MIC 111 Lecture 22: Mobile Genetic Elements and Resistance PDF
Document Details
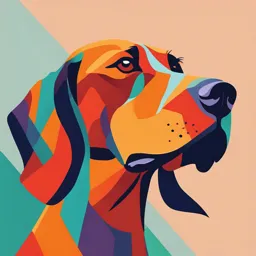
Uploaded by cookietongs
UC
Tags
Related
- Antibiotic Resistance Lecture 17 (PDF)
- Bacterial Genetics and Antibiotic Resistance PDF
- Antibiotic Resistance Lecture PDF
- Lesson 4: Acquisition and spread of antibiotic resistance PDF
- Antibiotic Resistance Profile of Pathogenic Bacteria from Mosul Government Hospital, Iraq PDF
- Antibiotic Resistance - Laura McCaughey - PDF
Summary
This lecture covers the short history of antibiotic discovery, screening methods for antibiotics, and the concepts of antibiotic resistance and the role of mobile genetic elements. It explains how antibiotics work, the mechanisms of resistance, how resistance spreads, and what plasmids are. The lecture also looks at how co-selection of antibiotic resistance affects microbiomes, and discusses the consequences of antibiotic use.
Full Transcript
Lecture 22: Mobile genetic elements and resistance Short history of antibiotic discovery Paul Ehlirch coined the terms “chemotherapy” and “antibiotic.” Found the drug (arsphenamine) with activity against Treponema pallidum (syphilis) in 1910. Alexander Fleming (1928) chance discovery...
Lecture 22: Mobile genetic elements and resistance Short history of antibiotic discovery Paul Ehlirch coined the terms “chemotherapy” and “antibiotic.” Found the drug (arsphenamine) with activity against Treponema pallidum (syphilis) in 1910. Alexander Fleming (1928) chance discovery of zone of clearing on agar plate from fungi (penicillium). Named extract “penicillin” - but this wasn’t made a therapeutic until Florey and Chain (1938) scaled up production Treated mice infected with Streptococcus with penicillin to cure infection He would need 2000 Liters of Penicillium culture to get enough penicillin to treat one person. More government and industrial scaling of antibiotic production during WW2. Screening for antibiotic sensitivity on bacterial “lawns” Selman Waksman screen soil actinomycetes for their ability to produce zones of growth inhibition with a test pathogen. Waksman and students discovered “actinomycin” in 1940 produced by actinomyces. Then “streptomycin” in 1942, produced by streptomyces. ○ This began the era of large-scale screening for antibiotics. Antibiotics (and Abx resistance) can be major disruptors of microbiomes Antibiotics target conserved components of cells like the cell wall and membranes and inhibit parts of the central dogma (replication, transcription, and translation) Antibiotic treatment reduces the overall diversity, including loss of important taxa Antibiotics causes metabolic shifts in communities, can reduce colonization resistance due to loss of taxa and can simulate the development of antibiotic resistance Survivor bacteria: not susceptible to antibiotics Lost bacteria: Susceptible or outcompeted Opportunistic bacteria: not susceptible to antibiotic but now lack competition after antibiotic use De novo (new) colonizer bacteria: lower abundance initially, then bloom after antibiotic What is antibiotic resistance? Four categories of resistance mechanisms: 1. Alters target of antibiotic - ex: mutations in the PBPs (Penicillin-binding proteins) stop methicillin from binding 2. Degrade antibiotic (outside the cell) - ex: beta-lactamase cleaves penicillins 3. Modify (inactivate) antibiotic - ex: adding methyl, acetyl, hydroxyl groups to kanamycin 4. Pump antibiotic out of cell - ex: multidrug transporter NorA in S. aureus Example resistance mechanisms for penicillin Penicillin interferes with PG (peptidoglycan) synthesis A plasmid-encoded antibiotic resistant gene produces beta-lactamase which cleaves penicillin Mutant PBPs or porins limit penicillin entry Efflux pumps pump antibiotic out How does antibiotic resistance spread? Antibiotics are made naturally some bacteria to kill other Bacteria rapidly reproduce and have extremely large populations “Standing variation” are rare members of a population possess antibiotic resistance genes Antibiotic resistance genes can thus be spread between different species of bacteria What are plasmids? Josh Lederberg coined “plasmid” which is a generic term for any extrachromosomal heredity determinant ○ Plasmids range from from thousands to millions of bp; circular dsDNA / ssDNA Episomes are non-essential genetic element replication autonomously or integrated into the chromosome Many plasmids carry useful “accessory” genes (not needed for replication/transfer) ○ Ex virulence, toxins, bacteriocins, siderophores, metabolism, exotic carbon sources, energy metabolism genes, chemotaxis, biofilm formation, antibiotic resistance, disinfectant resistance, metal resistance Co-selection of multiple antibiotic resistance games on same plasmid Co-selection: adding antibiotic A (or B or C) will select for all three resistance genes since there are on the same plasmid Plasmid can be transferred to other types of bacteria, bringing the multi-drug resistance along The plasmid “paradox” Use of antibiotics is a selective pressure to maintain plasmid with antibiotic resistant genes S. typhi can form “persister reservoirs” in host: antibiotic-resistant cells lurk in the gut tissue after an infection and migrate back into the gut lumen to cause reinfections after the antibiotic pressure is removed Plasmid paradox: maintenance of plasmids should have a fitness costs, but... plasmids are maintained in population off selection (no antibiotics). ○ Why not just integrate antibiotic resistance genes into the genome? ○ Does plasmid carry unknown fitness benefits besides antibiotic resistance? ○ Is plasmid acting as a “selfish” DNA element (only wants to replicate) Plasmid Experiment Researchers integrated the beta-lactamase gene into the E coli genome and compared to E coli cells carrying the beta-lactamase gene on plasmid the select with antibiotic E coli with the plasmid were able to grow at higher concentrations of antibiotic than the bacterial population encoding the beta-lactamase gene in the chromosome Strains with plasmids had better fitness - likely due to higher number beta-lactamase genes on multiple plasmid copies Viruses or bacterio(phage) also shape microbiomes Bacterio (phages) also contribute to the structure microbial communities Mediate genetic Exchange between hosts (like plasmids) Can shape microbiome diversity and expand the functional diversity in microbiomes “Phageome” are all the viral components of microbiome Capsid is the head, tail is where they inject genome Genome can be dsDNA, ssDNA, dsRNA, or ssRNA Phages and microbiomes 1200 viral genotypes associated with gut microbiome Phages are drivers of microbiome diversity c Can be biomarkers of disease in food and host Can give description of host microbiome diversity Used as delivery of genetic therapies Can be a treatment of bacterial diseases Phages alter microbiome diversity in infant microbiomes Infants colonized by viruses early after birth, within 0-4 days after birth During the first days of life, phage alpha diversity and bacterial alpha diversity low Reduction in Caudovirales diversity linked to expansions and diversity shifts in the microbial community composition. Increases in Microviridae phage abundance with age and with infant gut microbiome diversity. Stable phageome diversity (like gut microbiome) is maintained during the adult life. Virus Cycle Lytic Cycle: Attachment, Penetration, Biosynthesis, Maturation, Release Lysogenic Cycle: Attachment, Penetration, Integration, Cloning, Biosynthesis, Maturation, Release Costs of phage infection Infection can causes cell death (lysis), or temporary symbiosis (lysogeny, chronic infections) Lytic phages (virulent phages): inject viral genome into the cell, replicate, and lyse their host cell. Release virions that infect other hosts. Lysogenic phages (temperate phages), inject viral genome into host cell, than can either enter lytic or lysogenic cycle. May be a fitness cost to lysogeny Prophage =integrated phage. Prophages can activate and switch to the lytic cycle upon certain triggers (e.g., stress) Pseudolysogeny: viral DNA is maintained as an episomal plasmid. Once favorable conditions arise, the phage enters lytic or lysogenic cycle. Pseudolysogeny can lead to persistent infections: Bacteroides and Escherichia species are be infected this way Benefits of Phage Infection Lysogeny can influence the community composition through indirect benefits to prophage carrying bacterial hosts or horizontal gene transfer (HGT) of beneficial genes between hosts Prophage-encoded advantages include: protection from superinfection (infection with > 1 virus) Release of activated prophages that can lyse competing species Prophages can encode prophage can have genes that increase fitness ○ carbohydrate transport and degradation ○ resistance to antibiotics ○ pathogenesis determinants such as toxins or host adherence factors Phage-host arms race Hosts are under constant and strong selection to inhibit phage infection; Phages are under constant and strong selection to infect hosts ○ 1.Phage adsorption & surface resistance ○ 2.Restriction modification systems ○ 3.CRISPR/Cas9 adaptive immunity Phage Resistance and Counter-Resistance phage resistance: host mutations can prevent phage adsorption or injection (receptors, etc), CRISPR/Cas9, superinfection counter -resistance: phages adapt/mutate to overcome to host resistance or adapt to new hosts or encode anti-CRISPR proteins (ACRs) CRISPR spacers record of phage CRISPR/Cas9 is an adaptive, heritable, genetic immune system for bacteria: During adaptation, the CRISPR acquires spacers from the phages that the bacteria encounter. Spacers are inserted adjacent to the leader sequence at the start of the CRISPR array (Cas1 and Cas2) proteins. During expression, small CRISPR RNAs (crRNAs) are produced from transcripts of the CRISPR array. During interference, the crRNAs guide Cas9 to matching DNA sequences of invading phages, which are then cleaved by the Cas9 proteins. CRISPR-spacers thus have a record of past phage exposure that can help track hosts Role of phages in colonization resistance Bacteriophages can impact colonization resistance (positively or negatively) Pro-phages (lysogenic phages) in the microbiome can activate and become lytic in stress situations caused by pathogens Then lytic phages can infect other bacteria in the microbiome (lytic or lysogenic) lytic phages act on the fastest-growing sensitive population and limit growth of specific taxa but pathogens could also be sensitive to phages in the host “phageome loss/gain of taxa can then lose/increase mechanisms of colonization resistance (e.g, metabolic competition or production of AMPs) Resilience in phagenome contributes to resilience Resilience: healthy microbiomes recover quickly from perturbations, after which a new “steady state” is established. Antibiotic treatment can change the microbial community, but changes in the viral community are only moderate after antibiotic treatment. Phage–bacteria network interactions change during dysbiosis. New phage-host networks are established in the new steady states. Phages may improve the recovery microbiome diversity after dysbiosis due to movement (transfer) of beneficial genes between different bacterial hosts.