Lecture 3: Microbial Evolution and Diversity PDF
Document Details
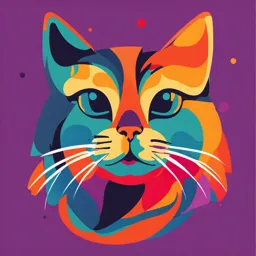
Uploaded by PreferableVampire3994
Tags
Summary
This lecture covers microbial evolution and diversity, discussing topics like cyanobacteria, anaerobes, and aerobes. The notes explore heterotrophs, autotrophs, and the role of oxygen in cellular processes. Furthermore, the document touches upon viruses, and delves into their impact. The content provides a basis for understanding of bacterial life and evolution.
Full Transcript
Lecture 3: Microbial Evolution and Diversity Modern Cyanobacteria: Thylakoids: Extensive intracellular membrane systems (in chloroplast) hosting pigments (chlorophyll) and electron transport components for photosynthesis...
Lecture 3: Microbial Evolution and Diversity Modern Cyanobacteria: Thylakoids: Extensive intracellular membrane systems (in chloroplast) hosting pigments (chlorophyll) and electron transport components for photosynthesis (convert light energy into chemical energy) Arranged in stacks called grana. Important: main site of light absorption in photosynthesis Producing ATP & NADPH Toxins: Some produce harmful or pharmacologically promising toxins. Prokaryotes Defined: Lecture 3: Microbial Evolution and Diversity 1 Single-celled organisms lacking a nucleus, typically smaller and structurally simpler than eukaryotic cells. Anaerobes: 2nd characteristics of earliest cell types Grow without oxygen and don’t use oxygen in metabolism. Photosynthetic & non-Photosynthetic are anaerobic (bacterial photosynthesis is an anaerobic process) Types of Anaerobes: Obligate Anaerobes: Cannot grow in presence of oxygen. O2 is lethal. (e.g., Human pathogen; Clostridium botulinum). Facultative Anaerobes: Prefer oxygen (grows aerobically) because more ATP is produced, but can grow anaerobically without O2 (e.g., E. coli). Aerotolerant Anaerobes: Grow equally well with or without oxygen (e.g., Streptococcus pyogenes - strep throat, flesh-eating disease). Aerobes: Require oxygen for metabolism. Generate ATP using O2 Microaerophiles: Require lower oxygen levels (2–10%) than atmospheric oxygen (20%) [e.g., Helicobacter pylori - gastric & duodenal ulcers] & [e.g. Treponema pallidum - causative agent of STD]. How Aerobes Use Oxygen (Aerobic Respiration) Aerobes generate energy through aerobic respiration, which occurs in three major steps: 1. Glycolysis (Cytoplasm) Glucose is broken down into pyruvate, producing 2 ATP molecules. 2. Krebs Cycle (Mitochondria) Pyruvate enters the mitochondria and is converted into energy-rich molecules NADH and FADH₂. Lecture 3: Microbial Evolution and Diversity 2 3. Electron Transport Chain (ETC) (Mitochondria) Oxygen acts as the final electron acceptor, allowing the production of up to 34 ATP molecules. End Products: ATP (energy), CO₂ (carbon dioxide), and H₂O (water) Aerobes vs. Anaerobes Feature Aerobes Anaerobes Oxygen Yes No requirement Energy efficiency High (36-38 ATP) Low (2 ATP) Humans, bacteria Example organisms Clostridium, Bacteroides like Mycobacterium Can be toxic to some Toxicity of oxygen Not toxic anaerobes Evolutionary Background of Anaerobes and Aerobes: Anaerobes and microaerophiles evolved before aerobes when Earth transitioned from an anoxic to an oxic environment. They lack all or some enzymes that allow them to live in an oxygenated environment Using Oxygen generates toxic by-products. Aerobes evolved detoxifying enzymes like SOD and catalase. Heterotrophs and Autotrophs: Third characteristics of earliest cell type Heterotrophs: Require organic carbon sources (e.g., glucose). Hetero = different: trophs = nourishment Heterotrophs cannot use CO2 as a major carbon source for the biosynthesis of cellular constituents [nucleotides, amino acids, fatty acids] Lecture 3: Microbial Evolution and Diversity 3 Photoheterotrophs (light + organic carbon): Use sunlight for energy (e.g., purple non-sulfur bacteria). Chemoheterotrophs: Use organic compounds for energy (e.g., fungi, animals). Most common group : associated with humans & other animals Use organic compounds [ sugars, amino acids] for energy source Use organic compounds as a source of carbon Autotrophs: Use CO2 as a carbon source (not direct). Auto = self ; troph = nourishment Photoautotrophsm: Use sunlight as energy source (e.g., cyanobacteria, purple, sulphur bacteria, green sulphur). Chemoautotrophs (Chemolithotrophs): Use inorganic compounds as energy (e.g., sulfur-oxidizing bacteria). Modern examples of autotrophs = [carbon dioxide as carbon source, usually light as energy source] green plants ; eukaryotic algae ; cyanobacteria ; photosynthetic bacteria (different from Cyanobacteria). Chemolithotrophs: certain types of non-photosynthetic autotrophic bacteria [i.e. Chemical rock eaters, Carbon dioxide as carbon source] only found in prokaryotes widely distributed in eubacteris & archae except for autotrophs listed above all other organisms on this planet are heterotrophs Heterotrophs vs. Autotrophs Feature Heterotrophs Autotrophs Carbon source Organic molecules CO₂ Lecture 3: Microbial Evolution and Diversity 4 Light (photoautotrophs) or Light (photoheterotrophs) or organic Energy source inorganic chemicals compounds (chemoheterotrophs) (chemoautotrophs) Humans, animals, fungi, most Examples Plants, algae, cyanobacteria bacteria What Are Chemolithotrophs? Chemolithotrophs = Chemical rock eaters prokaryotes (bacteria and archaea); get energy from inorganic compounds. Use CO₂ as their carbon source, just like plants, but they do not use sunlight for energy. Also called chemoautotrophs because they make their own organic molecules from CO₂ using chemical energy. How Do Chemolithotrophs Obtain Energy? Instead of light (photosynthesis), they use inorganic compounds for energy. Each type of chemolithotroph specializes in one kind of inorganic material, such as: H₂ (hydrogen gas) H₂S (hydrogen sulfide) Fe²⁺ (ferrous iron) NH₄⁺ (ammonium) perform oxidation reactions to extract energy, similar to how humans burn food for energy, but using rocks and minerals instead. Evolution & Adaptation Chemolithotrophs likely evolved from cyanobacteria, which originally performed photosynthesis. After the Earth's atmosphere became oxic (oxygen-rich), some cyanobacteria lost their photosynthetic ability and adapted to using inorganic chemicals Lecture 3: Microbial Evolution and Diversity 5 instead. Today, they thrive in extreme environments, such as: Sulfur hot springs (rich in H₂S). Deep-sea hydrothermal vents (rich in iron and sulfur). Caves and underground ecosystems with mineral deposits. Types of Chemolithotrophs & Their Roles Energy Source Type of Example (Inorganic Process Chemolithotroph Organisms Compound) H₂S (hydrogen Sulfur-Oxidizing Oxidizes H₂S to Thiobacillus, sulfide) or metal Bacteria SO₄²⁻ (sulfate) Beggiatoa sulfides Nitrogen-Oxidizing Converts NH₃ → NH₃ (ammonia) or Nitrosomonas, Bacteria (Nitrifying NO₂⁻ (nitrite) → NO₃⁻ NO₂⁻ (nitrite) Nitrobacter Bacteria) (nitrate) Iron-Oxidizing Acidithiobacillus Fe²⁺ (ferrous iron) Converts Fe²⁺ → Fe³⁺ Bacteria ferrooxidans Hypothesis of First Organisms: Likely prokaryotic, anaerobic, and heterotrophic. Simple likely evolved from complex. [eukaryotic cell structure relatively complex, prokaryotic relatively simple] The first organisms were likely anaerobic Evolved when Earth’s environment lacked oxygen and had reducing conditions. Heterotrophs likely gave rise to autotrophs due to selective pressure from nutrient depletion. 1. Primordial Soup Formation→ Organic nutrients accumulate by abiogenic synthesis 2. Heterotrophs Emerge → Early life forms consume these nutrients. Lecture 3: Microbial Evolution and Diversity 6 3. Nutrient Depletion → Consumption outpaces natural production. 4. Selective Pressure → Cells struggle to survive as key nutrients become short. 5. Autotrophy Evolves → Some cells develop biosynthetic pathways, gaining an advantage and eventually leading to autotrophic life Biosynthetic Pathway Evolution: Nutrient depletion led to selective pressure for cells that could synthesize essential nutrients, giving rise to autotrophs. Autotrophs restored organic substances to the biosphere, supporting heterotroph survival. Cyanobacterial Photosynthesis Cyanobacteria are aerobic, photosynthetic autotrophs that use light energy to convert CO₂ and H₂O into carbohydrates and release O₂ as a byproduct. This process is known as oxygenic photosynthesis and is different from the anaerobic photosynthesis used by earlier bacteria. Chemical Reaction for Cyanobacterial Photosynthesis: CO_2 + H_2O → CHO (Carbohydrates) + O_2 (Free Oxygen) ↑(release) CO₂ is fixed into carbohydrates (energy storage). H₂O donates electrons (instead of H₂S, as in anaerobic photosynthesis). O₂ is released as a byproduct. Evolutionary Origins of Cyanobacteria Cyanobacteria are believed to have evolved from anaerobic photosynthetic bacteria that used H₂S instead of H₂O to fix CO₂. Over time, these bacteria developed the ability to use water (H₂O) as an electron donor, producing oxygen (O₂) as a byproduct instead of sulfur. Lecture 3: Microbial Evolution and Diversity 7 First cyanobacteria → then eukaryotic algae → then higher plants followed the same oxygenic photosynthesis process. The Role of Cyanobacteria in Earth's Oxygenation Before cyanobacteria evolved, Earth's atmosphere was anoxic (lacking oxygen) and reducing (rich in molecules like methane and hydrogen). Cyanobacterial photosynthesis gradually increased oxygen levels, leading to oxidation of previously reduced substances in the environment. This changed Earth’s atmosphere from a reducing to an oxidizing atmosphere, marking a major shift in planetary conditions. The Reactivity of Oxygen and Ozone Formation Oxygen (O₂) is highly reactive and readily forms chemical compounds by oxidizing other molecules. In the presence of UV light, some oxygen (O₂) molecules reacted to form ozone (O₃), which created the ozone layer. The ozone layer protected life from harmful UV radiation, enabling more complex life forms to evolve. The Great Oxygenation Event (GOE) Timeline: Around 2.4 billion years ago, cyanobacteria caused a major rise in atmospheric oxygen.\n- Effects: Oxygen reacted with iron in the oceans, forming banded iron formations (BIFs). Many anaerobic organisms could not survive in oxygen-rich environments and either went extinct or adapted. Aerobic respiration evolved, leading to more efficient energy production (36-38 ATP vs. 2 ATP in anaerobic respiration). Key Takeaways ✔ Cyanobacteria were the first organisms to produce oxygen via photosynthesis. Lecture 3: Microbial Evolution and Diversity 8 ✔ They evolved from anaerobic photosynthetic bacteria that used H₂S instead of H₂O. ✔ Their oxygen production transformed Earth's atmosphere, leading to the rise of aerobic life. ✔ O₂ led to the formation of the ozone layer, protecting life from UV radiation. ✔ This process enabled the evolution of eukaryotic algae, plants, and ultimately more complex life forms. Endosymbiosis What Is Endosymbiosis? Endosymbiosis is a symbiotic relationship where one organism (endosymbiont) lives inside another (host cell) in a mutually beneficial or stable way. This process played a key role in the evolution of eukaryotic cells by incorporating smaller prokaryotic cells inside larger host cells. Key Concept: "Endo-" = Inside "Symbiosis" = Living together in a mutually beneficial relationship Lecture 3: Microbial Evolution and Diversity 9 The Endosymbiont Pathway The Endosymbiont Hypothesis suggests that modern eukaryotic cells evolved from symbiotic associations between different prokaryotic cells. According to this theory: 1. A large heterotrophic prokaryote (host cell) engulfed a smaller prokaryote (endosymbiont). 2. Instead of digesting it, the host cell kept the smaller cell alive because it provided some metabolic benefit. 3. Over time, the endosymbiont evolved into an organelle within the host cell, leading to the formation of mitochondria and chloroplasts. Modern Examples of Endosymbiosis: Rhizobium bacteria living inside plant roots (fixing nitrogen for plants). Coral and algae (zooxanthellae provide energy via photosynthesis). Evolutionary Variations of Endosymbiosis There are multiple versions of the Endosymbiotic Theory, but all involve a smaller bacterial cell being engulfed by a larger cell. The main variations explain the origin of: Mitochondria (from aerobic bacteria, likely related to Alphaproteobacteria). Chloroplasts (from photosynthetic bacteria, likely related to Cyanobacteria). Other cellular structures that might have evolved through similar processes. Modern Example – Giardia as an Evolutionary Intermediate The unicellular parasite Giardia is a modern-day eukaryote that has a nucleus but NO mitochondria. Why is this important? Lecture 3: Microbial Evolution and Diversity 10 It suggests an early stage of eukaryotic evolution before mitochondria were fully integrated. This supports the idea that some eukaryotic cells lost mitochondria over time. Giardia causes giardiasis, a gastrointestinal disease spread through contaminated water. Key Takeaways ✔ Endosymbiosis explains how eukaryotic cells evolved by engulfing prokaryotic cells. ✔ Mitochondria and chloroplasts likely originated from bacteria through endosymbiosis. ✔ Modern evidence (double membranes, independent DNA, binary fission) strongly supports this theory. ✔ Giardia is an example of a eukaryote without mitochondria, showing an intermediate evolutionary stage. Viruses Cellular Microorgansims: Lecture 3: Microbial Evolution and Diversity 11 1. Prokaryotes: eubacteria, archaebacteria & cyanobacteria (blue green alge) 2. Eukaryotes: fungi, protozoa, unicellular algae a lot of cellular microbes are infectious (exceptions - archaebacteria) Non-cellular (acellular) agents: Viruses Viroids Prions Plasmids Transposons All non-cellular agents are infectious. Characteristics of Acellular Microbes (Viruses): Not self-sustaining: Obligate intracellular parasites. No metabolism: Cannot generate energy or synthesize molecules outside the host. Reproduction: Use host machinery to replicate, harming the host in the process. Infectious Grouping Viruses: Based on: Shape of the capsid (protein coat: identical subunits, self assemble) … Icosahedral: Regular polyhedron w/ 20 equilateral triangular faces. helical: shaped like hollow protein cylinders Complex: neither purely icosahedral nor helical Presence or absence of an envelope. Type of nucleic acid (DNA or RNA, single or double-stranded). Disease or syndrome caused by virus Lecture 3: Microbial Evolution and Diversity 12 Human immunodeficiency virus (HIV) → immunodeficiency syndrome (AIDS) Influenza virus → Influenza (Flu) [symptoms fever, muscle aches, sore throat, cough & sometimes eye infection, conjunctivitis Examples of Virus Morphologies: Naked Virus: Lacks an envelope (e.g., Tobacco Mosaic Virus). Enveloped Virus: Surrounded by a lipid membrane (e.g., Influenza). Complex Virus: Combination of icosahedral and helical shapes (e.g., Bacteriophage T4). Host Specificity and Range: Determined by: Cell surface receptors for viral attachment. Mechanisms for viral entry and replication within the host. Examples: Bacteriophage (E. coli virus), Influenza (infects humans and birds). Viral Genome Variability: Can be organized as single or segmented molecules of DNA/RNA. Lecture 3: Microbial Evolution and Diversity 13 RNA viruses may have positive (sense) = same as that of a viral mRNA, such that RNA can be directly translated or negative (anti-sense) strands = packaged RNA is complementary to viral mRNA & can not be directly translated. Disease and Syndrome Caused by Viruses: Examples: HIV → AIDS. Influenza virus → Flu symptoms like fever, muscle aches, and sore throat. SARS-CoV → Severe Acute Respiratory Syndrome. Lecture 3: Microbial Evolution and Diversity 14