Metabolic Pathways PDF
Document Details
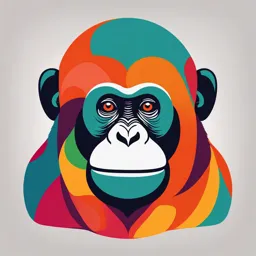
Uploaded by HeartfeltUnakite2024
Damanhour University
Tags
Summary
This document discusses metabolic pathways, emphasizing the importance of describing chemical changes in terms of enzymes and the diverse intracellular controls that regulate these pathways. Examining whole organisms and organs also reveals substance conversion processes and their localized tissue origins. Additional methods, such as studying metabolites under stress conditions, analyzing metabolic poisons, and tracing nutrient fates using isotopes are described as crucial tools for metabolic pathway analysis.
Full Transcript
The study of metabolic pathways: There are two main reasons for studying a metabolic pathway: 1. To describe, in quantitative terms, the chemical changes catalyzed by the component enzymes of the route; and 2. To describe the various intracellular controls that govern the rate at whic...
The study of metabolic pathways: There are two main reasons for studying a metabolic pathway: 1. To describe, in quantitative terms, the chemical changes catalyzed by the component enzymes of the route; and 2. To describe the various intracellular controls that govern the rate at which the pathway functions. Studies with whole organisms or organs can provide information that one substance is converted to another and that this process is localized in a certain tissue; for example, experiments can show that urea, the chief nitrogen-containing end product of protein metabolism in mammals, is formed exclusively in the liver. They cannot reveal, however, the details of the enzymatic steps involved. Clues to the identity of the products involved, and to the possible chemical changes effected by component enzymes, can be provided in any of four ways involving studies with either whole organisms or tissues. First, under stress or the imbalances associated with diseases, certain metabolites may accumulate to a greater extent than normal. Thus, during the stress of intense exercise, lactic acid appears in the blood, while glycogen, the form in which carbohydrate is stored in muscle, disappears. Such observations do not, however, prove that lactic acid is a normal intermediate of glycogen catabolism; rather, they show only that compounds capable of yielding lactic acid are likely to be normal intermediates. Indeed, in the example, lactic acid is formed in response to abnormal circumstances and is not directly formed in the pathways of carbohydrate catabolism. Second, the administration of metabolic poisons may lead to the accumulation of specific metabolites. If fluoroacetic acid or fluorocitric acid is ingested by animals, for example, citric acid accumulates in the liver. This correctly suggests that fluorocitric acid administered as such, or formed from fluoroacetic acid via the tricarboxylic acid (TCA) cycle, inhibits an enzyme of citrate oxidation. Third, the fate of any nutrient indeed, often the fate of a particular chemical group or atom in a nutrient can be followed with relative ease by administering the nutrient labeled with an isotope. 1 Isotopes are forms of an element that are chemically indistinguishable from each other but differ in physical properties. The use of a nonradioactive isotope of nitrogen in the 1930s first revealed the dynamic state of body constituents. It had previously been believed that the proteins of tissues are stable once formed, disappearing only with the death of the cell. By feeding amino acids labeled with isotopic nitrogen to rats, it was discovered that the isotope was incorporated into many of the amino acids found in proteins of the liver and the gut, even though the total protein content of these tissues did not change. This suggested that the proteins of these tissues exist in a dynamic steady state, in which relatively high rates of synthesis are counterbalanced by equal rates of degradation. Thus, although the average liver cell has a life-span of several months, half of its proteins are synthesized and degraded every five to six days. On the other hand, the proteins of the muscle or the brain, tissues that (unlike the gut or liver) need not adjust to changes in the chemical composition of their milieu, do not turn over as rapidly. The high rates of turnover observed in liver and gut tissues indicate that the coarse controls, exerted through the onset and cessation of synthesis of pacemaker enzymes, do occur in animal cells. Finally, genetically altered organisms (mutants) fail to synthesize certain enzymes in an active form. Such defects, if not lethal, result in the accumulation and excretion of the substrate of the defective enzyme; in normal organisms, the substrate would not accumulate, because it would be acted upon by the enzyme. The significance of this observation was first realized in the early 20th century when the phrase “inborn errors of metabolism” was used to describe hereditary conditions in which a variety of amino acids and other metabolites are excreted in the urine. METABOLISM OF CARBOHYDRATES Digestion of Carbohydrates Dietary carbohydrates principally consist of the polysaccharides: starch and glycogen. It also contains disaccharides: sucrose, lactose, maltose and in small amounts monosaccharides like fructose and pentoses. Liquid food materials like milk, soup, fruit juice escape digestion in mouth as they are swallowed, but solid foodstuffs are masticated thoroughly before they are swallowed. 2 1. Digestion in Mouth Digestion of carbohydrates starts at the mouth, where they come in contact with saliva during mastication. Saliva contains a carbohydrate splitting enzyme called salivary amylase (ptyalin). Action of ptyalin (salivary amylase) It is α - amylase, requires Cl- ion for activation and optimum pH 6-7. The enzyme hydrolyzes α- (1, 4) glycosidic linkage at random, from molecules like starch, glycogen and dextrins, producing smaller molecules maltose, glucose and disaccharides maltotriose. Ptyalin action stops in stomach when pH falls to 3.0 2. Digestion in Stomach No carbohydrate splitting enzymes are available in gastric juice. HCl may hydrolyze some dietary sucrose to equal amounts of glucose and fructose. 3. Digestion in Duodenum Food reaches the duodenum from stomach where it meets the pancreatic juice. Pancreatic juice contains a carbohydrate-splitting enzyme pancreatic amylase. Action of pancreatic Amylase It is also an α - amylase, optimum pH 7.1. Like ptyalin it also requires Cl- for activity. The enzyme hydrolyzes α-(1, 4) glycosidic linkage situated well inside polysaccharide molecule. Other criteria and end products of action are similar of ptyalin. 4. Digestion in Small Intestine Action of Intestinal Juice a. Pancreatic amylase: It hydrolyzes terminal α-(1,4), glycosidic linkage in polysaccharides and Oligosaccharide molecules liberating free glucose molecules. b. Lactase It is a β- glycosidase, its pH range is 5.4 to 6.0. Lactose is hydrolyzed to glucose and galactose. 3 Lactose Intolerance Lactose intolerance is a decreased ability to digest lactose, a sugar found in dairy products. Those affected vary in the amount of lactose they can tolerate before symptoms develop. Symptoms may include abdominal pain, bloating, diarrhea, gas, and nausea. These symptoms typically start thirty minutes to two hours after eating or drinking milk-based food. Their severity typically depends on the amount a person eats or drinks. Lactose intolerance does not cause damage to the gastrointestinal tract. Lactose intolerance is due to the lack of the enzyme lactase in the small intestines to break lactose down into glucose and galactose. There are four types: primary, secondary, developmental, and congenital. Primary lactose intolerance occurs as the amount of lactase declines as people age. Secondary lactose intolerance is due to injury to the small intestine. Such injury could be the result of infection, celiac disease, inflammatory bowel disease, or other diseases. Developmental lactose intolerance may occur in premature babies and usually improves over a short period of time. Congenital lactose intolerance is an extremely rare genetic disorder in which little or no lactase is made from birth. Diagnosis may be confirmed if symptoms resolve following eliminating lactose from the diet. Other supporting tests include a hydrogen breath test and a stool acidity test. Other conditions that may produce similar symptoms include irritable bowel syndrome, celiac disease, and inflammatory bowel disease. Lactose intolerance is different from a milk allergy. Management is typically by decreasing the amount of lactose in the diet, taking lactase supplements, or treating the underlying disease. People are usually able to drink at least one cup of milk per sitting without developing significant symptoms, with greater amounts tolerated if drunk with a meal or throughout the day. c. Maltase The enzyme hydrolyzes the α -(1,4) glycosidic linkage between glucose units in maltose molecule liberating two glucose molecules. Its pH range is 5.8 to 6.2. 4 d. Sucrase PH ranges 5.0 to 7.0. It hydrolyzes sucrose molecule to form glucose and fructose. Absorption of Carbohydrates Products of digestion of dietary carbohydrates are practically completely absorbed almost entirely from the small intestine. Absorption from proximal jejunum is three times greater than that of distal ileum. It is also proved that some disaccharides, which escape digestion, may enter the cells of the intestinal lumen by “pinocytosis” and are hydrolyzed within these cells. No carbohydrates higher than the monosaccharides can be absorbed directly in to the blood stream. Liver Metabolism The liver is the first organ to “get its pick” of the nutrients which enter the body from the intestine after a meal, and we might therefore predict that it would have a major role in energy storage after a meal. This is indeed so, at least for carbohydrate; storage and later release of glucose are major functions of the liver. It also has an important role in amino acid metabolism. Although most dietary fat bypasses the liver as it enters the circulation, the liver does have important roles in fat metabolism. Also, short- and medium-chain fatty acids from the diet reach the liver directly in the portal vein. Carbohydrate Metabolism in the Liver Fed Conditions Glucose is absorbed from the intestine into the portal vein, where its concentration may reach almost 10 mmol/l after a meal. (Arterial blood glucose concentration is normally around 5 mmol/l.) The hepatocytes, are exposed to high concentrations of 5 glucose during the absorptive phase. Liver cells have predominantly the GLUT-2 type of glucose transporter, which is not responsive to insulin, and has a relatively high Km for glucose; there is a high maximal activity (Vmax) for glucose transport. This means that the rate and direction of movement of glucose across the hepatocyte membrane are determined by the relative glucose concentrations inside and outside the cell. Within the hepatocyte, glucose is phosphorylated to form glucose 6-phosphate – the initial step in its metabolism by any pathway – by the enzyme glucokinase. This enzyme belongs to the family of hexokinases (hexokinase Type IV), but differs from the hexokinases found in muscle and other tissues in that it has a high Km for glucose (12 mmol/l) and is not inhibited by its product, glucose 6-phosphate, at physiological concentrations. Like the GLUT2 transporter it has a high capacity (high Vmax) and is unaffected, in the short term, by insulin. The overall result is that when the glucose concentration outside the hepatocyte rises, glucose will be taken into cells and phosphorylated The presence of the high-Km glucose transporter and the high-Km glucokinase would not, alone, enable the hepatocyte to take up unlimited quantities of glucose, as glucose 6-phosphate would simply accumulate within the cell until glucose phosphorylation ceased. Instead, there are specific mechanisms for stimulating the disposal of glucose 6-phosphate in the period after a meal. Glucose 6-phosphate may enter the pathways of glycogen synthesis or glycolysis GLYCOLYSIS Oxidation of glucose or glycogen to pyruvate and lactate is called glycolysis. This was described by Embeden, Meyerhoff and Parnas. Hence it is also called as Embden Meyerhoff pathway. 6 It occurs virtually in all tissues. Erythrocytes and nervous tissues derive its energy mainly form glycolysis. This pathway is unique in the sense that it can utilize O 2 if available (‘aerobic’) and it can function in absence of O2 also (‘anaerobic’) 7 Aerobic Phase 1. Aerobic phase includes the conversion of glucose to pyruvate 2. Oxidation is carried out by dehydrogenation and reducing equivalent is + transferred to NAD. NADH H+ in presence of O2 is oxidized in electron- transport chain producing ATP. Anaerobic Phase 1. This phase includes the conversion of Glucose to lactate 2. NADH cannot be oxidized, so no ATP is produced in electron transport chain. But the NADH is oxidized to NAD+ by conversion of pyruvate to lactate, without producing ATP. 3. Anaerobic phase limits the amount of energy per molecule of glucose oxidized. Hence, to provide a given amount of energy, more glucose must undergo glycolysis under anaerobic as compared to aerobic. Enzymes: Enzymes involved in glycolysis are present in cytoplasm. SIGNIFICANCE OF THE PATHWAY: 1. The glycolytic pathway is employed by all tissues for the breakdown of glucose to provide energy in the form of ATP. 2. Important pathway for the production of energy especially under anaerobic conditions. 3. It is crucial for generation of energy in cells without mitochondria. 4. It forms products that are intermediates for other metabolic pathways. 5. Glycolysis interfaces with glycogen metabolism, the pentose phosphate pathway, the formation of amino sugars, triglyceride synthesis (by means of 8 glycerol 3-phosphate), the production of lactate (a dead-end reaction), and transamination with alanine. REACTIONS OF GLYCOLYSIS The conversion of glucose to pyruvate occurs in two stages. 1. The first five reactions of glycolysis correspond to an energy investment phase in which the phosphorylated forms of intermediates are synthesized at the expense of ATP. 2. The subsequent reactions of glycolysis constitute an energy generation phase in which a net of two molecules of ATP are formed by substrate level phosphorylation per glucose molecule metabolized. 9 1. Uptake of Glucose by Cells and its phosphorylation Glucose is freely permeable to Liver cells. In Intestinal mucosa and kidney tubules, glucose is taken up by ‘active’ transport. In other tissues, like skeletal muscle, cardiac muscle, diaphragm, adipose tissue etc. Insulin facilitates the uptake of glucose. Glucose is then phosphorylated to form glucose -6- Phosphate. The reaction is catalyzed by the specific enzyme glucokinase in liver cells and by nonspecific Hexokinase in liver and extrahepatic tissues. 10 ATP acts as PO4 donor in the presence of Mg.One high energy PO4 bond is utilized and ADP is produced. The reaction is accompanied by considerable loss of free energy as heat, and hence under physiological conditions is regarded as irreversible. Glucose 6 phosphate formed is an important compound at the junction of several metabolic pathways like glycolysis, glycogenesis, glycogenolysis, glyconeogenesis, Hexosemonophosphate Shunt, uronic acid partway. Thus is a “committed step” in metabolic pathways. Enzymes of first steps: A. Hexokinase: In most tissues, the phosphorylation of glucose is catalyzed by hexokinase, one of three regulatory enzymes of glycolysis (see also phosphofructokinase and pyruvate kinase). 1. Hexokinase has broad substrate specificity and is able to phosphorylate several hexoses in addition to glucose. 2. Hexokinase is inhibited by the reaction product, glucose 6-phosphate, which accumulates when further metabolism of this hexose phosphate is reduced. B. Glucokinase: In liver parenchymal cells and β cells of the pancreas, glucokinase (also called hexokinase D, or type IV) is the predominant enzyme responsible for the phosphorylation of glucose. Glucokinase differs from hexokinase in several important properties 1. It has a much higher Km, requiring a higher glucose concentration for half-saturation Thus, glucokinase functions only when the intracellular concentration of glucose in the hepatocyte is elevated, such as during the brief period following consumption of a carbohydrate-rich meal 2. Glucokinase has a high Vmax, allowing the liver to effectively remove the flood of glucose delivered by the portal blood. This prevents large amounts of glucose from entering the systemic circulation following a carbohydrate rich meal, and thus minimizes hyperglycemia during the absorptive period. 3. Regulation by fructose 6-phosphate and glucose: Glucokinase activity is not directly inhibited by glucose 6-phosphate as are the other hexokinases , but rather is indirectly inhibited by fructose 6-phosphate 11 Hexokinase deficiency is a rare disease where the predominant clinical effect is Nonspherocytic Hemolytic Anemia (NSHA). The reasons why this genetically determined molecular lesion has only this consequence at clinical level are still unknown. Approximately 20 cases of this condition have been described to date. Signs and symptoms of hexokinase deficiency are very similar to those of pyruvate kinase deficiency but anemia is generally more severe. Some affected individuals reportedly have had various abnormalities in addition to NSHA including multiple malformations, panmyelopathy, and latent diabetes. It can be caused by genetic changes in the HK1 gene and is inherited in an autosomal recessive manner. Glucokinase functions as the glucose sensor in the beta cell by controlling the rate of entry of glucose into the glycolytic pathway (glucose phosphorylation) and its subsequent metabolism. In the liver, glucokinase plays a key role in the ability to store glucose as glycogen, particularly in the postprandial state. Heterozygous mutations leading to partial deficiency of glucokinase are associated with MODY and Homozygous mutations resulting in complete deficiency of this enzyme lead to permanent neonatal diabetes mellitus. As predicted by the physiologic functions of glucokinase, the increase in plasma glucose concentrations in patients with this form of diabetes results from a combination of reduced glucose-induced insulin secretion from the pancreatic beta cell and reduced glycogen storage in the liver after glucose ingestion. GCK-MODY (MODY2) is caused by heterozygous mutations in the GCK gene, which encodes the enzyme glucokinase. Glucokinase is expressed primarily in the liver and in the pancreatic β-cell. In the liver, it is involved in glycogenesis in response to postprandial elevations in glucose. Impairment of enzymatic activity in the liver contributes to the mild hyperglycemia seen in GCK-MODY. In the pancreatic islet, glucokinase triggers a cascade of events that facilitates insulin secretion in response to rising serum glucose. In this way, it acts as the glucose sensor in the β-cell. In the presence of the mutated enzyme, glucose-stimulated insulin secretion occurs at a higher serum glucose level when compared to individuals with the wild-type enzyme. 12 GCK-MODY is one of the more common MODY subtypes, accounting for 30%–60% of all MODY cases, depending on the age of the population studied. It is more common in pediatric MODY populations, compared to adult populations. It affects as many as 1 in 1000 people. 2. Isomerization of glucose 6-phosphate The isomerization of glucose 6-phosphate to fructose 6-phosphate is catalyzed by phospho glucose isomerase. The reaction is readily reversible and is not a rate-limiting or regulated step. 3. Conversion of Fructose 6phosphate to Fructose 1, 6 bisphosphate Fructose-6-p is phosphorylated with ATP at 1- position catalyzed by the enzyme phosphofructokinase-1 to produce the symmetrical molecule fructose 1, 6 bisphosphate. Note: 1. reaction one is irreversible and rate limiting 2. One ATP is utilized 3. Phosphofruvctokinase I is the key enzyme in glycolysis that regulates the pathway. PFK-1 is controlled by the available concentrations of the substrates ATP and fructose 6-phosphate. 4. PFK-1 is regulated by fructose 2,6-bisphosphate. Phosphofructokinase II is an is enzyme which catalyzes the reaction to form fructose 2 ,6- bis phosphate. 13 Phosphofructokinase: Patients with PFK deficiency (glycogenosis type VII, Tarui disease) may have mild jaundice, reflecting excessive hemolysis. This is because the muscle-specific subunit of this tetrameric enzyme is also present in erythrocytes, which have partial PFK deficiency. A unique pathological feature of PFK deficiency is the presence in muscle of a small portion of the abnormal glycogen, which by histochemical analysis, is diastase-resistant, and by electron microscopy, has a finely granular and filamentous structure, similar to the poorly branched polyglucosan that accumulates in branching enzyme deficiency. The presence of polyglucosan is probably due to the increased concentration of glucose 6 phosphate (G6P) resulting from the enzyme defect. As G6P is a physiological activator of glycogen synthetase, its excess may keep an abnormally high proportion of glycogen synthetase in the active form, thus altering the delicate balance between synthetase and branching enzyme activities in favor of glycogen chain elongation. Direct evidence for the validity of this mechanism comes from the discovery that polyglucosan myopathy in horses is due to a gain-of-function mutation in glycogen synthetase. Muscle phosphofructokinase deficiency (Type VII, Tarui's Disease) Phosphofructokinase (PFK) deficiency is similar to myophosphorylase deficiency in its clinical presentation and diagnosis. This enzyme converts fructose-6 and fructose-1-phosphate to fructose- 1,6-diphosphate, with the defect thus blocking the metabolism of glycogen, glucose, and fructose. As with myophosphorylase deficiency, prolonged ischemic exercise in PFK may result in muscle necrosis and myoglobinuria. However, renal failure is not as common in PFK as in myophosphorylase. Because the enzyme defect also affects erythrocytes, some patients also exhibit increased hemolysis with jaundice. PFK is a tetrameric enzyme that is under the control of three structural genes (M, L, P), but only the M gene subunit is expressed in mature muscle, whereas erythrocytes express both the M and L subunits. The absence of anemia in PFK patients is related to the ability of erythrocytes to synthesize a functional enzyme with the L subunit. The defect in the M gene is transmitted as an autosomal trait on chromosome 14 4. Cleavage of fructose 1, 6-bisphosphate Aldolase A cleaves fructose 1,6-bisphosphateto dihydroxyacetone phosphate and glyceraldehyde 3-phosphate. The reaction is reversible and not regulated. Fructose-biphosphate aldolase (Aldolase A, ALDOA), is the predominant isoform of aldolase in skeletal muscle and erythrocytes encoded by the ALDOA gene on chromosome 16. Autosomal recessive mutations in ALDOA, are extremely rare and were originally reported to cause hemolytic anemia by impairing thermostability of the enzyme. Subsequent reports have identified ALDOA deficiency as a cause of recurrent episodes of rhabdomyolysis, usually precipitated by fever, not always associated with hemolytic anemia, designating the disease as glycogen storage disease type 12 (GSD12) 5. Isomerization of dihydroxyacetone phosphate: Triose phosphate isomerase inter converts dihydroxyacetone phosphate and glyceraldehyde 3- phosphate. Dihydroxyacetone phosphate must be isomerased to glyceraldehyde3-phosphate for further metabolism by the glycolytic pathway. This isomerization results in the net production of two molecules of glyceraldehyde 3-phosphate from the cleavage products of fructose 1,6- bisphosphate. 15