Metabolism PDF
Document Details
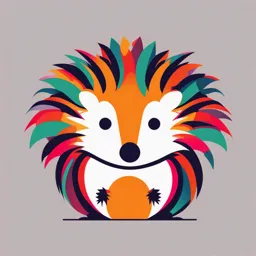
Uploaded by AmusingBambooFlute
The University of Hong Kong
Tags
Summary
This document provides an overview of metabolism, focusing on glucose, lipid, and amino acid metabolism. It covers topics such as the structure, digestion, absorption, and metabolic pathways for each. The document further details the regulation of glucose in the blood.
Full Transcript
4. Metabolism 4.1. Glucose metabolism 4.1.1. Structure Starch: major carbohydrate in diet Glycogen: fuel molecules our body stores Glucose: common monomer 4.1.2. Digestion Ca...
4. Metabolism 4.1. Glucose metabolism 4.1.1. Structure Starch: major carbohydrate in diet Glycogen: fuel molecules our body stores Glucose: common monomer 4.1.2. Digestion Carbohydrates Enzyme Amylose Salivary & (unbranched, pancreatic α- α-1,4 amylases glycosidic bond) Amylopectin (branched, α- 1,4 & α-1,6 glycosidic bond) Disaccharides Glycosidase Break down disaccharides to monosaccharides @ brush Lactase border of SI ○ Lactose → glucose + galactose Maltase ○ Maltose → 2 glucose Sucrase ○ Sucrose → glucose + fructose 4.1.3. Absorption Glucose transporter: GLUT 1 Bumble Bee’s Best Blood Foetus Blood brain barrier GLUT 2 Last Known Phrase Is Liver Kidney Pancreas Intestine GLUT 3 No Problem Neuron Placenta GLUT 4 My Friends Muscle Fat/ adipose (insulin dependent) 4.1.4. Metabolic pathways Glycolysis Glucose → pyruvate Aerobic condition: Anaerobic condition: Pyruvate → lactate Glycogenesis Glucose → glycogen Glycogenolysis Glycogen → glucose Gluconeogenesis Pyruvate → glucose Overview: 4.1.5. Abnormal glucose metabolism Regulation of glucose in blood: Blood glucose in blood Hormone levels Nerve impulse Negative feedback mechanism: Fasting Plasma Blood glucose in a person who has not eaten anything for at least 8 Glucose (FPG) hours Oral Glucose Blood glucose after a person fasts at least 8 hours and 2 hours after the Tolerance Test person drinks a glucose-containing beverage (OGTT) 1. OGTT must begin in the morning since glucose tolerance exhibits a diurnal rhythm with a significant decrease in the afternoon 2. Blood samples are collected at regular intervals up to 2 hours after the glucose challenge Random plasma Blood glucose without regard to when the person being tested last ate glucose test Enzyme Based Glucose Detection Colour intensity is measured by spectrophotometer to determine glucose concentration Dry strip: enzymes are in lyophilised form Wet strip: water reconstitute protein & chemical buffer can work to help enzymes carry out catalytic activities Chromogenic mixture: combination of reagents that produce colour change when glucose is present ○ Glucose oxidase ○ Peroxidase ○ o-dianisidine 4.2. Lipid metabolism 4.2.1. Structure Triacylglycerol (TAG): Fatty acids: Essential: linolenic acid Significant quantities in milk: capric acid Precursor of prostaglandins: arachidonic acid 4.2.2. Digestion Hormone Cholecystokinin Secrete bile acids (gallbladder) + digestive enzymes (pancreas) (by intestine) Hormone Secretin Secret bicarbonate (liver, pancreas, intestine cell) (by intestine) Lingual lipase & gastric Minor hydrolysis of TAG lipase Pancreatic lipase Hydrolysis of TAG 4.2.3. Absorption Formation of Small intestine Free fatty acids & monoglyceride bombine with bile salts micelles to form micelles To transport lipid to enterocytes Enterocyte Enterocytes Micelles diffuse through the membrane uptake (intestinal cells) Small micelles directly enters the blood capillary Larger micelles package as TAG into chylomicrons Re-esterification Enterocytes Fatty acids combine with monoglyceride to form TAG Chylomicron Enterocytes Packaging of TAGs, cholesterol, phospholipid, formation apolipoprotein into chylomicrons To transport lipid into the bloodstream Transport Lacteal (lymphatic Chylomicrons released to the lymphatic system enter the system) blood circulation at left subclavian vein 4.2.4. Accumulation of TAG Major site White adipose tissue Brown adipose tissues Energy storage Dissipate energy into heat Fuel molecule to generate ATP High mitochondrial content → Convert acetyl-CoA into fatty acids more enzymes to carry out (in humans the liver is the preferred oxidative phosphorylation place for FAsynthesis) Brown due to presence of haem- Use FA to make TAGs containing protein ○ FA from intestinal lipids via Primarily found in newborns, around chylomicrons/ liver via kidneys, spine VLDL Release FA when other tissues need them (skeletal muscle, heart) Minor site Liver Skeletal muscle 4.2.5. Storage of TAG in adipose tissue 4.2.6. TAG mobilisation Hormonal regulation Glucagon ○ Released in response to low blood glucose levels → promote breakdown of TAGs Triggered by stress condition (cortisol) Activation of lipase Hormones bind to specific receptors on adipocyte membranes Hydrolysis of TAG TAG breaks down into fatty acids and glycerol → travel to liver via blood Metabolism in liver Fatty acid: beta-oxidation Glycerol → gluconeogenesis Beta-oxidation Conversion of fatty acid to acetyl-CoA ○ acetyl-CoA is feed into the TCA cycle to released ATP Formation of Excess acetyl-CoA produced from beta-oxidation is converted to ketone bodies ketone bodies (during fasting/ prolonged exercise/ low-carbohydrates diets) (ketogenesis) acetyl-CoA → ketone bodies → blood Excess ketone bodies → metabolic acidosis (ketoacidosis) Ketone bodies DO NOT serve as a reserve De novo fatty acid synthesis 4.3. Amino acid metabolism 4.3.1. Structure 4.3.2. Digestion Protein Enzymes Protein/ Pepsin (gastric juice) Pepsinogen (inactive form) → pepsin polypeptide (active form) Stimulated by acidic environment (HCl) Peptides Endopeptidase Trypsinogen Trypsinogen → trypsin (pancreatic juice) Activated by enteropeptidase Chymotrypsinogen Chymotrypsinogen → chymotrypsin Proelastase Proelastase → elastase Procarboxypeptidase Procarboxypeptidase A & B → A&B carboxypeptidase A & B Exopeptidase Aminopeptidase (pancreatic juice) Di/Tripeptides Di/ Tripeptidase (intestinal juice) 4.3.3. Absorption 4.3.4. Protein degradation Proteasomal degradation Ubiquitin-proteasome system (UPS) Protein tagged with ubiquitin (Ub) is destined for degradation ATP dependent Lysosomal degradation Lysosome engulf protein 4.3.5. Metabolic pathway Transamination When the amino group of an amino acid is transferred to a carbon skeleton (𝛼- keto acid), forming a new amino acid A typical reaction coupled with amino acids transaminases and cofactors Fate of alpha-keto acid: Enter the TCA Cycle Reversible: TCA intermediates can also be used to make amino acids → possible for the liver to metabolic protein as energy source (during starvation) Deamination Urea cycle To convert toxic ammonia species into urea → maintain nitrogen balance in body Urea: soluble, non-toxic carrier of nitrogen, excretory product (blood → kidney → urine) Takes place in the liver hepatocytes (urea is synthesised in liver) Alanine & glutamine: major nitrogen carriers of amino acid nitrogen from peripheral tissues to the liver Urea is a soluble, non-toxic carrier of nitrogen 1 nitrogen comes from ammonium ion (released from deamination/ glutamate/ aspartate) Disorders of urea cycle: hyperammonemia Glucose/ alanine Alanine is primarily synthesised from pyruvate & glutamate cycle ○ Glutamate + Pyruvate ⇌ Alanine + α-Ketoglutarate Carbons of alanine are used for gluconeogenesis Nitrogen is used for urea biosynthesis Occurs mainly in muscle tissue Glutamine: forms ammonia and glutamate Glutamate forms urea and 𝛼-ketoglutarate Glutamine can be synthesised by 𝛼-ketoglutarate accepting two ammonium ions Glutamine can be formed in the muscles and peripheral tissue Glutaminase converts glutamine to glutamate (to 𝛼-ketoglutarate) Found in the liver Overview: 1. Maintenance of blood amino acid pool 2. Free amino acids from dietary proteins/ endogenous protein turnovers can provide source of essential amino acids 3. Blood amino acids can be used to form new proteins or part of nucleotide synthesis 4. Other compounds synthesised from amino acid precursors are essential for physiological functions (nucleotides, neurotransmitters, hormones, etc.)/ Amino acids degraded to nitrogen containing urinary metabolites and do not return to the free amino acid pool 5. Amino acid carbon skeletons are recycled for gluconeogenesis and other processes for energy generation 6. Nitrogen is removed from amino acids; nitrogen in amino acid degradation primarily appears in urine as urea, ammonia or ammonium Overview: 4.4. Generation of ATP 4.4.1. Definition Need for nutrients Catabolised to feed into the reactions leading to ATPsynthesis Some nutrients form structural components of proteins driving the ATP synthesis reactions Need for oxygen Oxygen sits at the final stage of electron transport chain X oxygen → X ATP produced → X cell functioning Structure: Break in phosphoanhydride bonds (phosphate bonds) generates energy 7.3 kcal/mol is released from the break of ONE phosphoanhydride bond in ATP 4.4.2. TCA cycle Goal: to generate NADH and FADH2 molecules 3 NAD+ and 1 FAD → 3 NADH and 1 FADH2 Mitochondria: Outer membrane: in contact with the cell cytosol, permeable to ions & molecules that are small enough to pass through Inner membrane: site for oxidative phosphorylation Inner circular space (matrix): site for TCA cycle ADP will stimulate TCA cycle ATP will inhibit TCA cycle NADH will inhibit TCA cycle 4.4.3. Oxidative phosphorylation Goal: to couple energy stored in electron acceptors to a proton gradient that drives ATP synthesis A. Electron transport chain 1. Complex I Accepts electrons from NADH Become supercharged, pumps protons (H⁺) from the matrix to the intermembrane space Transfers electrons to CoQ 2. Complex II Accepts electrons from FADH₂ Transfers electrons to CoQ Does not pump protons 3. Complex III Accepts electrons from CoQ Become supercharged, pumps protons (H⁺) from the matrix to the intermembrane space Transfers electrons to Cyt C 4. Complex IV Accepts electrons from Cyt C Become supercharged, pumps protons (H⁺) from the matrix to the intermembrane space Transfers electrons to oxygen (O₂), final electron acceptor ○ Combines electrons with protons and oxygen to form water (H₂O) B. Chemiosmosis 1. The electron transport creates a high concentration of protons in the intermembrane space, establishing an electrochemical gradient (proton motive force) 2. ATP synthase The flow of protons through ATP synthase (from intracellular space to matrix) drives the phosphorylation of ADP to ATP 3. ATP production Facilitate the exchange of ATP and ADP across the inner mitochondrial membrane Transports ADP into the mitochondria in exchange for ATP out into the cytosol, maintaining the necessary balance of nucleotides for continued ATP synthesis 4.4.4. ATP yield 4.4.5. Inhibitors and uncouplers A. Inhibitors Inhibition of: Inhibitor Complex I Rotenone (insecticide) FADH2 can still donate Amytal electrons via Complex II (barbiturates 安 眠藥) Complex III Antimycin (antibiotic) Prevents transfer of electrons before Complex III to after Complex III Complex IV Cyanide None of the complexes can Carbon monoxide pump protons, no ATP is synthesised Absence of Cyanide Bind to haemoglobin, oxygen ○ Binds to iron in blocking oxygen binding the heme of and transport cytochrome Carbon monoxide ATP Oligomycin Accumulation of protons synthase outside the mitochondria B. Uncouplers “Proton leak” created by carrier protein Protons re-enter the mitochondrial matrix without energy being captured as ATP Energy is released as heat Some synthetic uncouplers mimic this mechanism 4.4.6. Disorder Inherited Defective protein for oxidative phosphorylation diseases Mitochondrial encephalomyopathies → lactic acidosis ○ Cells are incapable of pyruvate oxidation in TCAcycle → conversion of pyruvate to lactate → lactate is released & accumulate in blood Vitamin/ mineral Iron deficiency deficiency ○ Affect iron-containing haem protein of electron transport chain Thiamine deficiency ○ Necessary for alpha-ketoglutarate dehydrogenase (coenzymes of TCA cycle) Obesity & Induces synthesis of uncoupler proteins (UCP2) in cells diabetes that makes insulin Lowers ATP concentration in cells for insulin secretion → type 2 diabetes 4.5. Integrated metabolism 4.5.1. Regulation of metabolic activities Enzymes to carry out both catabolic and anabolic reactions of the important biomolecules (carbohydrates, lipids and proteins) Simultaneous degradation and synthesis of biomolecules is energetically wasteful → when one pathway is active the other is suppressed Other regulation mechanisms At cellular level: Different cellular compartments: concentrations of intermediates, enzymes and regulators can be maintained at different levels E.g. fatty acid catabolism: mitochondria || fatty acid synthesis: cytosol Role and mechanism of specific enzymes Flux of metabolites through pathways Feedback regulation of metabolic pathways Transport of metabolites across organelle membranes At whole organism level: Metabolic activities of different tissues are regulated & integrated by growth factors/ hormones acting from outside the cells Flux of metabolites from organs to organs E.g. liver to brain/ liver to skeletal muscles 4.5.2. Metabolic homeostasis Goal: to provide a constant flow of fuel to make ATP Balance of nutrients taken in (availability) and their expenditure (need) Balance between: ○ Intake of carbohydrate, fat and protein ○ Rates of oxidation of carbohydrate, fat and protein ○ Rates of storage of these 3 macromolecules when they are in excess of immediate need When the demand of these carbohydrate, fat and protein increases for other purposes, we need a balanced regulation for: ○ Rate of mobilisation from storage sites ○ Rate of de novo synthesis Ways to achieve metabolic homeostasis 1. Concentration of nutrients or metabolites the blood affect the rate at which they are used or stored in different tissues 2. Hormones carry messages to their target tissues about the physiological state of the body and the nutrient supply or demand 3. The central nervous system using neural signals to control metabolism in tissues directly or via the release of hormones Example: maintenance of blood glucose level Insulin Major anabolic hormone Promotes storage of fuels ○ Glucose storage as glycogen in liver and muscle ○ Converting glucose to triacylglycerols in liver and storage in adipocytes (fat cells) ○ Stimulates amino acid uptake and protein synthesis in liver and muscle ○ Promotes usage of glucose as fuel by facilitating its transport to muscle and fat cells ○ Inhibits fuel mobilisation from stores like glycogen, triacylglycerols and proteins Glucagon Insulin counter-regulatory hormone Maintains fuel availability when there is no dietary glucose ○ Stimulates the release of glucose from liver glycogen during fasting ○ Stimulates the gluconeogenesis from lactate, glycerol and amino acids ○ Mobilises fatty acids from adipose triacylglycerol to provide an alternative source of energy NO effect on skeletal muscle metabolism Epinephrine Insulin counter-regulatory hormones Mobilises fuels during acute stress opposing the action of insulin (stress Stimulates glucose production from hormones) glycogen (muscle and liver) Stimulates fatty acid release from adipose tissue Cortisol Provides for changing requirements during stress Stimulates amino acid mobilisation from muscle protein Stimulates gluconeogenesis in order to produce glucose for liver glycogen synthesis Stimulates fatty acid release from Mediated by neuronal signals adipose tissue 4.5.3. Integrated metabolic pathway A. Carbohydrate metabolism in the liver (numbering not sequential) Glucose entering hepatocytes will be converted to glucose-6-phosphate (G6P) 1. G6P is dephosphorylated to free glucose exported back into the blood to send to other tissues 2. G6P not immediately needed is converted toliver glycogen 3. Yields acetyl CoA to be oxidised for energy 4. Acetyl CoA can serve as precursors of fatty acids and cholesterol 5. Enter the pentose phosphate pathway (PPP)giving both reducing power NADPH for biosynthesis and ribose-5-phosphate for nucleotide biosynthesis B. Amino acid metabolism in the liver (numbering not sequential) 1. Precursors for protein synthesis 2. Passed in the blood to other organs to synthesise tissue proteins 3. Precursors in the biosynthesis of nucleotides, hormones and other nitrogenous compounds 4. Amino acids not needed are transaminated or deaminated a. Degraded to yield pyruvate and citric acid cycle intermediates b. The ammonia released is converted to urea 5. Pyruvate can be converted to glucose and glycogen 6. Pyruvate can be converted to acetyl-CoA 7. Acetyl-CoA can be oxidised via citric acid cycle 8. Acetyl-CoA can undergo oxidative phosphorylation to get ATP 9. Acetyl-CoA can be converted to lipid for storage 10. Citric acid cycle intermediates can be used for gluconeogenesis 11. Alanine from muscles is transported to liver via and is converted to pyruvate C. Fatty acid metabolism in the liver (numbering not sequential) 1. Some fatty acids are converted to liver lipids 2. Fatty acids are the primary fuel in the liver. Free fatty acids are activated and oxidised to yield acetyl-CoA and NADH 3. Acetyl-CoA is oxidised in the citric acid cycle 4. Synthesise ATP from oxidative phosphorylation 5. Excess acetyl-CoA is converted to ketone bodies and enters the blood 6. Acetyl-CoA can also be used for making cholesterol 7. Fatty acids are converted to phospholipids and triacylglycerols of plasma lipoproteins 8. Free fatty acids enters the blood by binding to serum albumin and used in the heart and skeletal muscle D. Metabolism in muscles Energy sources for muscle contraction: O2 debt After vigorous exercise, rapid breathing continues Oxygen used for oxidative phosphorylation to build proton gradient & replenish ATP ATP used for gluconeogenesis to use up lactate & restore muscle glycogen concentrations Cori cycle Muscles under strenuous exercise use glycogen as energy source → generating lactate via glycolysis During recovery, lactate is transported to liver → converted to glucose by gluconeogenesis Glucose is released into blood and returned to the muscles to replenish the glycogen stores E. Metabolism in brain F. Metabolism in liver Well-fed state Fasting Prolonged fasting/ Type I diabetes Muscle begins to be used for fuel ○ The liver deaminates or transaminates amino acids Converts amino groups to urea C skeletons of glucogenic amino acids converted to pyruvate, then glucose via gluconeogenesis Provides glucose for brain ○ FA is oxidised to acetyl-CoA, but oxaloacetate is depleted to make glucose → ketone bodies are formed from the accumulated acetyl-CoA Exported to other tissues Reduction in blood pH (e.g. ketoacidosis) Diabetes Mellitus: Type I diabetes Type II diabetes Insufficient production of insulin Insulin resistance ○ due to autoimmune destruction of Develop in late adulthood beta cells Usually associated with obesity → cell do Develop early in life not respond appropriately to insulin Insulin dependent/ juvenile diabetes Diabetes symptoms: Blood sugar becomes elevated The body tries to dilute the glucose with water → excessive urination & thirst T1DM: fat breakdown is accelerated → high production of ketone bodies → raise blood [H+] → ketoacidosis ○ Activate bicarbonate buffering system → altered breathing ○ Breakdown of ketone body acetoacetate produces acetone → expelled via breath