns before the mRNA is transported to the cytoplasm. Introns and exons are present: Genes often contain introns (non-coding regions) and exons (coding regions). - What are operons? Be able to describe. Operons are a cluster of functionally related genes found in prokaryotic cells. An operon consists of: A promoter: The region where RNA polymerase binds to initiate transcription. An operator: A region that acts as a regulatory switch, controlling the transcription of the operon. Structural genes: The genes that code for proteins involved in a particular metabolic pathway or function. An activator or repressor protein: A regulatory protein that either enhances or inhibits the binding of RNA polymerase to the promoter. Example: The lac operon in E. coli controls the metabolism of lactose. It is regulated by a repressor protein that binds to the operator, preventing transcription when lactose is not present. - Be able to describe pre-transcriptional regulation of gene expression in bacteria. Pre-transcriptional regulation refers to mechanisms that control the transcription of genes before mRNA is synthesized. In bacteria, this includes: Repressors: Proteins that bind to the operator region of an operon, blocking RNA polymerase from transcribing the genes. Inducers: Molecules that bind to repressor proteins and inactivate them, allowing RNA polymerase to transcribe the operon. Activator proteins: Enhance the binding of RNA polymerase to the promoter to increase transcription. Catabolite repression: In E. coli, the presence of glucose inhibits the transcription of genes involved in the metabolism of other sugars, such as lactose. - Be able to describe post-transcriptional regulation of gene expression. Post-transcriptional regulation refers to mechanisms that control gene expression after mRNA has been transcribed. These include: RNA splicing: In eukaryotes, introns are removed, and exons are joined together to produce a mature mRNA. Alternative splicing: Different combinations of exons can be spliced together, producing multiple protein variants from a single gene. RNA stability: The stability of the mRNA molecule can be regulated, influencing how long it remains in the cell before being degraded. RNA interference (RNAi): Small RNA molecules (like miRNA and siRNA) can bind to mRNA molecules, leading to their degradation or preventing translation. - Be able to classify mutations by type. Mutations can be classified into several types based on their effects on the DNA sequence: Point mutations: ○ Substitutions: One nucleotide is replaced by another (e.g., A → G). Silent mutation: No change in amino acid. Missense mutation: A different amino acid is incorporated. Nonsense mutation: A stop codon is introduced. Frameshift mutations: Insertion or deletion of nucleotides that alters the reading frame. Insertion mutations: Addition of nucleotides into the DNA sequence. Deletion mutations: Removal of nucleotides from the DNA sequence. - Be able to describe two ways mutations can be repaired. DNA Repair Mechanisms: Mismatch repair: Repairs errors in DNA replication. The repair enzymes recognize the mismatch and remove the incorrect base, replacing it with the correct one. Nucleotide excision repair: Removes damaged DNA, such as thymine dimers caused by UV light. The damaged segment is cut out, and the correct nucleotides are synthesized and inserted. - Be able to describe the effect of mutagens on the mutation rate. Mutagens are physical or chemical agents that increase the mutation rate. They cause changes in the DNA sequence by inducing errors during replication or causing direct damage to the DNA. Examples of mutagens include: Physical mutagens: UV radiation, X-rays, and gamma rays, which can cause strand breaks, base modifications, or cross-linking of bases. Chemical mutagens: Chemicals like base analogs (which mimic bases and cause mispairing) or alkylating agents (which add alkyl groups to bases, causing mispairing) can alter the structure of DNA and lead to mutations. Mutagens increase the mutation rate by increasing the likelihood of errors during DNA replication or repair. - Be able to describe the functions of plasmids and transposons. - Plasmids: Small, circular pieces of DNA that are separate from the chromosomal DNA in prokaryotic cells. Plasmids can carry genes for functions such as antibiotic resistance, virulence factors, or metabolic capabilities. Plasmids can replicate independently and be transferred between cells through conjugation, transformation, or transduction. - Transposons: Also known as jumping genes, transposons are DNA sequences that can move from one location in the genome to another. They contain sequences that allow them to "cut and paste" or "copy and paste" themselves within the genome. Transposons can disrupt genes or cause mutations when they insert themselves into coding regions. Chapter 9 – Genetic Engineering - Be able to describe the following terms: biotechnology, genetic modification, and recombinant DNA technology. - Biotechnology: Biotechnology refers to the use of living organisms, cells, or biological systems to develop or create new products and processes for specific applications, such as in medicine, agriculture, and industry. It includes both traditional techniques (like fermentation) and modern methods (such as genetic engineering and recombinant DNA technology). - Genetic Modification: Genetic modification (or genetic engineering) involves the deliberate alteration of an organism's genetic material to achieve desired traits. This can involve adding, deleting, or modifying specific genes within an organism’s genome. Genetic modification can be used in agriculture (e.g., genetically modified crops), medicine (e.g., producing insulin), and research. - Recombinant DNA Technology: Recombinant DNA (rDNA) technology is a technique used to manipulate an organism’s DNA by combining DNA from different sources. This involves inserting foreign genes (such as human genes) into an organism’s DNA to produce new proteins or modify traits. The resulting recombinant DNA can be introduced into a host organism (e.g., bacteria, yeast, or mammalian cells) for protein production or further study. - Be able to describe the roles of a clone and a vector in making recombinant DNA. - · Clone: A clone is a genetically identical copy of a DNA molecule, cell, or organism. In recombinant DNA technology, a clone typically refers to a cell or organism that contains a specific piece of foreign DNA integrated into its genome. The goal of cloning is to propagate a gene of interest in a host cell (like a bacterium) to produce many copies of the gene or its protein product. - · Vector: A vector is a DNA molecule used to carry and introduce foreign DNA into a host cell. Vectors typically carry the gene of interest, along with other features that help with the replication, maintenance, or selection of recombinant DNA inside the host cell. Common vectors include plasmids, bacteriophages, and viral vectors. Vectors ensure the stable transfer and expression of foreign DNA. - Be able to describe restriction enzymes, and how they are used to make recombinant DNA. - · Restriction Enzymes: Restriction enzymes (also known as restriction endonucleases) are enzymes that cut DNA molecules at specific sequences of nucleotides, known as restriction sites. These enzymes serve as molecular scissors that allow scientists to cut DNA into smaller fragments. The cuts can produce "sticky ends" or "blunt ends," which are useful for recombination. - · Use in Recombinant DNA: Restriction enzymes are used in recombinant DNA technology to cut both the vector DNA (such as a plasmid) and the foreign DNA (such as a gene of interest) at specific sites. The sticky or blunt ends of the cut DNA can then be joined together using another enzyme called ligase to form recombinant DNA molecules, which can be inserted into host cells for replication or protein production. - Be able to describe the four common properties (components) of vectors. The four common properties of vectors used in recombinant DNA technology are: · Origin of replication (ORI): This is a sequence of DNA that allows the vector to replicate independently within a host cell, ensuring that the recombinant DNA is copied as t