MEDI214 Lecture 12 Transcript PDF
Document Details
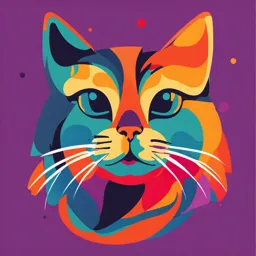
Uploaded by LavishInequality
Tags
Summary
This document is a lecture transcript on energy systems, relating exercise physiology to nutrition. The transcript covers the connection between food energy, movement, heat production, and homeostasis in the body.
Full Transcript
SPEAKER 0 Um this is mhm Here just you know. Yeah, we gonna we gonna get back something good. Yeah. Right. Is that right? What? SPEAKER 1 Alright guys, we'll make a start what we're going to have a look at today is um the energy systems this could easily slide in or slide into a puristic excise...
SPEAKER 0 Um this is mhm Here just you know. Yeah, we gonna we gonna get back something good. Yeah. Right. Is that right? What? SPEAKER 1 Alright guys, we'll make a start what we're going to have a look at today is um the energy systems this could easily slide in or slide into a puristic excise physiology subject. So a lot of the content is somewhat similar but what we want to do is take a little bit of a stance around how does this relate to nutrition? Cos it's pretty obvious, isn't it that particularly when we talk about the aerobic system? Um there's a very close link to what we consume in terms of our dietary intake. So the topic itself is an exercise physiology topic, but we'll take a little bit of a slant on how does this relate to nutrition? And then obviously the subject that towards nutrition? Ok. So if we have a look at this scenario, so if we think about then the connection between the food that we take in its energy and that energy is obviously converted into mechanical energy we move, but equally we produce heat. So it's a fairly complex reaction that occurs, isn't it? Ok. So we take that food in contains energy, we then use it to move, we use it to create homeostasis. So our cells can stay alive. But we also produce heat. And we know that cos we can feel it ourselves that we're actually producing heat all the time. And squitter muscles are a major component of that. What we also know is that inadequate food intake can lead to a lack of fuel and substrates. That means that the body doesn't have enough energy required to maintain homeostasis for the cell or in the aspect of sports, nutrition. If you think about it, there's not enough energy required or the components of energy required for the amount of intensity or duration that we're taking part in physical activity to sustain that physical activity. So we have levels of fatigue that become evident. So the question is, have you seen your own, in your own physical capacities or have you witnessed examples such as sport or physical activity where the activity is in excess of the energy supply in sort of a really raw way of thinking about it. And therefore, the activity started to look fairly like I've got on screen, they're hampered or it looks like it's starting to be um deteriorated. Has anyone got any clues or examples? Obviously, we've got the Olympics starting in the last 48 hours as well. And there's often some really good scenes around Olympians trying to push themselves to the, the nth degree. Has any, anyone got some clues on where you've seen that before? Yep. Marathon's a classic, isn't it? Cos you're lasting a couple of hours, um, combination of fuel, but also the hydration status is important as well. Any other examples. So it's very much long duration type or getting towards long duration. Does it only have to be with long duration? Yep. Yep. So, so maybe there's some balanced situation around ions, for example, and then there's also the exacerbation through to dehydration that might lead to cramping. Ok. So there's lots of examples that we can think about that you can see on screen, they involve quite dramatic type scenes sometimes. So right up there, towards the left there, you'll notice the Brownley Brothers, that's a classic one where one of them literally gets within 30 m of the line and just starts to meltdown. Ok. So it is a combination of neuromuscular and substrate availability can affect the brain as well and you can get, you can get collapse. Um but collapse can relate to then hydration status as well. So you can see there's uh this was com games, this one here. Ok. So with the walking Com games up at um the Gold Coast um and obviously the combination of dehydration with heat exhaustion because the cardiovascular system has to be able to pump blood around the body to get rid of heat means that you can end up with cardiovascular insufficiency and people actually sort of their blood pressure falls and they collapse. In this instance, there's classics around the Tour de France as well that we've seen. Ok. So it's really evident when someone's not meeting the energy requirements and there is a challenge to the homeostatic level of the body and that can result in collapse is the best way of putting it. Alright. So without reading all these out, what we're going to look at today is the energy systems, which is really sort of the top three or four there. Then as we get down to number five and six, we're looking at how that relates to carbohydrates, fats and proteins. It's, it's a tricky topic because there's obviously biochemistry involved with this. So we're gonna try and sort of tailor this to the idea that we want to look at from an unapplied point of view, as opposed to a biochemistry point of view, I'm gonna then take you through to what does this mean in terms of exercise, intensity and duration um which brings in components such as oxygen consumption. Ok. So when we consume oxygen, particularly over longer durations, that's got an indirect relationship to our energy expenditure and heat production. And so being able to look at oxygen consumption and say, is it flat as in doesn't seem to be rising or is it actually increasing? Can give us a feel about the intensity of which those sub traits are, are being uh required for the contracting skeletal muscle, for example. And so we'll, we'll introduce this concept called the oxygen slow component. And you've probably experienced that where you think you're exercising in a fairly steady state. But things seem to start to increase your heart rate is getting a little bit high. Your breathing's getting a little bit deeper, it's called the oxygen slow component. We'll try and explain what that means and what that means in terms of how diet can interrelate with the oxygen slow component as, as a relationship to that. Alright. So let's go with a little bit of revision to start with what you see on screen. There is a classic chemical reaction. We've got a TP being broken down to AD P plus phosphate, adenosine diphosphate. And we've got an enzyme sitting in the middle A TPAS means enzyme. OK. So in that instance, we can see that there's energy being liberated as part of that. So the question is the reaction glow, is it rep phosphorylation of A TP from AD P? Is it hydrolysis of A TP from AD P? Is it an endogen uh reaction or is it hydrolysis of A TP? Has anyone got, got a, so we've got repos relation of A TP, we're going this way and the reaction. Yeah, probably not. OK. So it's really hydrolysis. So in this instance, yeah, they go back and forth as a reaction. But in this instance, we can see our adenosine triphosphate is being broken with the use of the enzyme into AD P plus phosphate and the phosphates been blown off. And we've got energy release. And the reason why we've got energy, if you think about the triphosphate, the three phosphates actually line up one after the other. And the third one has to use as much energy as possible to hang on to the, the actual molecule. So if you break it off, you actually liberate the most amount of oxygen cos the question is, can we just break down AD P and become A and P? And the answer is yes, but you don't liberate as much oxygen co the second phosphate doesn't hold on with as much energy. And finally, when you get to the third one, which is really close, the hardware has any energy at all. So you get the most energy um release from releasing that third phosphate, which is used a lot of energy to hold on in the first place. OK. So that's your classic reaction. The question we're gonna work through today is how do we continue to re synthesize a TP in the face that there's only so much a TP that you can store in each cell. The classic I like to use is if you think about your heart cells, they have to use a TP under aerobic conditions, you've only got about eight seconds left of a TP left in your heart cells at any one time. So in other words, if you switch off oxygen, you've got eight seconds left before you go into arrhythmia. Ok. So that's how close you've got in terms of a TP supply. And therefore that's why it's really important for the heart to continue to supply oxygen through the coronary arteries. Ok? You can't keep storing a TP. The other example I like to use is if you were to store enough a TP in your skeletal muscles to run a marathon, you'd be carrying around about 80 to 90 kg backpack of a TP to run that marathon. OK. It's impossible, isn't it? Ok. So you have to be able to re synthesize it all the time. And so that's what we see that the primary example for re synthesis is on the basis that we're going to contract skeletal muscle. I remember there's a couple of different types of square muscle contraction. There's concentric or shortening contractions, there's isometric. So they don't change length and then there's centric. Now, what's interesting is each of those don't use the same amount of A TP. And the one that uses the most is the concentric, which is often what we engage in when we're looking at sort of whole body movements in physical activity and sport. What you can see there is quite clearly the A TP breaks down into AD P liberates the energy, the energy is then used to produce force. And if we think about force as one of those three types of contractions or a combination. And it makes sense that you've got to continue to supply a TP, just like the heart. You've got to supply a TP continuously to the skeletal muscle for it to go about continuing to contract. If you can't do that, that contraction force starts to decline over time. Alright. So in three fairly classic buckets, we like to think about the re synthesis of a TP based on a couple of different categories. So the first bucket is our cru and phosphate bucket. Ok. So that's the bucket that's associated with this high end ballistic type activity often. And I've got a question about this and often thought about as an anaerobic, we'll ask the question, is it truly anaerobic soon? But you can see that the cretin with the phosphate has a phosphate there ready to donate to a DB when required. And we can re already start to re synthesize that we've also got anaerobic glycolysis. Again, it's a quite a powerful engine associated with skeletal muscle contraction. It delivers a TP fairly quickly but doesn't deliver a huge amount of A TP. OK. So again, there's only so much anaerobic glycolysis that can occur the o oxidative phosphorylation. We're starting to move into the concept that we also need a combination of the fuel. It could be fats, it could be carbohydrates and also the supply of oxygen. Ok. So this is the one that the heart is obviously most uh wanting to use because again, the heart has to require AAA aerobic basis to its contraction. Cos otherwise, it starts to produce all the bros associated particularly with anaerobic glycolysis. And that's gonna be damaging to the heart cells. What's interesting about skeletal muscle is it's not quite as susceptible to damage compared to heart cells. So it can kind of operate across all three of these. Ok. Skeletal muscle is quite happy to be ischemic. Heart cells are not happy to be ischemic. So you can see already there, there's a difference in terms of the aerobic and anaerobic systems according to what type of skeletal muscle or cardio muscle cell is related to those. Now, the one thing I don't want you to think about, even though we've got three boxes on the screen, we don't just switch between these energy systems, ok? The energy systems are always operating and it's really important we start to understand the predominance of these under different conditions which makes energy expenditure tricky. Alright. So this is a classic study. It's actually done by some Australian physiologist, uh Mark Hargraves, uh who's down in Melbourne, but it's this idea trying to get away from the concept that if you're exercising ballistically for up to 30 seconds that somehow it's all anaerobic. Ok. So what you see on screen here is a graph that's a TP turnover, ok? On here against time. So the first thing you should look at that graph is the idea that the time is only up to 30 seconds. OK. So this is an activity of all out maximal activity. And you're asked to perform a wing test or run 300 m around the track roughly, you know, qu quite ballistically. OK. It's an all out effort over that time. So what you can see is that we've also got three color codes occurring here. We've got our light moderate and dark green. And what that's representing as we go through those colors is the relative contribution of those three buckets I had on the screen before, between the crute and phosphate, anaerobic glycolysis and the oxy ahoy relation. So what you'll notice over time is the A TP turnover obviously declines. Ok. So it's really quite quick here in the first six seconds. And, and notably, that's actually a lab that you, what you're gonna do in the lab today is you, you're gonna be asked to go all out for six seconds. So you can see that the turnover is the highest at that point in time. And you can see that even in six seconds, there's some aerobic based activity that's occurring in that six seconds. So it's not just purely cretin phosphate, but you'll notice the A TP turnover, it comes less and that makes sense. You can't run 400 m as quick as what you can run 100 m. But you'll also notice that at 30 seconds, there's now a predominance of aerobic metabolism occurring. Ok. So, even though we always think about it as an all out type sprinting activity, the reality is that a TP is actually coming from aerobic pathways and it's starting to also starting to predominate by the time you get out to 30 60 seconds. And that's the second test that we're gonna ask you to do later today is we're actually gonna ask you to cycle for 60 seconds and you can't get out of that without having huff and puff. Ok. The the aerobic metabolism is really evident after 60 seconds, it's a kind of equivalent for the average person running around a 400 m uh effort in that instance. Alright. So this is where it gets a little bit biochemistry complicated. So I'll just step through this slowly and it's really just take home messages. OK? So we're not asking you to reproduce the biochemistry of this. We're just asking you to consider the themes that are coming through, right? So what you see here is this is a cell membrane. So this is the blood that's on the outside, this is the cell membrane and this is the intracellular environment, the intracellular environment's made up of where you see the arrow there. It's the cytosol and then we have our mitochondria. Now, mitochondria is a double membrane system in this instance. Uh and it's deep with inside the cell and obviously, some cells have lots of mitochondria, like the heart cells and other cells have lower mitochondrial density like scal muscle cells. And then even within SCLE muscle cells, we have slow twitch and fast twitch. Right. So we've got our outside blood, we got our membrane cytosol and then the mitochondria. So what you'll notice is that from the blood where transporting oxygen? So let's pretend this is a skeletal muscle cell. So oxygen's coming through it and it has the potential to be absorbed into the cell itself. So you'll notice that the oxygen's heading deep inside where it can then play a role in terms of mitochondrial oxidative phosphorylation. So it's available. Hopefully it's available. But what you'll also notice is the key thing that we wanna do is have contraction of our key proteins. So that's our Acton and Mycin. So in red is the Mycin, it's the big heavy chain and the greens, the Acton, the idea is a sliding film that says that they're going to pull on each other and the muscle cells gonna shorten to do that, it requires energy. So you'll notice that sitting under there, there's a range of little activities going on, which involves cretin phosphate involves a re synthesis of A TP in this instance and then the breakdown to AD P plus phosphate. OK. So that's sitting close by and we have um the energy that's being used and then rebuilt all the time. So how do. We do that. Obviously, we can have a couple of different pathways. We can feed fats, we can feed carbohydrates through the cell using transporters. And what you'll notice is they go inside the cell and then they're available to be broken down. If we start with glucose, you can see that glucose can either come from within the blood or stored muscle glycogen. And it can be broken down to this little chemical called pyrite. If there's not a lot of oxygen available over here, that can be converted into lactate. Ok. So if there is a low level of oxygen available in the cell, we can obviously use anaerobic pathways to produce lactate. In that instance, if there is oxygen available, pyruvate gets converted into cyl coa and that enters what we call the citric acid cycle or the creb cycle. Again, we don't want to go into a huge amount of detail. But what you'll notice in this cycle is we produce these little electron transporters called N AD and N A DH. And they're providing h positive ions to the electron transport chain. The electron transport chain can receive oxygen and we can re synthesize A TP. So that's our aerobic metabolism. What you'll also notice is our fatty acids can also come into the cell. They can be stored as intramuscular triglycerides or they can be brought through and they can also be converted into acetyl coate. So they can also contribute to aerobic metabolism in that instance. So we've got aerobic metabolism, fatty acids, aerobic metabolism, glucose, and a aerobic metabolism, glucose in this instance, producing lactase. And then finally, what's sitting over here is our intracellular creatin that can then obviously donate a phosphate once it's cretin phosphate back to a TP. OK. So ad P can become a TP and then A TP can be broken down. So already that's also giving you a clue that there has to be some form of aerobic metabolism that's involved with that rep phosphorylation. I'm creating phosphate. OK. So quick question again, which of the following is an incorrect statement about the energy systems. Energy systems are all working and switched on at the same time, energy systems operate one at a time and if one is switched on the others are switched off, one energy system may maybe predominantly used during a certain activity, which is, which is incorrect. B OK. So it has to be B cos we did say that they're all on, but it's the proportion of what we use in this instance. OK. So it's not correct to say that you just switch on and off. OK. So you've gotta think of them like dimmer lights and you can turn the dimmer lights up and down on them so they can all be on this. So, so you can flex them or move that energy through that system under a different intensity by switching your dimmer lights up and down in that instance. So it's often sort of um shown like this. We've got our three systems that we've talked about. We've got our cretin phosphate anaerobic glycolysis that you saw on the screen before and our oxidative phosphorylation predominantly through the fatty acid and the glucose it sends taken down into the mitochondria and used in the citric acid cycle and we can start to categorize them. So you can see that the speed of action is obviously very fast from crude and phosphate, fast from anaerobic glycolysis, and it's slow or very slow. In terms of aerobic metabolism kinda makes sense. Cos if you think about the number of steps and the number of membranes that you've got to pass through, obviously, it becomes more complicated in its system by the time you get down to the aerobic metabolism. However, on the other side is that we trade that off against the amount of A TP that we produce. So you can see that we can produce fast energy supplies to recruit and phosphate, but it's only a very small amount of A TP in terms of its 1 to 1 turnover. However, if we come through the systems, we actually ar arrive at oxidative phosphorylation, which is a large amount of A TP in the presence of oxygen. And when we say large, well, it depends on what you're talking about. We, we'll show you some slides in a minute where if we're speaking about glucose it might be 30 something A TP. But if you think about how long the fatty acid chain is, it could be over 100 a TP per fatty acid chain. So very large can be really large. And obviously, it takes longer to go through that process. The duration then is also uh indirectly related to the speed of action. So very short through to very long. And again, that gives us a clue of why we can only operate for about 6 to 10 seconds under crepin phosphate. And we're gonna already start to switch into aerobic metabolism at that point in time. On the other end, if we want to run a marathon, we're gonna have to predominantly use aerobic based pathways to supply that reef synthesis. Alright. So let's dive into a bit quicker for each of them. So let's start with the cr cretin phosphate. We said it's a high energy phosphate compound similar to a TP. So it's got that ruin plus phosphate. But having the phosphate means it can give that back to AD P to form a TP stored in the muscles and other tissues may also be referred to as the phosphocreatine PC PC R and CP system. So it just depends on what textbook you're kind of looking at at any one time North American European Austral Asian. Uh it serves readily accessible reservoir of energy and it makes sense. It's sitting in the muscle, it's close to those contractile proteins that we talked about before. If they break a TP down, cretin can resupply that phosphate and you can resync the AD P into A TP pretty quickly. It's just that we're limited on how much cretin is sitting inside that muscle fiber and therefore match phosphates available through reining the A TP. So what you can see here, cretin phosphate plus AD P using the cretin kinase enzyme re synthesizes A TP. And then we've got, we're left with cretin, it can go around and sort of find another phosphate if needed. Alright. So what we know is that occurs at a rapid rate and we can have very powerful muscle contraction. In other words, if we talk about muscle fibers being slow twitch, intermediate and fast twitch, it's gonna be those fast twitch fibers, you'll probably find the highest concentration of creten cos the mice and chains, those contractile chains are built for power and they're also slightly bigger in terms of their radius. So you can actually pack in quite a bit of cretin compared to a slow twitch fiber. What's interesting about crete metabolism is like most homeostatic models. There's a bit of a balancing going on. OK. We like to measure stuff all the time and then we like to make claims about that measurement. But what you can see like blood lactate like oxygen, all of these things are a balance. OK? So if we look at rein in the blood, it is a balance and it's a balance between what are you currently consuming or eating? What are you using? In terms of the um creatinine that is then taken from the blood, how much creatinine is then broken down to creatinine and then excreted from the body through the kidneys. And how much are we actually producing ourselves? Because we can actually produce creatin through various amino acid pathways and that would also form part of the net balance. So this idea of, of rein in your blood is actually a combination of all of these things in terms of net gain and net loss at any one time. So it's not, it's not simple. Um It can be quite complicated, but just in terms of its intake, you'll see that if you have a look at the range of different intake options you've got. And this one here is what is the amount of food required to reach 5 g of cretin. So if you obviously come down to the creten powder that you buy off the shelf, 5 g is 5 g, but you can look across the food and obviously, there's a predominance there of meats. You can see that there is a different amount of each of those foods depending on the crete and concentration. And you can see that for example, cow's milk and this one would be about 200 cups equivalent. OK. So again, depending on the food, depending on the food source, and depending on how rich that rein um concentration is, depends on how much you're actually going to consume if you want to consume equivalent to our 5 g of powder that you see in the bottom here. So as I mentioned before, it's a balance between what you eat. In this instance, we've got our dietary creatin coming in. It's absorbed through the small intestine comes in. It's used within the muscle. But creatinine can be metabolized into creatinine and that can be excreted from the body and it's done. So at the level of the kidneys, we can also go through these are just amino acids. And again, I'm not asking you to learn the biochemistry. But amino acids that are produced at the level of the kidney here can feed up to the liver and the liver can actually produce creatin in this instance. And that will contribute that creatin as well as the ingested to the total cretin pool in the, in the actual skeletal muscle. All right. So, obviously, from a sports nutrition point of view, this is probably the area that's most considered and it's probably one of the most researched sports nutrition supplements, isn't it? Ok. So if you think about it, it's easy to uptake, it's easy to digest overall, it seems to get good um outcomes, but it depends on the physiological situation. Is it a one off sprint? Is it repeated sprint? So, again, we won't go into that today, but there's a context of whether cretin is actually an ergo GIC aid. But it is just off note, it is we'll do a supplement lecture later. It is regarded as what we call a group, a supplement, meaning that it has good evidence that it supports physiological uh adaption and performance in terms of um uh performance outcomes in this instance. Alright. So just one more quick slide on this when you take that into the blood cretin, uh it makes its way through the circulation and you can see that there's a transporter. So in this instance, our cretin transporter allows that to be taken up into the skeletal muscle. Um but here's our bro here, creatine and that is then excreted at the kidney. So again, it's this net balance and it's the idea too that you think about most systems with transporters, if you've only got a certain number of gates that in itself is a limiting factor on how much creatinine can be taken up into a skeletal muscle cell, ok. So it's just think about it's not about just preloading as much creatinine into the blood. If there's only so many gates for it to get through, that's also gonna be one of the limiting factors for its uptake. Alright, so what happens when we start to use cretin and a TP in terms of sprint based activity? So this is our 100 m sprint occurring here. So for these athletes that you can see over on the left hand side, it's a, just over 10 seconds for the, for the females. Ok. So if we have a think about it, it's occurring pretty quickly. If we look at muscle, A TP and phosphocreatine concentration of the skeletal muscle, you can see that the A TP over that course of that sprint is relatively undisturbed. Ok. It goes down a little bit, but the concept is we're trying to keep it as undisturbed as possible. What's doing that is we've got cretin that's very high at the start of the um activity within around about 2 to 3 seconds. You can see a massive drop, ok? In other words, cretin phosphate is delivering phosphate across to AD P for that re synthesis uh back into att. OK. So you can see the biggest change there occurring in our CRP or cretin phosphate as it does its job for re synthesizing AD P plus phosphate. Ok. So what are some training activities in sporting events or exercises a predominantly utilized recruit and phosphate system? Alright. So again, the 100 m sprints there, but don't forget there's very powerful activities that are lasting for only a couple of seconds at a time. Bit of a summary. So it's a one chemical step system, it's catalyzed by, you would have seen that enzyme pop up. Cretin Kase. Again, the clue is if you see a se on the end, you know it's an enzyme. Ok. So cretin kinase that means it's an enzyme. It's facilitating the reaction. It's very fast. But what we're getting is one A TP re synthesized for each cretin phosphate molecule that's available within the cell. I know, in other words, it has the phosphate to give back to the AD P as I showed you before, it's around about 10 seconds. It's is its maximum duration. We saw that from the graph, from the 32nd graph, the aerobic metabolism is starting to start to predominate by the time we get out to 32nd, it's generally regarded as anaerobic. But don't forget that A TP itself is re synthesized by aerobic based systems. OK. So it's important to think about the overall uh long-term um production of that A TP. Um and it's obviously predominant in these power type events. So the question is, is it or is it not completely anaerobic? Ok. So sure on face value, it looks without oxygen, but the answer is not completely anaerobic. OK. And the answer of why this, why this is is important. So if you think about in terms of the entire pathway of creatinine cretin phosphate, in terms of the creatinine shuttle, it actually involves the mitochondria. So the mitochondria has to underpin some of this um turnover. And so there has to be some level of aerobic metabolism that sits underneath this. Alright. So let's have a look at the anaerobic glycolysis then now this is starting to move us towards a couple of different substrates or parts of the diet. So the first one here on screen is cho so it's a short handful carbohydrate. So carbohydrate in this instance is broken down into lactic acid, which is further moved across to lactate plus H positive ions. H positive ions being the acidotic part of the process in here. So you can see there's a couple of A TP being now produced, we only have one from the cretin phosphate. Now, we've got a few A TP. It gets a bit complicated because sometimes we actually have to spend a TP to make a TP. So let's have a look at sort of this net gain of A TP and whether we've had to spend it to make it in the first place. Alright, so again, we're gonna have a couple of pathways that on face value look a little bit more complicated, but you'll notice that a lot of the, the intermediates as we call them has been removed. So we're not asking you to replicate biochemistry pathways, but understand the concept that's occurring in this instance. Alright. So let's have a look and familiarize ourselves. We've got two starting points. The first one is glucose and the other is glycogen. OK. So remember we take carbohydrates in there's a range of different sugars that are involved with that. But if we just think about glucose as a primary one in this instance, what we can see in this instance is we can have glucose that travels through the circulation and it can arrive at the liver or the skeletal muscle or the heart and it can be uptake into the cell. Now, if this is a skeletal muscle cell, and we're not really using it at the moment that glucose can be stored as glycogen for later on. But it means that glycogen can equally be broken into pieces via glucose and be available to then enter into the glycolytic pathway. So what you'll notice is the glycolytic pathway involves spending a TP. OK. So to get glucose to glucose, six phosphate or glucose, six phosphate down into the rest of the biochemical tree, there's actually some spending that occurs, we then produce our electron transporters such as N A DH, but we also produce directly A TP. So in this instance, what you'll notice is there's four A TP and we've arrived at the bottom with two pyruvate. OK. So the fact that we've divided our glucose molecule in half means we've gone from glucose has six carbons. We've essentially sliced it in half. And we've ended up with 23 carbon pyruvate molecules at the bottom. You know, in this instance, what you'll notice is that oxygen supply or partial pressure of oxygen is low. So we're actually moving across and producing lactate. So that gives us a clue that in this instance, it's a fast anaerobic glycolytic pathway. There's in this particular cell, there's a low level of oxygen available and the contraction intensity if it was a muscle cell is quite high. So we need to produce this A TP quite rapidly in this instance. So it's an anaerobic process. But I think the key part is you can come from glycogen or glucose. And one of the advantages from glycogen is obviously already intracellular at that point in time when we come from glycogen, this is a process called glycogenolysis. OK. Alright. So the question here is glycolysis produces so many A TP but so many A TP are used in the process. And so the final or net A T production is. What out of those? What were you looking at? C? Yep. So we're 422. So glycolysis produces four but two are used in the process. So the final net what you've spent and what you're left over is two overall. OK. So they're pretty quick double what you got from the crete and phosphate system. But you've had to go through a few more steps. And again, it's still occurring in the cytosol of the cell. We haven't entered into the mitochondria. At that point in time in the cytosol, we've got our contractile proteins so we can shorten muscle powerfully. So obviously, if we talk about SCLE muscle cells, it's probably gonna be a predominance to the fast twitch still and probably the intermediates in this instance. Alright. So if we start to summarize this system, then it's an 18 chemical step system and six of the steps are repeated overall, we end up with lots of compounds and lots of enzymes. So, 11 and 12, but we're not asking you to learn the names of all those just realize there's lots of steps occurring. We have a rate limiting enzyme. So there's a couple of enzymes that are of interest in, particularly in sports nutrition. And this is the phosphofructokinase. Let me just go back and show you where that was. OK. This little enzyme up here and it's helping us to move our glucose six phosphate down into the next level of the um pathway. So this is what we call rate limiting enzyme. In other words, if we've only got so much of that enzyme, we can only convert so much glucose six phosphate into the next step. It's fast but not as fast as cru. And that makes sense. There's more steps has to be a bit slower, but it's still much more quick. It's much quicker than aerobic metabolism, which is gonna go round and round the citric acid cycle a little bit later. Overall, we classify it as anaerobic because oxygen hasn't been part of this process for a net gain of two A TP. And the predominance is around that 1 to 2 minute. OK. But like the graph I showed you before we're already starting to see that come through at about 30 seconds to 60 seconds. OK? But we, we certainly know events like the 400 m track event, for example, is very anaerobic type event with some aerobic metabolism starting to underpin that towards the end of the race fatigue is c pretty evident. Ok. So if anyone's using a predominance of anna and metabolism, it does come with what we call metabolic acidosis. So this is where lactate has been given a little bit of a bad name. Lactates, not necessarily bad lactate can actually be quite useful. Cells can re synthesize glucose from that and use it as a fuel. The acidotic part comes from the fact that the H positive ions are floating around and aren't being dealt with. OK. And that's the acid, that is what acid is. It's H positive ions not lactate, lactate is quite useful. Um So we end up with predominant systems in high intensity exercise, sustained, repeated sprits, for example. And that's why our glycogen levels will fall quite rapidly in, in events where we have to repeat, sprint over and over and over again in that instance. And hence, when we get back to the energy supply, it's gonna have to be a lot of carbohydrates that come on the back of that to re synthesize that Glycogen. OK. So what are the training activities, sporting events, you can see the classic here, the 400 m hurdles. Um but don't forget some of the events like BMX riding. Ok. They ballistically ride around that track in, you know, under a minute. Ok. So that is an anaerobic based event that's occurring in that sort of short track BMX type riding. Yeah, there's, there is a little bit of a difference if you've come from Glycogen because yeah, you, you haven't used that um A TP to come into the cell o on that instance at some point, glucose has to get into the cell at some point. But Puristic, you're absolutely right. Alright. This is another slide that I don't want you to get overly well overwhelmed with. Ok. So what we got here is two different exercise intensities, A and B exercise intensity A is at 60% of peak power, aerobic peak power and B is 100 and 10% of aerobic peak power. What we mean by aerobic peak power is we get you on a sort of a ramp test and we get you to cycle or run on a treadmill and you gradually work your way up until you reach peak VO two, ok? So that would be your peak power. You then set yourself at 60% or 100 and 10%. So 100 and 10% is, let's say your peak power on a bike was 400 watts. You'd have another, you'd have recovery. We come back the next day and you would be set on 440 watts. In other words above peak power. So there are two conditions the first one A is under peak power and the second one B is above peak power. Now we know we can ride 440 watts just cos our vo two max says we only got to 400. We can still, we can still generate more watts wattage than that. And that's what you can see. The, the problem is you don't last for very long, do you? Alright, so you can see what's happening here. These are our pathways that I showed you before. There's our glucose and glycogen going to pyruvate, there's our fats coming in and they're coming into the mitochondria and that works through this re synthesize of A TP and there's our cretin phosphate. What you'll notice is when you work at 60% we ve get very little or no accumulation of h positive ions or phosphates building up cos what you'll notice is they're being quickly retaken backup into the mitochondria. And that's actually the reason why having high training volumes and high mitochondrial volumes is actually really useful because what you can see is they're mopping that up. So if you take an athlete like Pogo, who doesn't seem to fatigue at all, one of the primary reasons that they reckon he can go day after day after day is his mitochondrial volume is so high and his ability to use lactate as a fuel and mop up h positive irons is, is massive. In other words, he has big energy mitochondrial systems available for him to do that. So who knows? And that makes sense. If you, if you're then just riding along at a submaximal pace, you don't really build-up acidotic conditions within the muscle. And obviously the higher you are trained, the higher you can actually perform those tasks for longer without those conditions changing. But if we ask you to cycle at 100 and 10% of your peak aerobic power, there's your glycogen. And what, what this diagram is showing is the relative portion. So you can see how the, the diagrams becoming thicker. So there's a big proportion of glycogen being used. There's a lower proportion of fatty acids. OK? So at high peak or bug peak aerobic power, you're not worried about using fatty acids. They take too long. You're gonna have to breakdown glycogen at that point in time because you're doing it at such high rate that results in accumulation of h positive ions and phosphate. And that in that instance, it starts to accumulate fatigue. So the contractile proteins start to suffer under those conditions. So it's not surprising you'll only last for a minute or 2, 100 and 10% but you could last for 45 minutes at 60% with a power. What's interesting is if you have a look at the release of h positive ions from the muscle cell and lactate, they don't relate directly to each other. OK? They're both kind of going up and you'll notice in that first time period, the phosphate, uh so the h positive lines are going up much quicker and the lactate are a little bit slower. Ok. So they're kind of related but not in sports science. We've got this absolute fascination with measuring lactate all the time and then assigning lactate to the anaerobic conditions that athletes are experiencing. And you can see from that graph, it's not exactly 1 to 1 and the relationship's not always the same. And the reason why is lactates produced, but it's also used and re synthesized. It's also taken up into things like liver and other skeletal muscle cells. So what appears in the blood is not necessarily what has been produced all the time. So it's, it's not the perfect marker that everyone likes to think in terms of its ability to assign the types of aerobic glan AOP metabolism that's occurring uh in, in the athlete. And so that's what you can see here. So we've got our glucose or glycogen breaking down to lactate. Lactate is taken back out into the veins and appears in the arteries. So you can see there's lots of different organs that are really interested in not taking that lactate. It's used as a fuel. Ok. So, and the, the classic example is go back to the SCLE, uh go back to the liver and the liver can actually through glu it's called gluco genesis. It can actually reform glucose in that instance and send it back out as glucose. Hence, if we get back down to an athlete like pogo, he's actually extremely good is what they're climbing at doing this particular um uh cycle or shuttle. Ok. Uh Hart loves lactate as a fuel as well. Alright, I'm going to break at oxidative phosphorylation. Give you guys a sort of a 10 minute break and then we'll get started again and we'll go through oxidative phosphorylation and then we'll have a look at some applied exercise physiology, conditions with some um dietary manipulations in the second half. OK. So take a 10 minute break and we'll get started again at sort of 25 past two, come and ask any questions in the meantime too. So I don't have to stop recording anything. SPEAKER 0 Yeah. And then just leave those hairs and like just yeah. State Department on Saturday. Yeah. You why? So? SPEAKER 1 Alright, let's go guys. So we can get out in good time. You can have a break if you happen to be going to the lab. So our third system is our oxidative phosphorylation system. In other words, our aerobic based system. Alright. So just as I showed you on that earlier slide, we've got a combination of substrates as we call them. But then if we think about the diet, our dietary intake that's going to be providing a fuel or pathway into our aerobic chain. So our cho is our carbohydrate, we've obviously got our fats and then PR O stands for our proteins. Remember, it's a little bit more complicated in just those three categories. And the example I like to give is fatty acids. So, fatty acids can range from only 12 or 14 carbons long and they can go all the way up to 24 carbons long. And then we've obviously got different types of fats. We've got saturated fat, mono unsaturated, polyunsaturated fat. So it's important to realize that again, we're simplistically thinking about these at the moment. But there's also obviously a lot of complications depending on how you actually break that each of those categories through the combination. Though, is we have carbohydrates fats and proteins that are providing a fuel or substrate source for our crib cycle and electron transport chain. As soon as we see those terms, we know that we've moved into the muscle fiber through the c of soul. And now we're thinking about the energy system being the mitochondria. So this is the powerhouse of the cell if we want to re synthesize a lot of A TP from any of these sources. So you can see on the slide here, we've got just three being shown, it's gonna be a lot more. As I said, one fatty acid chain potentially can be providing up to 100 and 1020 A TP depending on how many carbons is in that, right. So if we go back to this diagram that we showed before we arrived at this level, before we had glucose and glycogen being converted into glucose, six phosphate. We're spending a TP, producing a TP. But we finished there at Pyruvate and we moved over and we said that at this point in time, there's not enough oxygen inside the mitochondria. Let's just convert that into lactate h positive ions and we'll be done with it if we do have oxygen that's now being supplied. And we've got a good mitochondrial density. So particularly slow twitch muscle fibers are a good example of that. Then pyruvate will actually then be able to sense that instead of just being converted into lactate and leaving it at that point in time that it can actually move its way across the mitochondrial membrane. And you can see that here. So pyruvate moves itself across the mitochondrial membrane and converts into this little next step called acetyl coa. So acetyl coa is important because you'll notice it's also part the common part of the pathway where any of the fatty acids are also joining as well. If we're just considering those two substrates, we also don't want you to learn all the steps in place. But what we do want you to recognize is that there is a large number of steps that take place when that pyruvate gets broken into acetyl coa acetyl coa enters the citric acid cycle and it goes around and around and around. And depending on how many carbons is involved? It'll go around once, twice, 10 times. What it's doing is producing our little N A DH plus H positive iron electron transporters. Now, that's the key cos if you think about it, what we're doing is we're producing electrons that can charge, create energy. And if we can create energy, we could reattach phosphates to adenosine diphosphate dot AD P to recreate a TP in this instance. So that's what you're seeing. So the two energy, let's let's think of an electron taxis. The electron taxis are N A DH S and fa DH two. So they're just electron taxis that can convert and take electrons into the mitochondria and produce energy to do that. It requires oxygen. So oxygen is the final step in the electron transport pathway. And what it does is it then um picks up the ox, it picks up the electron and becomes water. So water then is the by-product of this process. OK. So here's an our next knowledge. Um fact checker. So we've got four statements there. We've got one. It is shuttled to the mitochondria after glycolysis, two pyruvate gets converted into this intermediate compound. Three, the final electron acceptor and four, this is formed during the creb cycle. Has anyone got any clues of what's going on? So this is shuttled to the mitochondria after glycolysis, it p pyruvate is converted into the intermediate compound. SPEAKER 0 Yeah. SPEAKER 1 Ah Well, it's really let's I'll, I'll flick through it, it's really acetyl coa in this instance. So the first one's pyruvate, pyruvate gets converted into this intermediate compound. So we go back up to this little thing. Pyruvate is shuttled through and it's converted to this intermediate compound. In this instance, the final electronic acceptor, as I mentioned before is oxygen, you have to have oxygen or this whole chain doesn't exist. In which case pyruvate is able to sense that and therefore just simply sort of cuts out at that point in time becomes lactose and four, this is formed during the creb cycle. Remember, I also call it the citric acid cycle. So, citric acid is formed during the cycle. OK. So they're kind of the four key things you should be sort of taking away from this part of the, the process so far, right. So the question is, how much A TP can you produce? Again, this is um a little schematic that shows our glucose that we had before going through glycolysis producing pyruvate p pyruvate is then shuttled through to acetyl coa. It goes round the creb cycle, oops sorry around the creb cycle and enters um the electron tran sorry, enters the electron transport chain produces our N A DH fa DH electron transporters and they're used to then produce a TP. So our levels are cytosol mitochondria and our mitochondrial membrane. So what you'll notice is that if we look at our A TP, it sort of changes as we go down, we're producing our two that we talked about before. And this is just net production. We actually produce two more just simply going around the creb cycle. But the majority of these are produced. If you notice here, six and 20 are produced because we produce these electron uh transport taxis and those electron transport taxis go into the electron transport chain. And then you can see there's a little multiplying factor. So for fa DH two, you get about 1.5 A two PS for N A DH S, you get about 2.5. So if you multiply those out, you get another six and another 20 it totals up to 30 in that instance. Now, just to note some textbooks will vary this number slightly. OK. So this sometimes will be varied up to two, it will be rounded up and this will be rounded up to three and you'll get a slightly different number at the end. OK? But it's actually the concept that's important. The concept is that we've produced our N A DH is an FA DH two. They're electron transporters, we can use the electrons to charge a little pump that then re synthesizes AD P into a TP. And it does it with those sort of proportions and therefore we can walk away with 30 A TP if we've gone through the whole process. OK. So that's an aerobic process. And you can see how important the mitochondria is in that process. OK. So those last two steps there have to be inside the mitochondria for that to occur. OK. So in summary, then we've got 100 and 24 chemical steps, 30 compounds, lots of enzymes. We've got rate limiting enzymes again, like phosphofructokinase. But we've also got some enzymes that sit within the electron transport chain. So in other words, just takeaway the idea that these don't just simply convert on their own, there has to be an enzyme present. And if the enzyme's not, there obviously slows the system down, we end up with um 30 A TP from glucose. And there's that point about if you come from Glycogen, you actually end up with 31 in that instance. The point is it's slow but potentially limited, limitless in terms of its duration. So you're more likely to use it in long, slow, low, low intensity based activity. So it's aerobic, but the fatigue is associated with fuel depletion over time. For example, SCLE muscle glycogen starting to become um lower. And so after a couple of hours, those glycogen levels fall and it becomes more difficult to then increase intensity at that point in time, long distance running, cycling, tour de France, riding, all those things associated with the aerobic phosphorylation system. Alright. So if we're going to now combine all of these systems into what we call fuel use, ok. How do actual athletes or individuals wanting to take part in physical activity. How do they actually mix these systems together? There's a couple of different key factors that are involved in that the ones that come to mind are exercise, intensity and exercise duration. Now, they're kind of inversely related, aren't they? So obviously, if you, the duration of exercise is only a minute, the intensity will be really high. If it's a couple of minutes, it might be still hybrid a little bit lower. But if your duration is now gonna be, oh, the intensity is now the intensity is gonna be much lower. So what we do is we often graph that off. As for example, here, the exercise intensity based on V two max. OK. So this is the max amount of oxygen an athlete can take in and utilize to produce aerobic A TP. So in this graph, we've got three intensities, we've got a low intensity 25% a moderate intensity, 65% and a high intensity 85%. In other words, we're going from kind of walk, walking type based activities to jogging based type running activities to all our efforts at 85%. Now, this hasn't come up nicely. There's actually a really light gray bar sitting just up. Can you see that? Yeah. So see where it says muscle glycogen here. OK. It's not, it's not in this one, I'll just draw it out for you. Draw a little bar goes up to there up to 200 OK. So draw a little bar there just hasn't come out on screen and then this 185 you can take it all the way up to 300. OK. So your muscle glycogen bar is actually sitting above that. I just realized it's not showing on that light screen there. So what you can see is that at low intensity, if we have a look at the fuel use between glucose and fats, we've got muscle glycogen, muscle triglycerides fats stored within muscle plasma free fatty acids fats running around in the blood circulation, plasma glucose, glucose running around in the circulation. So you can see at low intensity here, we're using plasma fatty acids, a little bit of plasma glucose and a little bit of intramuscular triglycerides. There is no muscle glycogen sitting on that. In other words, you can kind of chug along for hours on end and really not disturb your muscle glycogen much at all. Ok. It's not as impossible, but there's not a lot of effort to, to do that. 65%. You can see there's starting to be a bit more even distribution. Your blood glucose comes up a little bit free fatty acids are still providing energy. But your intramuscular triglycerides are now starting to provide a more predominant amount and lo and behold your muscle glycogen levels will start to fall. So if you've got an athlete running around 65 to 70% of their vo two peak or max aerobic capacity. The chances are that they can last for an hour or two, but they're gonna walk away with low muscle glycogen at the end. And therefore they're gonna need carbohydrate resin, well, resupply. So they can re synthesize glycogen after that activity. If we go to our 85% our bar goes right up to 300. So make sure you draw in your muscle glycogen bath. So in other words, once we go up to a high intensity exercise, blood glucose goes up a little bit, cos we can supply through the glycolysis process. Fats are actually coming down a little bit in a proportion, but our muscle glycogen is really high. So in other words, if we've got an athlete that's running around at 85% of vo two max for 30 40 50 minutes, they're actually gonna be using a lot of muscle glycogen during that time under that high intensity activity. Now, it's not to say you can't sustain 85% for hours either. There's some elite athletes who are quite happy to chug along at 85 and 90% for a couple of hours, which obviously then exacerbates it more, which means that they've got to be muscle glycogen really loaded prior because that's gonna be the primary source during that time. However, always say, however, the other interesting thing about it is that if you're a highly trained athlete, they actually get really good at using intramuscular triglycerides. So this is kind of let's call it a standard curve. But if we could put that curve up again and say this is an elite tour de France athlete, they potentially would also use a high proportion of intramuscular triglycerides, which does what saves on the muscle glycogen a little bit. Ok. So it's always a however, isn't it depending on the cir circumstance? Right? So, what we've got then is an intersection of carbohydrates, proteins and fats and they're occurring at this level here at acetyl coa. So you can see that we've talked about um glycolysis producing pyruvate aerobically around about 30 A TP or 31 from Glycogen. If we were to come all the way through the system from the top, we've got our fatty acids in this instance, they come through beta oxidation and you can see that they also join at the same level here. If we use P metate as the example to acetyl Coa, it's over 100 A TP. But obviously, that's a lot of a lot slower process to, to do that. And what's interesting is is if you do a calculation for the amount of oxygen used per A TP, fats are actually really quite inefficient. Overall glucose is actually the most efficient fuel fats are per oxygen fats produce a lot of A TP, but the amount of oxygen is slightly higher per A TP compared to glucose. And then finally, we haven't spoken too much about this today because it gets a little, a bit complicated. But we are, we do have the capacity in ourselves to use proteins or amino acids. And they can feed into the system in a similar position. And you can see here, we've got two alanine is soine. Two examples. After removing the nitro nitrogen, we can then produce about 10.5 A TP or about 34 from isoleucine. OK. So the capacity for bringing proteins through the system is possible. It's a much slower process and it occurs at a much more delayed time period. Ok. It can be quite serious for athletes who are obviously then depleted in those two first primary sources because protein then becomes part of the conversation on whether or not it's being um uh depleted in in those particular uh groups. So why carbs and fats preferred as a fuel source over a protein? Obviously, the steps are quicker uh and the ability to then move down the chain is um enhanced where proteins through the amino acid groups is a lot more complicated to um to feed into the system. What we do know is that we can look at the relative percent of certainly carbohydrates and fats through expired gas analysis. OK. Cos obviously, we consume oxygen and we produce CO2 and there's a nice interrelationship between our what was called the respiratory exchange ratio and the relative percentage of carbohydrates and fats. So, if we have a respiratory exchange ratio of one. That means that we're producing one carbon dioxide molecule for every oxygen consumed. Now, as you guys are all sitting around, you're all sitting around with an R er more like 0.7 0.75. Ok. What that says is that the relative percentage of carbohydrate use in the resting condition is very low and you're predominantly using fatty acid metabolism at this point in time. Ok? So just that ratio between carbohydrates and fats gives us a rough idea of the relative percentage um of those two fuel sources. Now, I'm not gonna read all this table out what this table is doing. And it's a sort of a study note table for you is that we've got liver muscle, adipose tissue and central nervous system. So sort of three big body systems that are really predominant on what they are demanding in terms of the energy supply. And then on the first column, we've kind of got a couple of different states as we call it. First one is a fed absorbent state. In other words, you've just had a meal, then you've got a post absorbent state a little bit later. And we've got our first fasting state 18 to 48 hours and then a starvation state. Ok. So what you'll notice is there's a couple of different comments about what happens to each of those systems. So I'll just run through a couple of examples here. So for example, in the fed state glucose, use as energy stored as glycogen in the liver converted to fatty acids if needed in the muscle glucose is used as energy or stored as glycogen in the fed state. But as if we start to come through to the fasted state liver, glycogen is now depleted because we haven't eaten. And the glycogen is then broken down and then used and spilled out into the bloodstream to be uptake by other tissues. So, in other words, as I said, I'm not gonna read them all out. It's just a, a overall summary between the fastest state, sorry, the fed state where you're taking in energy and storing it and then gradually, if you stop taking in energy, each of those systems, then have to then say, well, I'm gonna supply s previously stored fuel, preferentially glycogen for a period of time off to the bloodstream to keep blood glucose up. Um or to the muscle where the muscle glycogen levels will start to fall. The key part to this is what are we regulating? Does anyone actually understand what we're, what is regulation and control? Like what is the body trying to regulate in the, in? You've eaten? You've fueled up, you've stored this fuel as glycogen, for example, intramuscular triglycerides, you've stopped eat. What are we ultimately trying to regulate here? The body's sensing something and saying we've got to do something about it. We've got to keep this to a certain level blood glucose. So blood glucose needs to be regulated. It's like most things in the body, we have to look, we've got sensors and then we have to regulate. So what we're doing in this instance is we're looking at the blood glucose and from the stored previously stored energy supply, that blood glucose is being checked and then we can then start to release, for example, liver glycogen to maintain at least for a period of time. And obviously, if you don't eat again, that becomes more and more difficult over time. Alright. So what's the relationship between then triglycerides and fat oxidation? So this is an interesting little study. It's got three different energy expenditure conditions and they're made maintained in a very simple way. So in this, for instance, we've got our normal number of steps that you're trying to achieve. We've got a limited and a low number of steps. In other words, we're up at close to 10,000, we're halving that down to about 5000 and then we're quite low at about 2.5 1000. OK. So what you can see on the graph then is we've got a couple of postprandial areas under the curve for um plasma triglycerides and fat oxidation. So you can see for a normal person taking in our meal at this point in time and also kind of meeting the public health recommendations of being physically active. You can see that in this instance, the fat oxidation is highest and the postprandial plasma triglyceride is the lowest. In other words, by doing that physical activity, they've been able to um use fatty acids and keep the level of fatty acids in the circulation fairly low. You can see it then tips back. So as we come down to limited and low number of steps, our fat oxidation becomes low cos essentially, we're not being physically active and the area under the curve is now higher. So in other words, our post cranial plasma triglyceride area, small fats circulating around in the system at that time. So it gives us a clue just on normal low intensity activity, you can still get an interrelationship from a public health point of view on meeting kind of guidelines of physical activity or not meeting them and becoming sedentary at that point in time. Alright, so this relates to the lab that you're about to go through in the next week or two. This is the interrelationship. Then we've recognized that the energy systems kind of provide power under short intense periods, slightly longer, less intense periods and then long duration, low intensity in that point in time, we can sort of graph that off, can't we? So in this instance, we've got peak power output, it'd be like riding a cycle ogo meter. And if you ask someone to ride the cycle of Gome for a couple of seconds using the A TPP system, they can generate a lot of power, ok? And then stops, ok? It switches off if you ask them to do that activity again. But this time I want you to sustain it for about 30 or 40 seconds. The average power is gonna be lower. They can't do the same. You can't run 400 m at the same speed that you can run 100 m. Ok? You, you can't do it. Ok? So what you'll notice in the bike here, the power comes down if, then I'm asking you to run 800 m. Of course, the speed comes down and if I'm asking you to run five kilometers, the speed will come down again. So what you can see it's, it's called a hyperbolic relationship. So it's not a linear line, it's curve and you can see the greatest power output or velocity or speed intensity of exercise is highest in that 1st 30 40 50 seconds. And then there's a hyper bike or a rapid decline or decay occurring. And what we'll notice is as we get out towards about 10 or 12 minutes, we then get to a point where it doesn't matter if it's 15 minutes or 20 minutes or half an hour, you don't see much change at all. OK. So we end up with what we call a theoretical possible sustained power output. Now, that doesn't bring into account dehydration substrates, uh Glycogen depletion, boredom. Um All these things will eventually mean that we can't really sustain that forever. But at least from a theoretical point of view, we reached kind of a point in the curve where we're using aerobic metabolism by majority. And theoretically, we could continue to do so for a period of time. So our lab one is we're actually gonna ask you to create some power profiles of people in your group. We'll ask you to perform a couple of different activities using a cycle odometer and create this profile. Now, what would you predict if I had the 100 m Olympic sprinter doing this power profile? What would you predict in terms of the type of curve they produce? Yeah, extremely sharp, wouldn't it? And comes down? But if you ask him to run a marathon, they'd laugh at you. In fact, uh are you saying bolt has been claimed to have never run around a 400 m track at all? That's what he claims. He's never actually run a lap of a track. Alright. And that's how they train. Ok. They just train up and down straight. Alright, so what about if we put uh ultra marathon runner on it, what would that curve look like flatter, wouldn't it? Ok. So you can see that the power profile is actually starting to characterize the energy system predominance or the characteristics of that person, whether endurance predominantly or power or intermediate. OK. Field based activities like field hockey, for example, are kind of a mix of the two overall. And obviously that relates to the muscle fibers that we have and the majority energy systems that those fibers like to use as well. Alright, so this is a little um study that was done looking at what we call functional threshold power uh over 20 minutes. So this is sustained aerobic metabolism and the interrelationship to then a time trial. Ok. So it's a cycling based study. So the FTP you may have heard, often regarded as sort of that sustained 20 or 60 minute FTP is the ability to sustain that power, constantly providing aerobic based fuel source over and over again. So you can see here that this group here are averaging around about 260 to 100 and 70 watts. It's pretty steady. There's a large variation within the group, meaning that some people are probably sustaining close to 400 other people can only do about 250 same individuals giving a power profile here. So this is a power profile based on a slightly different activity rather than the work done over 20 minutes. They actually then say do a race that goes for 16.1 kilometers and we'll have a look at what sort of power profile you'd provide from that, which is a bit more like racing. It's a time trial. So you can see here they go out the blocks pretty quickly compared to the FTP. OK. But they can't sustain it. And then they sort of settle in. They sort of think halfway through that they're getting excited, can't sustain it. And they settle in again. So it's around about sort of 300 watts. Ok. So it's again, something, something similar. So the question that they asked was, is there interrelationships between these go again? I don't want to spend a lot of time. But what that shows is there's sort of pretty decent little correlations occurring here between actual time t performance and the predicted. And then what we call critical power and which is the critical power I talked about before, if I just go back here and look at this 12 minute sort of critical power time, sorry back here versus the FTP at 20 minutes underneath, you can see there's a bit of an interrelationship occurring. OK. So there is correlations with doing these types of assessments in terms of the aerobic based um uh characterisations that occur based on doing the assessment. The reason why I'm raising this is we're getting you to create power profiles. You're gonna create a power profile of around about 12 minutes in this lab. And that little average workload that you can do is a theoretical one that you can continue to do. But we're gonna then ask you to then do a longer activity in the labs coming up after that. So we'll set you at a relative percentage slightly lower. OK? But the reason why we can do that and be pretty assured that you can last for a certain amount of time is because of these correlations that occur in terms of the aerobic metabolism. Alright, so what happens when you start to exercise? We said before oxygen consumption, if it's an aerobic based activity, oxygen consumption is an indirect measure of your energy expenditure and heat production. So in this instance, this is a classic exercise test where we've starting the person off at very low um percentage. And we're asking them to either run on a treadmill or a, or ride a bike until they reach a theoretical max. And you can see that our VO two has risen from under 20 mils per kilogram. It's up to about 65 it's not elite, but it's well trained in that instance. And you can see at that point in time, it kind of reaches a theoretical plateau. In other words, they continue to exercise for another 30 seconds and the oxygen consumption doesn't go up. You don't often see that and needs high motivation to be able to do that. Uh And they re then reach what we call voluntary exhaustion. So they can't go any further based on the aerobic based system in that instance. OK. So that's your classic what we call linear response. But if we do submaximal activity, which is generally most of the time, what athletes are doing over and over again is that we get these kind of patterns don't, we? So we have a low oxygen consumption. We then start exercise. It doesn't go straight up. It actually takes a little bit of time to go up. It reaches a theoretical oxygen consumption that meets the aerobic metabolism. Ok. So they're now delivering enough oxygen to supply the mitochondria to do its job of re synthesizing A TP and then stop exercising, the oxygen consumption goes down and eventually comes back to rest. So we can see there's this period of time where we don't quite meet the oxygen consumption. There's also a period of time afterwards where we're actually over excessive post oxy exercise, oxygen consumption, even though we've stopped exercising. Now that if you were in the 19 eighties, you would say this was oxygen debt and oxygen repay that's no longer existing anymore. OK. We don't believe that anymore. What we do know is that this slow sluggish star, it does take time for your heart rate to get up your breathing, to increase your blood flow, to increase. It does take time for that to occur. It doesn't go up. We're not robots, it doesn't go up in a second equally when we stop exercising blood flow is still up where then obviously resetting things like electron balance across cells. Um There's blood supply that's still continuing to occur. So oxygen uptakes hi has to stay high afterwards as well. So the question is why is there a lag time uh at the onset of exercise. I think I've slightly answered that. If you think about it, if you start exercise, heart rate will take 30 40 seconds to start to rise, breathing frequency, the same blood flow, the same. Therefore, there will be a period of time where oxygen consumption will be below the desired for. The task. Doesn't mean that you're fatiguing. It just means that that period of time is, is what we call a delay. What I'm interested in is what happens between different exercise intensities, which then relate to obviously what fuel use is being used during aerobic based activity. So this is our incremental exercise test that I showed before moderate heavy, severe vo two max vo two peak. And you can then see that if we then set the person on an individual, um, period of moderate, heavy or severe on three different occasions. For up to half an hour, your oxygen consumption will follow three different paths. If you're under moderate exercise intensity. Back at these low conditions here, your oxygen consumption will rise and stay pretty flat if we're asked to exercise for a sustained period of time, for heavy oxygen consumption will come up. But what you'll notice it will continue to crawl even though workload hasn't increased oxygen consumption will continue to rise. Ok. So you can still be running at the same speed, still be cycling at the same power output. But your oxygen consumption will actually continue to rise at that instance, if we give you a severe bout. So in other words, give you something close to your VO two max. You can see that it's supposed to be here. It'll actually go up and continue to rise and potentially actually reach VO two peak during that time. So what that says is under constant work rate, depending on whether it's moderate heavy or severe, your oxygen uptake will continue to rise and exponentially arise, which we see in this graph here, the slow component is really quite this. So just to go back, this is called the slow component. In other words, it slowly drifts up. But what you can see is the slow component becomes more exacerbated, the higher the exercise intensity. So the question I'm getting to is what's driving that if your speed on the treadmill is the same and your cycling power outputs the same. Why is it the oxygen consumption is continuing to rise based on the energy systems? Alright. So if we have a look at the oxygen flow component, there's obviously lots of things that are asking for oxygen. So 80% of what's asking for oxygen is the contracting muscles, you can't forget that there's also a around about 15 to 20% oxygen being asked for by other supporting systems. So here is the work of breathing the heart, uh auxillary muscles associated with, you know, just simply maintaining your posture. What we do know is that from the contracting muscle point of view, we've obviously then depending on the intensity, if we go to severe, we're starting to produce things like the H positive ions and phosphates that we talk about before, that can't be then taken back up into the mitochondria. So they start to accumulate. We also know that the higher and severe exercise intensity, if we're gonna maintain that, we actually need to start to recruit more muscle fibers into the system. So remember, muscle fibers can be their motor units. And if we do a very easy task, we only use a few moderate heavy, you start to recruit more muscle fibers as we recruit into those muscle fibers, we're recruiting into our type two B fast twitch fibers which are low oxidated fibers and quite inefficient. In other words, they then start to say, well, I can give you the work, but you're gonna have to give me more oxygen to be able to do that. In other words, your efficiency drops and your oxygen consumption starts to rise at that point in time for the athlete. That means that they're gonna potentially gonna be using things like muscle glycogen at a much higher rate at those more severe exercise intensities compared to the low. Right. Again, this looks fairly complicated, but I'm gonna try and boil it down for you. We've always said that your factors for determining your energy expenditure is your, how intense you're exercising and how long you're doing it for the factors that boil into that is, are you going to change your metabolites? Like producing h positive ions? How much energy is going into the actual coupling of the proteins inside the muscle? How much muscle fiber recruitment into those type two B? And then the muscle temperature itself, what these do is they then are affected by the training status. So ultimately, what athletes are doing is they're trying to modulate these to maintain as least disturbance as possible. And therefore they can continue to sustain that exercise for as long as possible. So if I just flick up the next one, what I want to show you is that from the oxygen slow component point of view, the studies that we've done in terms of being able to change metabolites or change the excitation construction coupling or the muscle temperature don't really have much of an effect. The thing that has the major effect is this muscle fiber recruitment. So if you have to ask more fibers to do the task and sustain the task and those fibers are fast twitch, inefficient fibers, that's actually what's going to drive that oxygen consumption up during those more severe exercise bouts. Ultimately, what's happening is that the work or mechanical efficiency is becoming quite poor. In other words, you're using a lot of oxygen for the same amount of work and you're probably not gonna last for much longer at the same time, you're also going to be using up a lot of fuel like muscle glycogen during that period of time. Alright. So the question is what from our point of view in sports nutrition, where is the evidence that that oxygen slow component could be changed or modulated? Ok. So I'm just gonna give you three to finish with today. The first one is carbohydrate depleted versus carbohydrate restored. So in other words, what we've got here is a little experiment. It's got pulmonary oxygen uptake. Just think of that as your VO two. OK, you're consuming oxygen and this is the time it's occurring for. OK. So it's around about, it's like that FTP test. I show it's around about 20 minutes. You can see that it's, it's a fairly steady state, but there's a couple of things occurring in this instance, in the left hand graph, we've got our control versus our carbohydrate depleted. So the first thing you'll notice in the same individuals, if there are control. In other words, their muscle glycogen levels are normal inverted commas, they go up like we experienced before. So they're given the task of running or cycling and their oxygen stays pretty steady during that time. If they come in as carbohydrate depleted, you can see straight away that they become more inefficient. And in fact, by the end of the activity, they're statistically higher. In other words, there's more oxygen slow component occurring in the carbohydrate depleted group. If we go to the left hand side here and we have this is replenished. You can see that you can restore that oxygen efficiency by replenishing the muscle glycogen. So, from a sports nutrition point of view, it's really important to understand the severity of the exercise, the intensity. And then also whether or not you've got enough carbohydrate to restore muscle glycogen. So that on the next exercise about you don't lose efficiency in that task. Ok. So straight away, there is an interrelationship between diet and exercise efficiency. Beet juice. Has anyone, does anyone use beet red juice? It's quite popular. No, no-one's used it. No. Ok. So it's, it's boomed on in the last 20 years. The really, the reason why beetroot juice is it. It's right. It's not the beetroot juice per se. It's the fact that beetroots contain dietary nitrates. Ok. So if you think about it from that point of view, there's lots of foods, green leafy foods, there's lots of stuff that contain it. But it's obviously the one that's got the most amount of, uh, sports science literature that that's occurring. Uh, there's a guy named Andrew Jones from the US, uh UK that's done a lot of work on this. So he's shown over and over again that exercise performance can be enhanced by consuming beetroot juice. Now, the beetroot juice is just a carrier for the nitrates, the nitrates, what they're doing. They're actually classed as a group, a supplement. So they're performance enhancing. So there are so many papers now that prove that consuming nitrate actually helps blood flow. And we're actually thinking it's more than blood flow. It's actually skeletal muscle function occurring as well. And the reason why it's doing that is the nitrate contributes to nitric oxide synthese production, which in turn promotes vasodilation. So in other words, when you, particularly when you start that exercise, the ability to vasodilate and allow blood to bring oxygen in is enhanced after being on nitrates. OK. In that instance, so it's a group a it's perform enhancing. So part of that is L arginine also stimulates nitric oxide. So they've also proven it through that particular pathway that again, as long as you can produce nitric oxide, you can produce blood flow enhancement. Um And so what this study here shows this is a beet reduce study showing again oxygen uptake um with without, so without beat reduces, the higher one with beet reduce is the lower one and it's also got muscle phosphocreatine that we talked about before. So what you'll notice is when the subjects or the participants in this trial are given beet reduce, they're more efficient in their task and they actually last longer, see, see how the dots out further here. So they're lasting longer before fatigue and have a look at this, they disturb their th they there is a less disturbance of their phosphocreatine levels in that instance. So in other words, they're able to cope with the task better. And if you boil it back to the mechanism, it's probably because of the muscle fiber type difference in terms of the opening up blood flow, supplying oxygen, maintaining type one efficient fibers and having to recruit less into the type two effi inefficient fibers during that time. So that comes back to delivering oxygen. Ok. So that's the second example. So we've done carbohydrate depletion. As one example, nitrate provision is another sport science or sports nutrition approach to prove a change. Third one and the last one I'll do with you today is the classic bicarbonate. Again, this is also regarded, in fact, all three I've given you today are regarded as group. A carbohydrate loading is a performance enhancer. Nitrate is a performance enhancer and sodium bicarbonate is regarded as a performance enhancer as well. So this is based on the Australian studio, sport classification guidelines. We'll talk about that later in the session. But again, the group a take it, they'll probably have an effect on an athlete. So what this little test is is we've got a, again, a rapid increase in oxygen consumption related to a initial surge in oxygen consumption when you've started running on the treadmill, riding the bike and then a gradual crawl in oxygen consumption. And then whether or not you then enter into the slow component or not. Ok. So there's two arms to the test. So they were individuals that were given bicarbonate and then not given bicarbonate, they've done them in a classic crossover. You get that first, you get that first, we'll cross you over a week later and make sure that there's no bias. What you'll notice there's a couple of things occurring here that these two components have changed in the presence of sodium bicarbonate. OK. So in the first instance, if we look at this part of the curve, the rapid component can part, they spend more time in that part. So in other words, they, they don't enter into the slow component until later. In other words, the efficiency is improved probably because the bicarbonate buffering some of that acid production. In that instance, the second part is if we have a look at this a three part here, the slow component, they've got a lower increase in the oxygen slow component. In other words, they've stayed within those more efficient type one fibers and haven't to, haven't had to recruit into the type two fibers as quickly. Therefore, they've both experienced the slow component, but the slow component is less aggressive once on sodium bicarbonate. So again, it means that they can buffer this change here. And if they buffer that change, they potentially are going to ride longer run, longer fatigue less at that point in time. OK. So that's our third example for m manipulating the energy systems in this instance. Alright. So that's the end, there's a summary there. What we've got is hopefully you've taken away the idea of exercise, intensity and duration enables us to start to predict the dimmer switches in terms of our energy system predominance. In that instance, even short bouts of exercise require aerobic metabolism. So hopefully you take the matter the way as well. Um We've talked about the mix of fuels and then we've done a little bit on the oxygen slow component and then how it can be influenced by nutritional interventions. And the three that I've given you an instance with carbohydrate depletion, the nitrates and the bicarbonate. Uh all being um group a performance enhancing. It's not surprising I haven't given you group C because you wouldn't see anything. Group C are nonperformance enhancing and you wouldn't see anything at that instance. Ok. Any questions. Otherwise we're gonna end up having a break before you head to the lab with Joel. Any questions I I'm back in about four weeks or so and I, yeah, so back to Joel, I'll come back and we'll do some supplement lectures in a couple of weeks time. Alright, thanks. Oz. SPEAKER 0 Thank you. And.