Membrane Transport PDF
Document Details
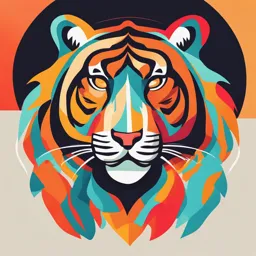
Uploaded by TrustyPigeon
Adamas University
Dr. Manoj Kumar Singh
Tags
Summary
This document provides an overview of membrane transport, discussing passive and active transport mechanisms and different types of transport proteins.
Full Transcript
1 MEMBRANE TRANSPORT Virtually any molecule will diffuse across a protein-free lipid bilayer down its concentration gradient. The rate of diffusion, however depends partly on the size of the molecule and mostly on its relative hydrophobicity (solubility in oil). Smaller, more h...
1 MEMBRANE TRANSPORT Virtually any molecule will diffuse across a protein-free lipid bilayer down its concentration gradient. The rate of diffusion, however depends partly on the size of the molecule and mostly on its relative hydrophobicity (solubility in oil). Smaller, more hydrophobic, or nonpolar molecules diffuse across a lipid bilayer more easily (such as O2 and CO2). Small uncharged polar molecules, such as water or urea, also diffuse across a bilayer, but much more slowly. Lipid bilayers are essentially impermeable to charged molecules (ions). Transporters and Channels Transporters and channels are the two major classes of membrane transport proteins. Transporters (also called carriers, or permeases) bind the specific solute to be transported and undergo a series of conformational changes that alternately expose solute-binding sites on one side of the membrane and then on the other to transfer the solute across it. Channels (eg. aquaporins), by contrast, interact with the solute to be transported much more weakly. They form continuous pores that extend across the lipid bilayer. When open, these pores allow specific solutes (such as inorganic ions of appropriate size and charge and in some cases small molecules, including water, glycerol, and ammonia) to pass through them. Transport through channels occurs at a much faster rate than transport mediated by transporters. Fig..Transporters and channel proteins. (A) A transporter alternates between two conformations, so that the solute-binding site is sequentially accessible on one side of the bilayer and then on the other. (B) In contrast, a channel protein forms a pore across the bilayer through which specific solutes can passively diffuse. Compiled by Dr. Manoj Kumar Singh (Asst. Prof., School of Life Science and biotechnology, Adamas University) Compiled from-Cell and Molecular Biology Concepts and Experiments, 6 th edition by Gerald Karp; Molecular Biology of the Cell, 6th Edition by Bruce Alberts; Molecular Cell Biology, 8th Edition by Harvey Lodish 2 Passive Transport Active Transport Passive transport down a concentration Active transport requires an input of gradient (or an electrochemical gradient) metabolic energy and is always mediated by occurs spontaneously, by diffusion, either transporters that pump the solute against its through the lipid bilayer directly or concentration or electrochemical gradient. through channels or passive transporters. Fig. B. The electrochemical gradient of a charged solute (an ion) affects its transport. This gradient combines the membrane potential and the concentration gradient of the solute. The electrical and chemical gradients can work additively to increase the driving force on an ion across the membrane (middle) or can work against each other (right). Compiled by Dr. Manoj Kumar Singh (Asst. Prof., School of Life Science and biotechnology, Adamas University) Compiled from-Cell and Molecular Biology Concepts and Experiments, 6 th edition by Gerald Karp; Molecular Biology of the Cell, 6th Edition by Bruce Alberts; Molecular Cell Biology, 8th Edition by Harvey Lodish 3 TRANSPORTERS AND ACTIVE MEMBRANE TRANSPORT The process by which a transporter transfers a solute molecule across the lipid bilayer resembles an enzyme–substrate reaction. By contrast to ordinary enzyme–substrate reactions, however, the transporter does not modify the transported solute but instead delivers it unchanged to the other side of the membrane. Fig. A model of how a conformational change in a transporter mediates the passive movement of a solute. The transporter is shown in three conformational states: in the outward open state, the binding sites for solute are exposed on the outside; in the occluded state, the same sites are not accessible from either side; and in the inward-open state, the sites are exposed on the inside. The transitions between the states occur randomly. They are completely reversible and do not depend on whether the solute binding site is occupied. Therefore, if the solute concentration is higher on the outside of the bilayer, more solute binds to the transporter in the outward-open conformation than in the inward-open conformation, and there is a net transport of solute down its concentration gradient (or, if the solute is an ion, down its electrochemical gradient). It requires only a relatively minor modification of the model shown in Figure above to link a transporter to a source of energy in order to pump a solute uphill against its electrochemical gradient. Cells carry out such active transport in three main ways : 1. Coupled transporters harness the energy stored in concentration gradients to couple the uphill transport of one solute across the membrane to the downhill transport of another. 2. ATP-driven pumps couple uphill transport to the hydrolysis of ATP. 3. Light- or redox-driven pumps, which are known in bacteria, archaea, mitochondria,and chloroplasts, couple uphill transport to an input of energy from light, as with bacteriorhodopsin, or from a redox reaction, as with cytochrome c oxidase. Compiled by Dr. Manoj Kumar Singh (Asst. Prof., School of Life Science and biotechnology, Adamas University) Compiled from-Cell and Molecular Biology Concepts and Experiments, 6 th edition by Gerald Karp; Molecular Biology of the Cell, 6th Edition by Bruce Alberts; Molecular Cell Biology, 8th Edition by Harvey Lodish 4 Fig. Three ways of driving active transport. The actively transported molecule is shown in orange, and the energy source is shown in red. ACTIVE TRANSPORT CAN BE DRIVEN BY ION-CONCENTRATION GRADIENTS There are three types of coupled transporters: Uniporters: They simply passively mediate the movement of a single solute from one side of the membrane to the other. Coupled transporters: in this case the transfer of one solute strictly depends on the transport of a second. They are further of two types: Symporters (also called co-transporters)- They drive the simultaneous transfer of a second solute in the same direction. Antiporters (also called exchangers)- They drive the transfer of a second solute in the opposite direction. Compiled by Dr. Manoj Kumar Singh (Asst. Prof., School of Life Science and biotechnology, Adamas University) Compiled from-Cell and Molecular Biology Concepts and Experiments, 6 th edition by Gerald Karp; Molecular Biology of the Cell, 6th Edition by Bruce Alberts; Molecular Cell Biology, 8th Edition by Harvey Lodish 5 Fig. Mechanism of glucose transport fueled by a Na+ gradient. As in the model shown above, the transporter alternates between inward-open and outward-open states via an occluded intermediate state. Binding of Na+ and glucose is cooperative—that is, the binding of either solute increases the protein’s affinity for the other. Since the Na+ concentration is much higher in the extracellular space than in the cytosol, glucose is more likely to bind to the transporter in the outward-facing state. The transition to the occluded state occurs only when both Na+ and glucose are bound; their precise interactions in the solute-binding sites slightly stabilize the occluded state and thereby make this transition energetically favorable. Stochastic fluctuations caused by thermal energy drive the transporter randomly into the inward-open or outward-open conformation. If it opens outwardly, nothing is achieved, and the process starts all over. However, whenever it opens inwardly, Na+ dissociates quickly in the low-Na+-concentration environment of the cytosol. Glucose dissociation is likewise enhanced when Na+ is lost, because of cooperativity in binding of the two solutes. The overall result is the net transport of both Na+ and glucose into the cell. Because the occluded state is not formed when only one of the solutes is bound, the transporter switches conformation only when it is fully occupied or fully empty, thereby assuring strict coupling of the transport of Na+ and glucose. The Na+ that enters the cell during coupled transport is subsequently pumped out by an ATP-driven Na+-K+ pump in the plasma membrane, which, by maintaining the Na+ gradient, indirectly drives the coupled transport. Such ion-driven coupled transporters as just described are said to mediate secondary active transport. In contrast, ATP-driven pumps are said to mediate primary active transport because in these the free energy of ATP hydrolysis is used to directly drive the transport of a solute against its concentration gradient. Compiled by Dr. Manoj Kumar Singh (Asst. Prof., School of Life Science and biotechnology, Adamas University) Compiled from-Cell and Molecular Biology Concepts and Experiments, 6 th edition by Gerald Karp; Molecular Biology of the Cell, 6th Edition by Bruce Alberts; Molecular Cell Biology, 8th Edition by Harvey Lodish 6 THREE CLASSES OF ATP-DRIVEN PUMPS ATP-driven pumps are often called transport ATPases because they hydrolyze ATP to ADP and phosphate and use the energy released to pump ions or other solutes across a membrane. There are three principal classes of ATP-driven pumps , and representatives of each are found in all prokaryotic and eukaryotic cells. 1. P-type pumps are structurally and functionally related multipass transmembrane proteins. They are called “P-type” because they phosphorylate themselves during the pumping cycle. This class includes many of the ion pumps that are responsible for setting up and maintaining gradients of Na+, K+, H+, and Ca2+ across cell membranes. 2. ABC transporters (ATP-Binding Cassette transporters) differ structurally from P-type ATPases and primarily pump small molecules across cell membranes. 3. V-type pumps are turbine-like protein machines, constructed from multiple different subunits. The V-type proton pump transfers H+ into organelles such as lysosomes, synaptic vesicles, and plant or yeast vacuoles (V = vacuolar), to acidify the interior of these organelles. Structurally related to the V-type pumps is a distinct family of F-type ATPases, more commonly called ATP synthases because they normally work in reverse: instead of using ATP hydrolysis to drive H+ transport, they use the H+ gradient across the membrane to drive the synthesis of ATP from ADP and phosphate. ATP synthases are found in the plasma membrane of bacteria, the inner membrane of mitochondria, and the thylakoid membrane of chloroplasts. Fig. Three types of ATP-driven pumps. Like any enzyme, all ATP-driven pumps can work in either direction, depending on the electrochemicalgradients of their solutes and the ATP/ADP ratio. When the ATP/ADP ratio is high, they hydrolyze ATP; when the ATP/ADP ratio is low, they can synthesize ATP. The F-type ATPase in mitochondria normally works in this “reverse” mode to make most of the cell’s ATP. Compiled by Dr. Manoj Kumar Singh (Asst. Prof., School of Life Science and biotechnology, Adamas University) Compiled from-Cell and Molecular Biology Concepts and Experiments, 6 th edition by Gerald Karp; Molecular Biology of the Cell, 6th Edition by Bruce Alberts; Molecular Cell Biology, 8th Edition by Harvey Lodish 7 P-TYPE ATPases Ca2+ Pump : it Pumps Ca2+ into the Sarcoplasmic Reticulum in Muscle Cells Eukaryotic cells maintain very low concentrations of free Ca2+ in their cytosol (~10–7 M) in the face of a very much higher extracellular Ca2+ concentration (~10–3 M). It is thus important that the cell maintains a steep Ca2+ gradient across its plasma membrane. Ca2+ transporters that actively pump Ca2+ out of the cell help maintain the gradient. One of these is a P-type Ca2+ ATPase; the other is an antiporter (called a Na+–Ca2+ exchanger) that is driven by the Na+ electrochemical gradient. Here we will discuss the Ca2+ ATPase, of the sarcoplasmic reticulum (SR) membrane of skeletal muscle cells. Fig. The structure of the sarcoplasmic reticulum Ca2+ pump. The three globular cytosolic domains of the pump—the nucleotide-binding domain (dark green), the activator domain (blue), and the phosphorylation domain (red),—change conformation dramatically during the pumping cycle. These changes in turn alter the arrangement of the transmembrane helices, which allows the Ca2+ to be released from its binding cavity into the SR lumen. Compiled by Dr. Manoj Kumar Singh (Asst. Prof., School of Life Science and biotechnology, Adamas University) Compiled from-Cell and Molecular Biology Concepts and Experiments, 6 th edition by Gerald Karp; Molecular Biology of the Cell, 6th Edition by Bruce Alberts; Molecular Cell Biology, 8th Edition by Harvey Lodish 8 Fig. The pumping cycle of the sarcoplasmic reticulum Ca2+ pump. Ion pumping proceeds by a series of stepwise conformational changes in which movements of the pump’s three cytosolic domains [the nucleotide-binding domain (N), the phosphorylation domain (P), and the activator domain (A)] are mechanically coupled to movements of the transmembrane α helices. Helix movement opens and closes passageways through which Ca2+ enters from the cytosol and binds to the two centrally located Ca2+ binding sites. The two Ca2+ then exit into the SR lumen and are replaced by two H+, which are transported in the opposite direction. The Ca2+-dependent phosphorylation and H+-dependent dephosphorylation of aspartic acid are universally conserved steps in the reaction cycle of all P-type pumps: they cause the conformational transitions to occur in an orderly manner, enabling the proteins to do useful work. (Adapted from C. Toyoshima et al., Nature 432:361–368, 2004 and J.V. Møller et al., Q. Rev. Biophys. 43:501–566, 2010.) Compiled by Dr. Manoj Kumar Singh (Asst. Prof., School of Life Science and biotechnology, Adamas University) Compiled from-Cell and Molecular Biology Concepts and Experiments, 6 th edition by Gerald Karp; Molecular Biology of the Cell, 6th Edition by Bruce Alberts; Molecular Cell Biology, 8th Edition by Harvey Lodish 9 The Na_/K_-ATPase. (a) Simplified schematic model of the transport cycle. Sodium ions bind to the protein on the inside of the membrane. ATP is hydrolyzed, and the phosphate is transferred to the protein Conformation of the transport protein is changed This allows the sodium ions to be expelled to the external space.Potassium ions then bind to the protein The phosphate group is subsequently lost This causes the protein to snap back to its original conformation, allowing the potassium ions to diffuse into the cell. Compiled by Dr. Manoj Kumar Singh (Asst. Prof., School of Life Science and biotechnology, Adamas University) Compiled from-Cell and Molecular Biology Concepts and Experiments, 6 th edition by Gerald Karp; Molecular Biology of the Cell, 6th Edition by Bruce Alberts; Molecular Cell Biology, 8th Edition by Harvey Lodish 10 ABC TRANSPORTERS ABC transporters (Largest Family of Membrane), are so named because each member contains two highly conserved ATPase domains, or ATP-Binding “Cassettes,” on the cytosolic side of the membrane. ATP binding brings together the two ATPase domains, and ATP hydrolysis leads to their dissociation. Fig. Small-molecule transport by typical ABC transporters. ABC transporters consist of multiple domains. Both importing and exporting ABC transporters are found in bacteria;(A) an ABC importer is shown in this cartoon. (B) In eukaryotes, most ABC transporters export substances—either from the cytosol to the extracellular space or from the cytosol to a membrane-bound intracellular compartment such as the endoplasmic reticulum—or from the mitochondrial matrix to the cytosol. Compiled by Dr. Manoj Kumar Singh (Asst. Prof., School of Life Science and biotechnology, Adamas University) Compiled from-Cell and Molecular Biology Concepts and Experiments, 6 th edition by Gerald Karp; Molecular Biology of the Cell, 6th Edition by Bruce Alberts; Molecular Cell Biology, 8th Edition by Harvey Lodish 11 TYPICAL STRUCTURE OF ABC TRANSPORTERS Typically, two hydrophobic domains, each built of six membrane-spanning αhelices, together form the translocation pathway and provide substrate specificity. Two ATPase domains protrude into the cytosol. In some cases, the two halves of the transporter are formed by a single polypeptide, whereas in other cases they are formed by two or more separate polypeptides that assemble into a similar structure. Without ATP bound, the transporter exposes a substrate-binding site on one side of the membrane. ATP binding induces a conformational change that exposes the substrate-binding site on the opposite side; ATP hydrolysis followed by ADP dissociation returns the transporter to its original conformation. Most individual ABC transporters are unidirectional. Compiled by Dr. Manoj Kumar Singh (Asst. Prof., School of Life Science and biotechnology, Adamas University) Compiled from-Cell and Molecular Biology Concepts and Experiments, 6 th edition by Gerald Karp; Molecular Biology of the Cell, 6th Edition by Bruce Alberts; Molecular Cell Biology, 8th Edition by Harvey Lodish 12 Ion Channels Are Ion-Selective And Fluctuate Between Open And Closed States Most ion channels are highly selective in allowing only one particular type of ion to pass through the pore. Most of the ion channels that have been identified can exist in either an open or a closed conformation; such channels are said to be gated. The opening and closing of the gates are subject to complex physiologic regulation and can be induced by a variety of factors depending on the particular channel. Three major categories of gated channels are distinguished: 1. Voltage-gated channels whose conformational state depends on the difference in ionic charge on the two sides of the membrane. 2. Ligand-gated channels whose conformational state depends on the binding of a specific molecule (the ligand), which is usually not the solute that passes through the channel. Some ligand-gated channels are opened (or closed) following the binding of a molecule to the outer surface of the channel; others are opened (or closed) following the binding of a ligand to the inner surface of the channel. For example, neurotransmitters, such as acetylcholine, act on the outer surface of certain cation channels, while cyclic nucleotides, such as cAMP, act on the inner surface of certain calcium ion channels. 3. Mechano-gated channels whose conformational state depends on mechanical forces (e.g., stretch tension) that are applied to the membrane. Members of one family of cation channels, for example, are opened by the movements of stereocilia on the hair cells of the inner ear in response to sound or motions of the head. Fig. The gating of ion channels. This schematic drawing shows several kinds of stimuli that open ion channels. Compiled by Dr. Manoj Kumar Singh (Asst. Prof., School of Life Science and biotechnology, Adamas University) Compiled from-Cell and Molecular Biology Concepts and Experiments, 6 th edition by Gerald Karp; Molecular Biology of the Cell, 6th Edition by Bruce Alberts; Molecular Cell Biology, 8th Edition by Harvey Lodish