Membrane Biochemistry Lecture 3 (Bio-4117) PDF
Document Details
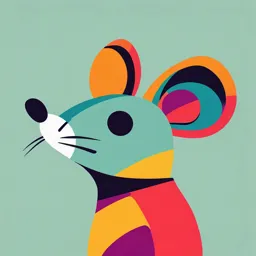
Uploaded by IntimateParody7065
Badr University in Cairo
Dr. Mariam Osama
Tags
Related
- Membrane Biochemistry Lecture 14 (Winter 2024)
- Membrane Biochemistry Lecture 10 - Transport Across Biological Membranes I - Winter 2024 PDF
- Membrane Biochemistry Lecture 10 - Transport Across Biological Membranes I
- Lecture 7: Cell surface Oligosaccharides I (March 13) - Membrane Biochemistry 2024 PDF
- Membrane Biochemistry Lecture 1 (Bio-4117) PDF
- Lec 3 Cell Membrane Biochemistry - Feb 24, 2024 PDF
Summary
These lecture notes cover membrane biochemistry, including details on insulin action, mechanisms, and its role. The lecture is specifically designed for a level 4 course, likely within a biological or health science program at BADR UNIVERSITY IN CAIRO
Full Transcript
Membrane Biochemistry (Bio-4117) lecture-3 level-4 Dr. Mariam Osama [email protected] Office: 236 Biotechnology Office Hours: Wednesday 2 : 4 Mechanism of Action of Insulin Outline… Introduction to Insulin History Structure of Insulin Biosynt...
Membrane Biochemistry (Bio-4117) lecture-3 level-4 Dr. Mariam Osama [email protected] Office: 236 Biotechnology Office Hours: Wednesday 2 : 4 Mechanism of Action of Insulin Outline… Introduction to Insulin History Structure of Insulin Biosynthesis of Insulin Degradation of Insulin Regulation of secretion Biological actions ❑ Effect on Carbohydrate, lipid, and protein metabolism Insulin Receptor Mechanism of action Insulin signaling pathways Disorders Introduction ▪ Insulin is a peptide hormone secreted by β-cells in the pancreatic islets of Langerhans. ▪ The main function of insulin is to lower serum glucose and promote anabolism. ▪ Insulin is an essential growth factor required for normal development. ▪ In 1869, a German medical student, Paul Langerhans , noted that the pancreas contains two distinct groups of cells the acinar cells, which secrete digestive enzymes, and cells that are clustered in islands, or islets, which he suggested served a second function. ▪ In 1889, Oskar Minkowski and Joseph von Mering observed that total pancreatectomy in experimental animals leads to the development of severe diabetes mellitus In 1920, Frederick Banting managed to isolate the fluid extract-that he called ‘isletin’- from Islets of Langerhans and injected it into diabetic dogs. The dogs abnormally high sugar levels in the blood lowered and they survived for as long as they received the extract. The Nobel Prize in Medicine and Physiology was awarded to Banting and Macleod with in 1923. Insulin is sequenced by British biochemist Frederick Sanger and is the first protein to be fully sequenced. In 1958 Sanger received the Nobel Prize in Chemistry. Dorothy Hodgkin won the 1964 Nobel Prize in Chemistry for her work in protein crystallography/ x-ray crystallography. 1978, Biotechnology firm Genentech (Califor.) used rDNA technology to produce synthetic “human” insulin- first human protein to be manufactured through biotechnology. Insulin should have been named “Protein of the 20th century” Insulin was: ❑ the first protein shown to have hormonal action ❑ the first protein to be crystallized in pure form (Abel, 1926) ❑ the first protein to be fully sequenced (Sanger et al, 1955) ❑ the first protein to be synthesized chemically (Du et al, Zahn, 1964) ❑ the first protein to be synthesized as a large precursor molecule (Steiner et al, 1967) ❑ the first protein synthesized for commercial use by DNA recombinant technology (1979) Structure of Insulin ▪ - Human insulin- 51 aa ▪ Mw; 5.7 kDa ▪ Comprises of 2 polypeptide chains I. A (with 21 amino acid residues) and II. B (with 30 amino acid residues) ▪ The two chains are linked by two interchain disulfide bridges that connect A7 to B7 and A20 to B19 ▪ A third intrachain disulfide bridge connects residues 6 and 11 of A chain 1. Insulin Structure and Forms Insulin exists as a single molecule (monomer) when the concentration is low (around 10⁻⁶ M). At higher concentrations, two insulin molecules bind together to form a dimer through hydrogen bonding, specifically between the C-terminal of the B chains. At even higher concentrations, especially in the presence of zinc ions, insulin molecules aggregate further to form hexamers (6 insulin molecules bound together). 2. Diffusion and Absorption Monomers and dimers can easily diffuse into the blood, making them fast-acting. Hexamers are large and diffuse poorly into the bloodstream, which delays absorption. This leads to a slower absorption rate, which is useful when the body needs to control blood sugar over an extended period of time. By forming dimers and hexamers, insulin becomes more stable and can be stored in dense, compact forms without degrading. When insulin is released into the bloodstream, hexamers break down into dimers and monomers. 3. Conserved Regions in Insulin Three important regions of the insulin molecule are conserved across different forms and are involved in interacting with the insulin receptor: N-terminal of the A-chain: GlyA1-IleA2-ValA3-GluA4 (or AspA4). C-terminal of the A-chain: TyrA19-CysA20-AsnA21. C-terminal of the B-chain: GlyB23-PheB24-PheB25-TyrB26. These regions are located near the surface of insulin, allowing them to interact with the insulin receptor. 4. Insulin Lispro (Recombinant Analog) Insulin lispro is a modified form of insulin that was created to reduce the formation of dimers and hexamers. In insulin lispro, the lysine and proline residues at the C-terminal of the B chain are reversed. This change does not affect the ability of insulin to bind to its receptor, but it makes the insulin molecules less likely to form dimers and hexamers, allowing for faster absorption into the blood. Biosynthesis of Insulin – 3 major steps ❑ Site; β- cells of Islets of Langerhan’s 1. Synthesis of Preproinsulin. 2. Conversion of preproinsulin to proinsulin. 3. Conversion of proinsulin to insulin. Insulin is synthesized by ribosomes of the rough ER as a larger precursor peptide that is then converted to the mature hormone prior to secretion ▪ Insulin is synthesized in the form of a precursor, preproinsulin (110 aa), processed via an intermediate precursor, proinsulin (86 aa) , to the mature insulin (51 aa) molecule Biosynthesis of Insulin contd….. Conversion of proinsulin to insulin Proinsulin undergoes maturation into active insulin through action of cellular endopeptidases known as prohormone convertases (PC1 and PC2), and the exoprotease carboxypeptidase E The endopeptidases cleave at 2 positions, releasing a fragment called the C-peptide, and leaving 2 peptide chains, the B- and A- chains, linked by 2 disulfide bonds ▪In the β cells, insulin combines with zinc to form complexes ▪In this form, insulin is stored in granules of the cytosol which is released in response to various stimuli. Regulation of insulin secretion by glucose in pancreatic β cells. Like other neuroendocrine cells, insulin-secreting cells are electrically excitable. This means that insulin secretion in response to glucose is dependent on the initiation of electrical activity from a basal electronegative resting state. In the normal course of glucose excitation coupling to insulin secretion, glucose enters the cell via GLUT2 (and GLUT1) glucose transporters and is trapped in the cell by phosphorylation via glucokinase, a specialized high Km hexokinase. The high Km of glucokinase for glucose underlies its role as the “glucose sensor” of the β cell, in that its enzyme activity reflects physiologic circulating glucose concentrations. (High 𝐾𝑚 of glucokinase means it doesn't bind to glucose very strongly unless glucose levels are high, which ensures that insulin is released only when there's a lot of glucose in the blood (after meals). Hexokinase in skeletal muscle cells has a high affinity for glucose, allowing it to function efficiently even at low glucose levels, and is inhibited by its product, glucose-6-phosphate, ensuring tight regulation of glucose use. In contrast, glucokinase in pancreatic beta cells has a low affinity for glucose, requiring higher glucose concentrations to activate, and it is not inhibited by glucose-6-phosphate. This makes glucokinase a key glucose sensor that triggers insulin release in response to elevated blood sugar levels, playing a crucial role in blood sugar regulation. ▪Following glucose phosphorylation, further metabolism via glycolysis, the Krebs (tricarboxylic acid, TCA) cycle, and oxidative phosphorylation in the mitochondria leads to an increase in the ATP-to-ADP ratio in the cytoplasm. ▪The ATP/ADP ratio increase is driven by metabolism that leads to ATP- dependent potassium channel (KATP) channel inhibition, in turn leading to the accumulation of positive charge (K+ and Na+) inside the cell and causing the cell membrane to depolarize. ▪As the membrane potential reaches about –20mV from the resting level of about –70mV, voltage-dependent calcium channels open, allowing entry of Ca2+. This rise in calcium inside the cell is a key signal for insulin release. Microdomains and Insulin Release: The rise in calcium is carefully controlled in specific small areas near the cell membrane, called "microdomains." These areas are where insulin granules get ready to fuse with the membrane and release insulin into the bloodstream. Insulin Release Mechanism: When calcium levels rise inside the cell, they activate various proteins, including small proteins (like Rabs) and SNARE proteins. These proteins work together to help the insulin granules fuse with the cell membrane and release insulin. Glucose ;key regulator (aa, ketones, various nutrients, GIPs, and NTs also influence insulin secretion.) Increased ATP closes the ATP-sensitive K+ channels K+ efflux Depolarizes the cell membrane Open voltage-sensitive calcium channels Calcium enters the cell Intracellular Ca 2+ Triggers insulin secretion by exocytosis Glucose levels > 3.9 mmol/L (70 mg/dL) stimulate insulin synthesis, primarily by enhancing protein translation and processing Degradation of Insulin 1. Insulin Half-Life: 1. Insulin, once released into the bloodstream, has a short half-life of about 4 to 6 minutes, meaning that it doesn’t stay active in the body for long before being broken down. 2. Liver as the Principal Site of Insulin Degradation: 1. The liver is the primary organ responsible for degrading insulin. 3. Hepatic Glutathione Insulin Transhydrogenase: 1. In the liver, an enzyme called hepatic glutathione insulin transhydrogenase works by reducing the disulfide bonds that hold the two chains of the insulin molecule (A-chain and B-chain) together. 2. Once these bonds are broken, the individual A and B chains of insulin are separated and quickly degraded by other enzymes. 4. Insulin-Degrading Enzyme (IDE): 1. Another important enzyme involved in insulin breakdown is the insulin- degrading enzyme (IDE). 2. IDE specifically cleaves the B-chain of insulin at the bond between two amino acids: Tyrosine (Tyr) at position 16 and Leucine (Leu) at position 17. This helps further degrade insulin into smaller fragments. Metabolic effects of insulin ▪Insulin promotes the anabolic state by channeling metabolism towards the storage of carbohydrates and lipids, and towards protein synthesis. ▪Insulin promotes energy storage by stimulating the synthesis of fatty acids and triglycerides in the liver and adipose tissue, glycogen in liver and skeletal muscle, and protein synthesis in muscles. ▪At the same time, it opposes the catabolism by inhibiting the breakdown of proteins, triglycerides and glycogen, and by suppressing gluconeogenesis by the liver. Different organs and tissues handle fuels differently ▪At rest, the brain uses approximately 20% of all oxygen consumed by the body. ▪Glucose is normally its only fuel: during starvation, however, the brain adapts to the use of ketone bodies as an alternative energy source. ▪The two pathways that provide glucose are glycogenolysis and gluconeogenesis. ▪When the glucose concentration in the extracellular fluid decreases, it is first replenished by degrading liver glycogen (glycogenolysis). ▪However, when the fasting period extends gluconeogenesis is initiated. Gluconeogenesis takes place mostly in the liver, and the kidneys also contribute during prolonged fasting. Its main substrates are lactate (from anaerobic glycolysis), alanine (from the amino acids released during the breakdown of muscle protein), and glycerol (from the breakdown of triacylglycerols in the adipose tissue. Insulin Action on Carbohydrate Metabolism: Liver: ▪ Stimulates glycolysis ▪ Promotes glucose storage as glycogen ▪ Inhibits glycogenolysis ▪ Inhibits gluconeogenesis Muscle: ▪ Stimulates glucose uptake (GLUT4) ▪ Promotes glucose storage as glycogen Adipose Tissue: ▪ Stimulates glucose transport into adipocytes ▪ Promotes the conversion of glucose into triglycerides and fatty acids INSULIN RECEPTOR AND MECHANISM OF SIGNALING Introduction: Insulin Insulin is a peptide hormone produced by β-cells of the pancreas in response to nutritional stimuli. Main sites of action of Insulin in the body include Liver, Adipose Tissue, Muscles & Brain. Insulin is secreted by β-cells in response to an increase in Blood glucose levels. Insulin binds to the insulin receptor and facilitates uptake of glucose by the cell. Insulin’s interaction with the cell triggers both metabolic and mitogenic cellular responses. Insulin-like Growth Factor, IGF-1 is a mediator of cell growth and differentiation. Introduction: Insulin Receptor Insulin receptor belongs to a family of receptor tyrosine kinases (RTKs), which phosphorylate their substrate proteins on tyrosine residues. The insulin receptor comprises of two subunits: the extracellular α-subunit and the transmembrane β-subunit. The functional receptor exists as a dimer/heterotetrameric complex: α2β2 The α-subunit contains the ligand binding site, as it is the only subunit identified by affinity labeling protocols. The β subunit is involved in intracellular signaling. ~ Tyrosine kinase activity Insulin Receptor When insulin interacts with the receptor, dimerization of the receptor takes place. Due to this, the cytoplasmic domains of the receptor come close together facilitating autophosphorylation and triggering the signal transduction pathway. Types of Signaling Mechanisms Insulin's interaction with its cell surface receptor triggers both metabolic and mitogenic cellular responses. To do this, Insulin employs two kinds of pathways: Ras-dependant and Ras-independent. Ras proteins are small GTP-binding proteins that act as molecular switches, controlling cell growth, division, and survival. They toggle between an active state when bound to GTP and an inactive state when bound to GDP. Ras proteins transmit signals from cell surface receptors to regulate key cellular processes, and mutations in Ras are often associated with cancer due to uncontrolled cell growth. Ras-dependant Pathway: Upon insulin binding, the Ras protein is phosphorylated leading to activation of Mitogen Activated Protein Kinase (MAPK) pathway which is involved in cellular growth and proliferation. Ras-independent Pathway: In this case, another pathway known as the PI3K/AKT signaling pathway is activated by a series of events facilitating glucose uptake by the cell. Ras-Independent Pathway: PI-3K/AKT Signaling Role of Proteins Involved in PI-3K/AKT Signaling Insulin & Insulin Receptor: When blood glucose levels rise, insulin released by the pancreatic beta cells binds to the Insulin Receptor on muscle cells and/or adipocytes, facilitating its dimerization. As a result of this dimerization, the receptor is autophosphorylated at specific residues. IRS: Insulin receptor substrate family are proteins that are primarily activated as a result of autophosphorylation. The Ser/Thr residues of these proteins are known to be phosphorylated. There are two types of IRS: IRS-1 and IRS-2. IRS-1 is known to function in glucose metabolism, while IRS-2 functions in lipid metabolism. However, both of these are recruited by the insulin receptor as well as IGF-R (insulin-like growth factor receptor) to exert different kinds of effects. PI-3 Kinase: Phosphatidylinositol kinase is activated by IRS-1 and acts as an adaptor protein. Activated PI-3Ks serve to phosphorylate phosphatidyl inositol (a lipid molecule) on the D3 position of the inositol ring generating PtdIns-3,4,5-P3 (phosphatidylinositol-3,4,5-triphosphate). These specialized lipids recruit PH-domain- containing proteins (Pleckstrin Homology domain) such as Akt (PKB) to the plasma membrane. Protein Kinase B or Akt: Akt helps in translocation of GLUT-4 storage vesicles to the plasma membrane by a series of events leading to the activation of Rab protein. As GLUT-4 vesicles fuse with the plasma membrane, no. of receptors increase thereby increasing glucose uptake. Also known as AKT Proteins involved in PI-3K/AKT Pathway PI-3K IRS-1 AKT Insulin binding to the receptor leads to a conformational change that induces autophosphorylation, similar to the activation of other RTKs. After IRS1 binds to a phosphotyrosine residue through a PTB domain, the activated kinase in the receptor’s cytosolic domain phosphorylates IRS1. One subunit of PI-3 kinase binds to the receptor-bound IRS1 via its SH2 domain, and the other subunit then phosphorylates phosphatidyl inositol generating PtdIns-3,4,5-P3. AKT The phosphoinositides bind the PH domain of protein kinase B (PKB)/Akt, thereby recruiting it to the membrane. Two membrane-bound kinases, in turn, phosphorylate membrane-associated PKB (AKT) and activate it. Activated PKB is released from the membrane and promotes glucose uptake by the GLUT4 transporter and glycogen synthesis. The former effect results from the translocation of the GLUT4 glucose transporter from intracellular vesicles to the plasma membrane. The latter effect occurs by PKB-catalyzed phosphorylation of glycogen synthase kinase 3 (GSK3), converting it from its active to inactive form. As a result, GSK3-mediated inhibition of glycogen synthase is relieved, promoting glycogen synthesis. GLUT-4 Translocation: A key step 1. GLUT4 Translocation: In the basal state, GLUT4 is mostly stored in intracellular vesicles. Insulin triggers GLUT4 to move to the cell surface, increasing glucose uptake. 2. Key Mediators: PI-3K, PtdIns-3,4,5-P3, Akt, and Rab proteins are crucial for the insulin-stimulated GLUT4 translocation. These findings emphasize the importance of the PI-3K/Akt pathway in GLUT4 translocation and glucose uptake in response to insulin. Ras-dependent Pathway: Shc/RAS/MAPK Signaling The pathway 1. Insulin Binding: Insulin binds to its receptor on the cell surface, which is a receptor tyrosine kinase (RTK). This receptor becomes activated and phosphorylated. 2. Activation of Shc Protein: Once the insulin receptor is activated, it phosphorylates the Shc protein, a key adaptor protein in this pathway. 3. RAS Activation: Phosphorylated Shc interacts with another protein called Grb2, which in turn activates Sos, a protein that facilitates the exchange of GDP for GTP on RAS, a small GTPase. This process activates RAS, which switches from an inactive (GDP-bound) state to an active (GTP-bound) state. 4. Activation of MAPK Cascade: Once RAS is activated, it triggers a cascade of protein activations: RAS activates Raf, a kinase. Raf activates MEK. MEK activates MAPK (Mitogen-Activated Protein Kinase), also known as ERK (Extracellular signal-regulated Kinase). 5. Cellular Responses: MAPK/ERK enters the nucleus and activates specific transcription factors that regulate gene expression. This signaling leads to important cellular responses such as cell growth, division, and survival. Regulation of Insulin signaling Insulin receptor (IR) signaling is regulated: 1. Dephosphorylation by Protein Tyrosine Phosphatases (PTPases): 1. PTPases are enzymes that remove phosphate groups from the insulin receptor (IR) after it has been activated by insulin. This dephosphorylation stops the insulin signal but does not degrade the receptor, allowing it to be reused. 2. Regulation of Cell-Surface IR Density: The number of insulin receptors on the cell surface is controlled by: 1. Addition from the Golgi apparatus, which supplies new IRs to the cell surface. 2. Insulin-stimulated IR endocytosis, where insulin binding triggers the receptor to be pulled into the cell, reducing the number of IRs on the surface and turning off the signal. 3. IR Serine Phosphorylation: 1. Phosphorylation of the insulin receptor on serine residues (as opposed to tyrosine) leads to negative regulation, meaning it reduces the receptor’s activity and slows down insulin signaling. Together, these mechanisms ensure that insulin signaling is tightly controlled and can be switched off when necessary. Diabetes Mellitus ▪ The word ‘diabetes’ -derived from a Greek word, meaning to siphon and refers to the marked loss of water by urination, polyuria. ▪ The word ‘mellitus’ derived from the Latin means sweet and thus diabetes mellitus is known as sweet urine disease. ▪ Group of metabolic diseases characterized by increased levels of glucose in the blood (hyperglycemia) resulting from defects in insulin secretion, insulin action, or both Person has high blood sugar. ▪This high blood sugar produces the classical symptoms of ▪Glycosuria, ▪Polyuria (frequent urination), ▪Polydipsia (increased thirst) and ▪Polyphagia (increased hunger). Diabetes mellitus is classified into four main categories: 1. Type 1 Diabetes: An autoimmune condition where the body's immune system attacks insulin-producing beta cells in the pancreas, leading to little or no insulin production. 2. Type 2 Diabetes: A condition where the body becomes resistant to insulin or doesn’t produce enough insulin, often associated with lifestyle factors such as obesity and inactivity. 3. Gestational Diabetes: Diabetes that develops during pregnancy and usually resolves after childbirth, though it increases the risk of developing Type 2 diabetes later. 4. Impaired Glucose Tolerance (Pre-Diabetes): A state where blood glucose levels are higher than normal but not high enough to be classified as diabetes, indicating a higher risk of developing Type 2 diabetes. Increased Insulin Level Insulinoma ▪ Adenoma of islets of langerhans ▪ Increased insulin secretion ▪ Features :neuropsychiatric symptoms, nervousness, confusion and hypoglycemic attacks Insulin shock High level of insulin. Fall in blood glucose level. CNS depression. 50-70 mg/dl CNS excitability 20-50 mg/dl CONVULSION & COMA < 20 mg/dl COMA What we have learnt…