MCDB 250 Exam 1 Guide PDF - Sex Determination and Reproductive Tract Development
Document Details
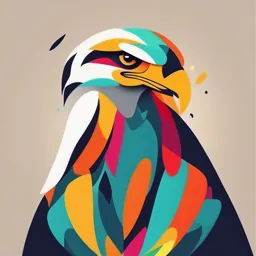
Uploaded by AdventurousCalcite
Tags
Summary
The document appears to be a lecture guide or notes on the development of the reproductive tract and sex determination, including topics such as Jost experiments, the SRY gene, and various disorders. It covers gonadal development, uterine development and external genitalia development. The guide also discusses conditions such as Turner's Syndrome, Klinefelter syndrome, intersex conditions, and disorders of sexual tract development.
Full Transcript
Lecture 2 - Development of Reproductive Tract Jost Experiments ○ Female = default state Male gonads needs to make something ○ Fetal rabbit gonad differentiated ○ Manipulation of developing reproductive tracts ○ Unilateral castration in...
Lecture 2 - Development of Reproductive Tract Jost Experiments ○ Female = default state Male gonads needs to make something ○ Fetal rabbit gonad differentiated ○ Manipulation of developing reproductive tracts ○ Unilateral castration in XY & testis graft in XX ○ male characteristics must be imposed on the fetus by the testicular hormones testosterone and AMH in the absence or inactivity of these hormones, the fetus becomes phenotypically female Need androgens ○ experiment blocked the normal process of hormonal production from the gonadal tissues during embryonic development hormonal signals from the male gonad are essential for embryonic development into males signals are missing, every embryo develops into the female sex ○ Central paradigm for reproductive tract development ○ Sexually dimorphic establishment of the reproductive tract requires Regression of 1 of 2 primitive ducts Maintenance of other Sex determination gene ○ SRY - sex determining region of chromone Y ○ DNA binding protein ○ Controls activity of certain genes ○ Activated shortly before gonadal differentiation ○ Found only in mammals (placental & marsupials) ○ Mutations in gene led to sex development disorders Female to male sex-reversal in XX Sry transgenic mouse ○ Single gene essential for initiation of mammalian testis development Gonadogenesis ○ Coup-tfll ablation leads to retention of wolffian duct in the female embryo ○ Active processes on both sites ○ Elimination of the male reproductive tract in the female embryo is actively promoted by COUP-TFII Supporting cells also require identity securing factors: RUNX1 & Granulosa Cells Fibroblast growth factor (FGF) promoted Wollfian duct maintenance ○ female mouse embryos lacking Coup-tfII in the Wolffian duct mesenchyme became intersex that contained both female and male reproductive tracts ○ enhanced p-ERK signaling in Wolffian duct epithelium was responsible for the retention in an androgen-independent manner support our hypothesis that elimination of Wolffian ducts in female embryos is actively promoted by COUP-TFII, which suppresses a mesenchyme-epithelium crosstalk responsible for Wolffian duct maintenance ○ p-ERK signaling and COUP-TFII are linked in that COUP-TFII, a nuclear receptor transcription factor, can be regulated by the ERK signaling pathway, often acting as a negative regulator by inhibiting its activity in certain cellular contexts, particularly during development and differentiation processes essentially, when ERK signaling is activated, it can influence the expression and function of COUP-TFII, impacting downstream cellular events like cell fate determination and tissue development ○ MD & WD = Mullerian & Wollfian ducts Gonadogenesis Primordial germ cells arrest in mitosis ○ Primordial germ cells become spermatogonia stem cell pool ○ Mitosis of spermatogonia occurs everyday post puberty Sertoli cell supports spermatogenesis Granulosa cell supports ovaries & oogenesis Leydig cell makes testosterone Theca cell makes estrogen & progesterone ○ In contact with oocyte Defined number of oocyte Millions of small sperm Germ cells line inside of seminiferous tubules Ovarian Development Gonad is regionalized, with the cords enclosing the germ cells in the cortex & the medulla without cords A lot of the oocytes die ○ Some go onto to develop surrounded by primary, secondary, and tertiary follicles ○ A, b, c, d follow primordial, primary, secondary, tertiary Uterine Development Uterus develops from middle section of Mullerian ducts after they fuse Progress regulated by homeobox genes Bipotential gonad = bipotential tissue Development of external genitalia ○ Shift from androgen independent to androgen dependent Non-binary & atypical reproductive tract development Intersex ○ Term for individuals born with several variations in sex characteristics: chromosomes, gonads, sex hormones, or genitals ○ Not same as transgender, but not mutually exclusive ○ Anatomy doesn’t fit binary female male ○ Doesn’t have to be just external Turner’s Syndrome ○ Complete or partial missing x chromosome ○ 1 in 2,00-2,500 females ○ 75-80% of cases the single X comes from egg & the sperm is missing the sex chromosomes ○ Can be treated with hormone replacement therapy Klinefelter syndrome ○ Male born with extra X chromosome ○ Affects 1 in 500-1,000 males ○ Syndrome variants with varying manifestations (behavior/cognition/androgen) ○ XXY, XXXY, XXXYY, XXXXY, any # of X & Y chromosomes True Hermaphroditism ○ Have both Mullerian & Wolffian Duct derivatives (ovarian & testicular tissue) ○ Karyotype variable: XX (with SRY translocation) XY (with Y point mutation - likely SRY) XX/XY mosaicism ○ Ambiguous external genitalia or genitals that don’t match karyotype ○ Gender assignment based on anatomical findings - surgical removal of structures not related to assigned sex Pseudohermaphroditism ○ Female pseudohermaphroditism: masculinization due to high levels of androgens from adrenal cortex (congenital adrenal hyperplasia-CAH) or excess maternal androgens (exposure during development) ○ Male pseudohermaphroditism: Genetic male with feminine external genitalia failure to produced or utilize testosterone androgen receptor deficiency Leydig-cell agenesis Congenital Adrenal Hyperplasia ○ Caused by lack of enzyme needed to make cortisol & aldosterone ○ Makes body make excess androgen ○ Accumulated precursor ends up as antigens? Leads to XX with XY phenotypes ○ Female pseudohermaphroditism Disorders of sexual tract development: female 3-6% general population 24% in women with miscarriage & infertility Difficult to determine since many don’t get found until trying to get pregnant Didelphus ○ Failure of Mullerian duct to fuse, results in 2 separate structures Unicornuate ○ One of the Mullerian ducts doesn’t fuse properly 1. Unicornuate Uterus (Type II) – A uterus that develops from only one Müllerian duct, resulting in a single uterine horn. ○ (a) Communicating: The underdeveloped horn has a connection to the functional uterus. ○ (b) Non-communicating: The horn is isolated and does not connect to the main uterus. ○ (c) No cavity: The underdeveloped horn lacks an internal cavity. ○ (d) No horn: The second horn is completely absent. 2. Uterus Didelphys (Type III) – A failure of the Müllerian ducts to fuse, resulting in two completely separate uteri, each with its own endometrial cavity and possibly a duplicated cervix. 3. Bicornuate Uterus (Type IV) – Partial failure of Müllerian duct fusion, leading to a uterus with two horns. ○ (a) Complete: A full division between the two cavities. ○ (b) Partial: A partial division where the two horns share some connection. 4. Arcuate Uterus (Type VI) – A mild midline indentation of the uterine fundus due to incomplete resorption of the septum. It is generally considered a normal variant. 5. DES-related Uterus (Type VII) – Caused by in utero exposure to diethylstilbestrol (DES), leading to a T-shaped endometrial cavity, which may be associated with reproductive complications. Certainly! Here are the reproductive implications of each uterine abnormality: 1. Unicornuate Uterus (Type II) Implications: ○ Increased risk of miscarriage, preterm labor, and intrauterine growth restriction (IUGR). ○ Higher chance of infertility due to reduced endometrial surface area. ○ If a non-communicating horn has endometrial tissue, it may cause pain or hematometra. ○ Increased risk of ectopic pregnancy. 2. Uterus Didelphys (Type III) Implications: ○ Often associated with a double cervix and sometimes a vaginal septum, which may require surgical correction. ○ Some individuals have normal fertility, but others experience recurrent miscarriage or preterm labor. ○ May cause complications in fetal positioning, leading to increased cesarean section rates. 3. Bicornuate Uterus (Type IV) Implications: ○ Increased risk of second-trimester pregnancy loss due to insufficient uterine space. ○ Higher rates of malpresentation (e.g., breech position). ○ Possible menstrual irregularities. ○ Increased risk of recurrent pregnancy loss and preterm labor. 4. Arcuate Uterus (Type VI) Implications: ○ Generally has minimal impact on fertility and pregnancy. ○ Slightly increased risk of miscarriage, but most pregnancies progress normally. ○ Often does not require treatment. 5. DES-related Uterus (Type VII) Implications: ○ High risk of infertility due to implantation failure. ○ Increased miscarriage and ectopic pregnancy risk. ○ Higher risk of structural cervical incompetence leading to preterm birth. ○ Associated with increased risk of clear cell carcinoma of the vagina and cervix in exposed individuals. Each condition varies in severity, and reproductive outcomes can depend on individual cases. Some may require surgical correction (e.g., metroplasty for a bicornuate uterus), while others may be managed with close monitoring during pregnancy. Let me know if you need more details! Disorders of the sexual tract development: Male Hypospadia ○ Abnormal positioning of the urethral opening ○ 1 in 250 male births Cryptorchidism ○ Failure of 1 or more testicle to descend ○ 3 in 100 male full-term births ○ 30 in 100 preterm male births Androgen exposure from PCOS women ○ Prenatal androgen exposure & transgenerational susceptibility to polycystic ovary syndrome Lecture 3 - Sex Determination Two types of sex determination: environmental & genetic Environmental ○ Non genetic cue ie temperature Genetic: Sex determined by chromosome content 3 levels of sex determination: chromosonal, gonadal, phenotypic 1) chromosomal ○ 46XX or 46XY ○ SRY gene ○ Can experience sex reversal due to chromosomal exchange 2) Gonadal (Primary) ○ Gonad develops as testes or ovaries ○ Determined b gonadal sex, SRY gene for testicular development ○ Gonad appears in week 4 as indifferent bipotential genital ridge Develops from embryonic mesoderm adjacent to developing adrenal & kidney Week 5 - cells from coelomic epithelium migrate inward to form primitive sex cords Week 6 - primordial germ cells have begun migrating to gonad Gamete Development: Specification ○ Without BMP4 signaling, PGCs fail to form, and without Blimp1, the specified cells may revert to somatic fate instead of becoming germ cells ○ BMP4 secreted by extraembryonic tissue Induces formation of primordial germ cell from epiblast by activating signaling pathways like SMAD Signals epiblast cells to become PGC ○ Blimp1 activated in response to BMP4 to repress somatic cell fate & help epiblast cells commit to germ cell lineage Represses genes associated with somatic differentiation Guards PBC from regressing back to somatic lineages ○ Migration ○ Target site of migration is the indifferent gonad ○ Chemokine gradient attracts germ cells to the gonads ○ Signaling protein expressed at high levels at the gonad & germ cells migrate toward the source ○ Attraction chemokine: SDF-1 ○ Receptor: CXCR4 ○ Disruption of signaling prPGC Formation & Early Migration ○ Primordial germ cells (PGCs) are specified in the posterior epiblast (in mammals) or extraembryonic mesoderm (e.g., near the allantois in mice) need to migrate to the developing gonadal ridges (indifferent gonad) to properly differentiate into spermatogonia or oogonia. ○ Guidance Mechanism: Chemokine Signaling ○ SDF-1 (Stromal cell-derived factor 1, also called CXCL12) is a chemokine secreted by the gonadal ridge. ○ CXCR4 is the receptor expressed on migrating PGCs.] ○ PGCs detect the increasing SDF-1 concentration and migrate toward the source (gonadal ridge), following the gradient.events proper migration ○ PGC originate in primitive streak (a) & migrate along the gut ot the developing paired gonadal ridge (b) ○ C & d = cross section of gonadal ridge at 4 & 5 weeks Gonad differentiation If SRY present in indifferent gonad at 6 weeks: ○ One set of specific genes activated ○ Indifferent gonad turns to testes Absence of SRY: ○ Different set activated ○ Becomes ovary Germ cells migrate into gonad at this time Gonadal development Male embryos with SRY, by week 8: ○ Cells of coelomic epithelium differentiate into Sertoli cells (make MIS (mullerian inhibiting substance), support spermatogenesis) ○ Sertoli cells organize into testis cords ○ PGCs incorporate into testisords ○ Leydig cells (make T) develop between testis cords Female embryos at week 10 ○ Inner medullary cords degenerate and new cords form in the cortex ○ PGCs mix with these new sex cords (if not, cords will degenerate) ○ Cords surround germ cells oocytes) to form primordial follicles ○ No MIS = Mullerian duct ○ develops into female tract After migration: Meiosis* (germ cell chromosomal development) and gamete development span fetal and adult life In males: Germ cells associate with testicular cords. ○ Onset of meiosis and sperm cell production begins at puberty Females: Germ cells associate with somatic pregranulosa cells to form primordial follicles ○ Meiosis begins during late fetal life ○ Eggs arrest and resume meiosis at ovulation 3) Phenotypic (secondary) ○ Determines sex structures outside gonad ○ At the same time germ cells are migrating, 2 sets of ducts develop that will eventually lead to the outside world ○ Internal genitalia Wolffian ducts = male Mullerian ducts = female ○ 2. External genitalia Two hormones made by the testis govern phenotypic sex: ○ Testosterone ○ Mullerian inhibiting substance or Antimullerian hormone (AMH) Development of external genitalia Both sexes start with same structures, which develop along different lines under the influence of dihydrotestosterone (DHT) The default condition is female: ○ In the absence of DHT, external genital structures develop along female lines T (testes) -> (5a- reductase; testes/skin) = DHT In the absence of DHT: ○ genital swellings -> labia majora genital folds remain unfused -> labia minora genital tubercle -> clitoris urogenital sinus -> lower ⅔ vagina In the presence of DHT: ○ genital swellings migrate -> scrotum ○ urogenital folds enlarge, enclose penile urethra -> penile shaft ○ genital tubercle -> glans penis ○ urogenital sinus -> prostate gland Can phenotypic sex differ from gonadal (or chromsomal) sex? Gonadal dysgenesis (XX or XY) ○ 46,XX Gonadal Dysgenesis (Pure Gonadal Dysgenesis, XX) Individuals have a typical female 46,XX karyotype but underdeveloped or streak (underdeveloped) gonads (fibrous tissue instead of functioning ovaries) often present with primary amenorrhea (no menstrual periods) and delayed puberty Normal female external genitalia and Müllerian structures (uterus and fallopian tubes) are present Can be caused by mutations in genes such as FOXL2, BMP15, or FSHR ○ 46,XY Gonadal Dysgenesis (Swyer Syndrome) Individuals have a 46,XY karyotype but develop as phenotypic females due to dysfunctional testes that fail to produce testosterone and anti-Müllerian hormone (AMH) Since AMH is absent, the Müllerian structures (uterus and fallopian tubes) develop, but gonads become streak gonads Affected individuals typically have female external genitalia and lack secondary sexual development without hormone therapy Often caused by mutations in SRY, SOX9, or other genes involved in testis development High risk of gonadoblastoma (germ cell tumors) due to the presence of Y chromosome material ○ Androgen insensitivity ○ A mutation in the X-linked androgen receptor gene (AR) causes XY males to become phenotypic females ○ Testosterone is produced, but not testosterone receptors; cells develop as ○ females ○ Phenotype: testes present, but female external genitalia, scant ○ pubic hair, +breast development 5α-reductase deficiency ○ A mutation in the enzyme which converts T to DHT ○ Phenotype: undescended testes, internal male ducts present, but ambiguous genitalia, underdeveloped penis, and short vagina ○ Often raised as girls ○ At puberty, some DHT gets made, and they develop a male appearance: The testes descend, the penis enlarges, facial hair appears, the voice deepens, muscles develop. Chromosomes & gonadal development Lecture 4 - Sexual reproduction & meiosis Types of reproduction: Asexual ○ New organism from 1 organism ○ Mitosis Sexual ○ Unique organism from 2 parents ○ Meiosis then fertilization Some of combination of asexual & sexual Parthenogenesis ○ Development of unfertilized egg into an adult organism without the contribution of sperm ○ Lizards, bees Two fold cost of sex ○ An asexual reproducing population will grow at twice the rate as a sexually reproducing one because members of a sexually reproducing population must produce both sexes ○ but only half of them can “give birth” to offspring of their own Evolution of sexual reproduction Karyotype Bird sex determination chromosomes are ZW (female) ZZ (male) Mammals have internal fertilization ○ Fish is not Egg sizes are also different per species Homologous chromosomes - pair of same chromosome ○ Sister chromatids are two replica held by centromere Mitosis and meiosis in human cells Mitosis: Mid Prophase – Chromosomes condense, becoming visible, and spindle fibers start forming. The nuclear envelope begins to break down. Metaphase – Chromosomes align at the metaphase plate, and spindle fibers attach to centromeres. Anaphase – Sister chromatids separate and are pulled toward opposite poles of the cell. Telophase – Chromosomes decondense, the nuclear envelope reforms, and two identical daughter cells are produced. Meiosis: Meiosis I: Prophase I (Leptotene) – Chromosomes start condensing, becoming visible as thin threads. Prophase I (Zygotene) – Homologous chromosomes begin pairing up (synapsis), forming tetrads. Prophase I (Pachytene) – Crossing over occurs, exchanging genetic material between homologous chromosomes. Prophase I (Diplotene/Diakinesis) – Homologs begin to separate but remain connected at chiasmata. Metaphase I – Homologous chromosomes align at the metaphase plate. Anaphase I – Homologous chromosomes (not sister chromatids) separate and move to opposite poles. Late Telophase I – Two haploid cells form, each with half the chromosome number. Meiosis II: Metaphase II – Chromosomes align at the metaphase plate again, similar to mitosis. Anaphase II – Sister chromatids are pulled apart toward opposite poles. Telophase II – Nuclear envelopes reform, and four haploid daughter cells are produced, each genetically unique. Chiasmata ○ Evidence of exchange between chromatids ○ Chiasmata form when programmed DNA breaks are repaired through recombination ○ Chiasmata link homologous chromosomes together until anaphase I ○ Chiasmata are essential for the segregation of homologous chromosomes during meiosis I ○ allow for the exchange of genetic material between homologous chromosomes ○ Chiasmata promote the attachment of sister chromatids to the same spindle pole ○ Chiasmata promote the co-segregation of sister chromatids during meiosis I ○ When chiasmata form ○ Chiasmata are a key feature of meiosis, particularly during meiosis I ○ Chiasmata are visible in the diplotene stage of meiotic prophase Male vs female meiosis Male Meiosis (Spermatogenesis) ○ Location: Testes (Seminiferous Tubules) ○ Start: Begins at puberty and continues throughout life ○ End Result: 4 haploid sperm cells per spermatogonium ○ Steps: ○ Mitosis of Spermatogonia: Diploid spermatogonia (2n) undergo mitosis, producing more spermatogonia. Some differentiate into primary spermatocytes (2n) ○ Meiosis I (Reduction Division): ○ Primary spermatocyte (2n) → undergoes Meiosis I → forms two secondary spermatocytes (n, haploid). ○ Meiosis II (Equational Division): Each secondary spermatocyte (n) undergoes Meiosis II → forming four spermatids (n) ○ Spermiogenesis (Maturation): Spermatids develop into mature spermatozoa (sperm) by acquiring a flagellum, condensing the nucleus, and losing excess cytoplasm Female Meiosis (Oogenesis) ○ Location: Ovaries ○ Start: Begins during fetal development (before birth), arrests, and resumes at puberty (with ovulation). ○ End Result: 1 mature ovum + 3 polar bodies per oogonium ○ Steps: ○ Mitosis of Oogonia (Fetal Life): Diploid oogonia (2n) proliferate via mitosis, producing primary oocytes (2n). These enter Meiosis I but arrest in prophase I (diplotene stage of prophase I) until puberty ○ Meiosis I (Puberty Onward): Each month, one primary oocyte completes Meiosis I before ovulation produces one secondary oocyte (n) and one polar body (n) (which degenerates) ○ Meiosis II (Ovulation and Fertilization): The secondary oocyte begins Meiosis II but arrests at metaphase II Meiosis II only completes if fertilization occurs → forms one ovum (n) and a second polar body (n). Meiosis I (Reductional Division) Prophase I – Homologous chromosomes pair up (synapsis), form tetrads, and crossing over occurs. Metaphase I – Homologous pairs align at the metaphase plate. Anaphase I – Homologous chromosomes (not sister chromatids) are pulled apart. Telophase I & Cytokinesis – Two haploid (n) cells form, each with duplicated chromosomes (sister chromatids still attached) Meiosis II (Equational Division) Prophase II – Chromosomes condense again. Metaphase II – Chromosomes line up at the metaphase plate. Anaphase II – Sister chromatids are separated. Telophase II & Cytokinesis – Four haploid (n) gametes form, each genetically unique. Meiotic errors can lead to non-disjunction ○ Aneuploidies Monosomies, trisomies Patu Microcephaly (small head size) Cleft lip and palate Heart defects Eye defects (e.g., small eyes, cataracts) Hand and foot deformities (e.g., clubbed feet) Brain and spinal cord abnormalities Seizures Feeding difficulties Edwards Low birth weight Small, abnormally shaped head Small jaw and mouth Clenched fists with overlapping fingers Heart, lung, kidney, intestine, and stomach defects Down Syndrome Small head, face, and ears Upward slanting eyes Flattened nasal bridge Short neck Small mouth and tongue Loose joints and poor muscle tone Single palmar crease (a line across the palm of the hand) Small hands and feet Short fingers Intellectual disability of varying severity, Delayed language development, Difficulty with memory and attention, and Challenges with problem-solving and decision-making. Other Health Issues: Heart defects (present in about 50% of cases) Thyroid problems Eye problems (such as nearsightedness or farsightedness) Hearing loss Increased risk of infections Digestive problems Sleep disturbances Totipotency - Pluripotency A nucleus that is totipotent can produce all the different cells in the body of an adult ○ In mammals, this includes the cells that make the placenta. A nucleus that is pluripotent can produce many of the cells in the body of an adult Summary Sexual reproduction is the formation of different individuals from two parents Asexual reproduction depends mitosis, while sexual reproduction depends on meiosis for gamete production Meiosis is the process that produces haploid gametes Crossing-overs (chiasmata; genetic recombination) contributes to genetic diversity The maternal and paternal nuclei divide symmetrically. The zygote contains totipotent and pluripotent cells in different stages of its development ○ Cells transition from totipotent to pluripotent during the early stages of embryonic development ○ around the blastocyst stage, when the embryo is comprised of a distinct inner cell mass (ICM) which will give rise to the embryo itself ○ outer layer (trophoblast) develops into the placenta and other extraembryonic tissues ○ occurs around day 4-5 after fertilization in humans Lecture 5 - Germ Cell Development Germ cells are cells that give rise to male gametes (spermatozoa) & female gametes (oocytes) Oocyte, spermatozoon: fully mature germ cells Zygote = soma Female reproductive organs ○ Germ cell = oocyte in ovary ○ Oocyte = egg in ovary Male reproductive organs ○ Germ cell = spermatozoa ○ In testis Role of germ cell: give rise to new organism Interaction of germ & somatic cells 1. Fertilization The sperm fuses with the oocyte, forming a zygote (a single diploid cell). This triggers cortical reactions to prevent polyspermy. The zygote begins metabolic activation to start development. 2. Cleavage The zygote undergoes rapid mitotic divisions without increasing in size. This forms smaller cells called blastomeres. By the 8-cell stage, cells undergo compaction, increasing cell-cell adhesion. 3. Morula Stage (~Day 3-4) A solid ball of 16-32 blastomeres forms. Cells differentiate into an outer layer (trophoblast, contributing to placenta) and inner cell mass (embryoblast, forming the embryo). 4. Blastocyst Stage (~Day 5-6) The morula develops a fluid-filled cavity (blastocoel). The outer trophoblast layer facilitates implantation. The inner cell mass differentiates into the epiblast and hypoblast. 5. Implantation (~Days 6-10) The blastocyst attaches to the uterine wall. Trophoblast cells invade the endometrium, forming the syncytiotrophoblast and cytotrophoblast. hCG secretion begins, maintaining the corpus luteum for progesterone production. 6. Bilaminar Disc Formation (~Week 2) The inner cell mass differentiates into two layers: ○ Epiblast → Forms amniotic cavity and future embryo. ○ Hypoblast → Contributes to the yolk sac. 7. Gastrulation (~Week 3) The primitive streak forms, marking the beginning of germ layer differentiation. Three germ layers develop: ○ Ectoderm → Skin, nervous system. ○ Mesoderm → Muscles, bones, cardiovascular system. ○ Endoderm → Gut, liver, lungs. Primordial Germ Cell formation and migration into the Gonadal Ridges 1. Specification of PGCs (~Week 2-3 in Humans) PGCs originate from the epiblast during early gastrulation. Inductive signals, including Bone Morphogenetic Proteins (BMP4 and BMP8b) from the extraembryonic tissues, initiate PGC specification. The specified PGCs express key transcription factors such as BLIMP1 (PRDM1), PRDM14, and TFAP2C, which suppress somatic differentiation and maintain pluripotency. 2. Migration from the Yolk Sac (~Week 4-6 in Humans) Initially, PGCs migrate to the posterior primitive streak and settle in the extraembryonic mesoderm of the yolk sac, near the allantois. This transient location protects them from differentiation signals that could induce somatic fate. 3. Directed Migration to the Gonadal Ridges (~Week 5-6) PGCs migrate along the hindgut and through the dorsal mesentery toward the developing gonadal ridges. This movement is guided by chemotactic signals, particularly: ○ SDF-1 (Stromal-Derived Factor-1) secreted by the gonadal ridges. ○ CXCR4 (receptor on PGCs) that allows directional migration. Proper migration requires the interaction of extracellular matrix components (e.g., fibronectin) and adhesion molecules (e.g., integrins). 4. Colonization of the Gonadal Ridges (~Week 6-7) Once PGCs reach the gonadal ridges, they proliferate rapidly. They interact with somatic cells of the gonads, leading to sex-specific differentiation: ○ Males: PGCs are surrounded by Sertoli cells and enter mitotic arrest as spermatogonia. ○ Females: PGCs enter meiosis and arrest in prophase I as oogonia. Clinical Relevance Defects in PGC migration can result in conditions such as germ cell tumors (e.g., teratomas) or gonadal dysgenesis (e.g., Turner syndrome). Posterior Primitive Streak: Definition and Role in Development The posterior primitive streak is a specialized region of the primitive streak, a structure that forms early in embryonic development during gastrulation. It plays a critical role in cell migration, germ layer formation, and axial patterning. 1. Location & Formation Appears at the caudal (posterior) end of the developing embryo. Forms from the epiblast around day 14-15 in humans. Extends from the posterior towards the midline as cells ingress and move inward. 2. Function in Gastrulation Epiblast cells migrate through the primitive streak and differentiate into three germ layers: ○ Ectoderm (forms skin and nervous system) ○ Mesoderm (forms muscles, bones, and circulatory system) ○ Endoderm (forms gut and internal organs) The posterior primitive streak is particularly important for mesoderm formation, including: ○ Extraembryonic mesoderm (supports early development). ○ Paraxial, intermediate, and lateral plate mesoderm (which form somites, kidneys, and other structures). 3. Role in Primordial Germ Cell (PGC) Development Primordial Germ Cells (PGCs) originate from epiblast cells that migrate to the posterior primitive streak. They are specified under the influence of BMP4 and BMP8b, signaling molecules from the extraembryonic ectoderm. PGCs then leave the primitive streak and migrate to the yolk sac wall near the allantois, a temporary safe zone where they avoid somatic differentiation before migrating to the gonadal ridges. 4. Clinical Relevance Defects in primitive streak formation can lead to congenital disorders such as caudal dysgenesis (e.g., sirenomelia or "mermaid syndrome"). Abnormal migration of PGCs from the primitive streak can result in germ cell tumors or infertility. Mouse primordial germ cells during early embryo development Primordial germ cell migration regulated by attractive & repulsive cues Primordial Germ Cell (PGC) Migration: Regulation by Attractive & Repulsive Cues Primordial germ cells (PGCs) undergo a highly regulated migration process from their site of origin in the posterior primitive streak to the gonadal ridges, where they will form the future gametes. This migration is controlled by a delicate balance of attractive and repulsive molecular cues to ensure they reach the correct location while avoiding incorrect differentiation or apoptosis. 1. Key Stages of PGC Migration 1. Specification (Epiblast → Posterior Primitive Streak) ○ PGCs are specified by BMP4 and BMP8b signaling from the extraembryonic ectoderm. ○ They arise from epiblast cells and migrate to the posterior primitive streak. 2. Migration to the Yolk Sac ○ PGCs move out of the primitive streak and settle temporarily in the yolk sac near the allantois. ○ This step protects PGCs from somatic differentiation signals. 3. Guided Migration to the Gonadal Ridges ○ Around week 4 in humans, PGCs re-enter the embryo and migrate through the hindgut and dorsal mesentery toward the developing gonads. ○ By week 6, they colonize the gonadal ridges (future testes or ovaries). 2. Molecular Cues Regulating PGC Migration PGC migration is controlled by a combination of attractive (chemotactic) and repulsive (chemorepulsive) signals. (A) Attractive Cues: "Guiding the PGCs to the Gonads" These cues pull PGCs toward their correct destination. SDF-1 (Stromal Cell-Derived Factor 1) ○ Key chemokine expressed in the gonadal ridges. ○ Binds to CXCR4 receptors on PGCs, directing them toward the gonads. ○ Essential for proper homing of PGCs. ○ Loss of SDF-1/CXCR4 signaling leads to mislocalized PGCs and infertility. SCF (Stem Cell Factor) & c-Kit ○ SCF is expressed along the migratory path, while c-Kit is a receptor on PGCs. ○ SCF helps promote survival and proliferation of migrating PGCs. ○ Mutations in c-Kit (e.g., in W mutant mice) result in PGC loss and sterility. (B) Repulsive Cues: "Preventing Wrong Migration" These signals prevent PGCs from straying into incorrect tissues. BMP Signaling (BMP2, BMP4) ○ Ensures PGCs stay in a migratory, undifferentiated state. ○ Helps regulate the balance of attraction and repulsion. Ephrin/Eph Receptors ○ Ephrin ligands repel PGCs from inappropriate tissues. ○ This prevents ectopic PGC migration, which could lead to teratomas (germ cell tumors). ECM (Extracellular Matrix) Barriers ○ Components like fibronectin and laminin help shape the migration path. ○ Integrins on PGCs interact with ECM molecules to navigate correctly. 3. Consequences of Disrupted PGC Migration If the balance of attraction and repulsion is disrupted: PGCs may fail to reach the gonads → Leads to infertility. PGCs may colonize ectopic sites (e.g., liver, adrenal glands) → Can lead to germ cell tumors like teratomas. Conclusion PGC migration is a highly coordinated process involving SDF-1/CXCR4 attraction and Ephrin repulsion to ensure correct positioning. Disruptions in these signals can result in infertility or germ cell tumors. Male gonad differentiation 6 weeks in humans, male embryo, SRY gene ○ Vigorous proliferation of the sex cord cells into gonad primordial germ cells are the precursor cells that will become sperm or eggs, while sex cord cells are the supporting cells that form the structure of the gonad, essentially providing the framework for the developing germ cells to reside within ○ Form testis cords ○ Organize PGCs into columns & make outer basement membrane ○ Seminiferous tubules in adult Prospermatogonia, sertoli & leydig cells Female gonad differentiation 6 weeks, female embryo Some cortical cords Germ cells called oogonia Small cell clusters ○ Oogonia + somatic cells = primordial follicles ○ Somatic cells = granulosa cells Spermatogenesis Spermatogenesis: Step-by-Step Breakdown Spermatogenesis is the process of sperm cell development occurring in the seminiferous tubules of the testis. It takes ~64 days in humans and involves mitosis, meiosis, and spermiogenesis, leading to mature sperm (spermatozoa). 1. Spermatogonial Phase (Mitosis) → Stem Cell Renewal & Proliferation Occurs in the basal compartment of the seminiferous tubules. Begins with spermatogonial stem cells (SSCs), which undergo mitosis to produce a pool of dividing cells. Types of spermatogonia: ○ Type A (dark & pale) → Self-renewing stem cells. ○ Type B → Differentiate into primary spermatocytes and enter meiosis. 🔹 Key Event: Spermatogonia undergo mitotic divisions, ensuring a continuous supply of sperm precursors. 2. Meiotic Phase (Meiosis I & II) → Reduction of Chromosome Number (A) Meiosis I: Primary Spermatocyte → Secondary Spermatocyte Primary spermatocytes (2n, diploid) enter meiosis I. Homologous chromosomes pair and undergo crossing over (genetic recombination) in prophase I. At the end of meiosis I, two haploid secondary spermatocytes (n, haploid) are formed. 🔹 Key Event: Genetic variation is introduced through recombination. (B) Meiosis II: Secondary Spermatocyte → Spermatid Secondary spermatocytes (n, haploid) rapidly enter meiosis II. Sister chromatids separate, forming haploid spermatids (n, haploid). No further division occurs after this step. 🔹 Key Event: Each secondary spermatocyte produces two spermatids, totaling four spermatids per primary spermatocyte. 3. Spermiogenesis (Maturation of Spermatids) → Morphological Changes Spermatids transform into mature spermatozoa by undergoing several morphological changes: 1. Condensation of Nucleus → DNA is tightly packed. 2. Acrosome Formation → Golgi apparatus forms the acrosome, which contains enzymes for egg penetration. 3. Flagellum Development → Centriole forms the tail for motility. 4. Cytoplasm Reduction → Excess cytoplasm is removed by Sertoli cells. 5. Mitochondrial Arrangement → Mitochondria migrate to the midpiece, providing ATP for movement. 🔹 Key Event: Spermatids become highly motile spermatozoa but are still non-functional until capacitation. 4. Spermiation (Release into Lumen) Mature spermatozoa are released from Sertoli cells into the lumen of the seminiferous tubules. Transported to the epididymis, where they gain motility and fertilization ability. Hormonal Regulation of Spermatogenesis 1. GnRH (Hypothalamus) → Stimulates release of FSH & LH. 2. LH (Anterior Pituitary) → Stimulates Leydig cells to produce testosterone. 3. FSH (Anterior Pituitary) → Stimulates Sertoli cells to support sperm development. 4. Testosterone (Leydig Cells) → Essential for spermatogenesis progression. 5. Inhibin (Sertoli Cells) → Negative feedback to regulate FSH levels. Summary of the Steps Step Cell Type Ploidy Process Key Outcome Spermatogonia Stem cells 2n Mitosis Self-renewing pool of (Diploid) cells Primary Pre-meiotic 2n Meiosis I Two haploid secondary Spermatocyte (Diploid) spermatocytes Secondary Post-meiotic n Meiosis II Four haploid spermatids Spermatocyte (Haploid) Spermatids Round, n Spermiogenes Morphological maturation immature (Haploid) is Spermatozoa Fully n Spermiation Functional sperm developed (Haploid) released Complete Overview of Spermatogenesis & Sperm Movement in the Male Reproductive Tract Spermatogenesis occurs inside the seminiferous tubules and is followed by sperm transport through various parts of the male gonad and reproductive tract before ejaculation. 1. Spermatogenesis (Occurs in the Seminiferous Tubules) Location: Seminiferous tubules of the testis. Process: Begins at the basal side of the seminiferous tubules and moves toward the lumen. Cell types involved: ○ Spermatogonia (stem cells) divide mitotically. ○ Primary spermatocytes undergo meiosis I. ○ Secondary spermatocytes undergo meiosis II. ○ Spermatids mature into spermatozoa. Support Cells: ○ Sertoli cells → Provide nourishment & remove excess cytoplasm. ○ Leydig cells → Secrete testosterone for spermatogenesis. 🔹 Key movement: Developing sperm move from the basement membrane → adluminal compartment → lumen of seminiferous tubules. 2. Sperm Transport in the Male Reproductive Tract (A) Seminiferous Tubules → Rete Testis Spermatozoa are released into the lumen of the seminiferous tubules but are non-motile. They are passively moved through fluid flow (produced by Sertoli cells) toward the rete testis. (B) Rete Testis → Efferent Ductules Rete testis: A network of tubules where sperm start to concentrate. Efferent ductules: Ciliated cells help move sperm into the epididymis. 🔹 Key function: Fluid absorption concentrates sperm. (C) Epididymis (Head → Body → Tail) Function: Sperm mature and gain motility. Takes ~12 days to pass through the epididymis. Regions: ○ Caput (Head): Initial concentration and absorption of testicular fluid. ○ Corpus (Body): Sperm undergo biochemical changes, improving motility. ○ Cauda (Tail): Sperm storage site until ejaculation. 🔹 Key function: Sperm maturation (gain motility but are still immotile in the female tract). (D) Vas Deferens Muscular contractions propel sperm from the epididymis into the ejaculatory duct. Stores sperm for a short period. (E) Ejaculatory Duct & Urethra The seminal vesicles, prostate, and bulbourethral glands add seminal fluid. During ejaculation, sperm are transported through the prostatic urethra → penile urethra and expelled. Sperm Movement Summary Structure Function Seminiferous Sperm production (spermatogenesis) Tubules Rete Testis Initial sperm concentration Efferent Ductules Move sperm to epididymis Epididymis Sperm maturation and storage Vas Deferens Sperm transport during ejaculation Ejaculatory Duct Mixes sperm with seminal fluid Urethra Pathway for sperm exit during ejaculation Female germ cell development Middle-late fetal life (human): follicle formation & meiosis entry Follicle arrest: oocytes are in prophase of meiosis 1 & granulosa cells are flat ○ Flare epithelial granulosa cells don’t divide Primary oocyte + granulosa cells = primordial follicle ○ Doesn’t grow ○ Thousands of primordial follicles wit until adult life & hormone activation ○ Primordial follicles are stockpile of oocyte for life Oocyte Development to Ovulation: A Step-by-Step Overview Oocyte development, also known as oogenesis, occurs in the ovary and follows a complex timeline, beginning before birth and continuing until ovulation (and potentially fertilization). Below is a breakdown of the process from fetal development to ovulation. 1. Fetal Development: Formation of Primordial Germ Cells & Oogonia Primordial germ cells (PGCs) migrate to the developing gonadal ridges (~week 4–6 of embryonic development). PGCs differentiate into oogonia, which proliferate via mitosis. By mid-gestation (~20 weeks), all oogonia enter prophase I of meiosis and become primary oocytes. Meiosis is arrested in prophase I (diplotene stage)—this is known as the dictyate stage, and the oocytes remain here until puberty. 🔹 At birth, the female has ~1–2 million primary oocytes arrested in prophase I. 2. Puberty & Folliculogenesis: Growth & Maturation of the Oocyte With each menstrual cycle, a cohort of follicles is recruited, but only one follicle fully matures and is ovulated. (A) Primordial Follicle → Primary Follicle Primordial follicle: Primary oocyte surrounded by a single layer of flat granulosa cells. During puberty, some primordial follicles begin developing into primary follicles, characterized by: ○ Growth of granulosa cells (cuboidal shape). ○ Formation of the zona pellucida (glycoprotein shell surrounding the oocyte). (B) Secondary Follicle Granulosa cells proliferate, and the theca cells form around the follicle. Theca cells produce androgens, which granulosa cells convert into estrogen via aromatase. Estrogen supports follicular development and endometrial thickening. (C) Tertiary (Antral) Follicle Fluid-filled antrum forms within the follicle. Estrogen production increases significantly. Only one dominant follicle is selected for ovulation, while others undergo atresia. (D) Preovulatory (Graafian) Follicle & Completion of Meiosis I LH surge triggers: ○ Completion of meiosis I, forming a secondary oocyte and a polar body. ○ Secondary oocyte enters meiosis II and arrests in metaphase II. 🔹 Oocyte is now ready for ovulation but will only complete meiosis II if fertilized. 3. Ovulation: Release of the Oocyte from the Ovary LH surge (~36 hours before ovulation) causes follicular rupture. The secondary oocyte (still in metaphase II) is released from the ovary. The fimbriae of the fallopian tube sweep the oocyte into the ampulla, where fertilization can occur. Summary of Oocyte Development & Ovulation Stage Key Events Fetal Stage PGCs → Oogonia → Primary Oocytes (arrest in prophase I) Puberty Onset Folliculogenesis begins (primordial follicle recruitment) Primary Follicle Granulosa cells grow, zona pellucida forms Secondary Follicle Theca cells produce androgens, granulosa cells make estrogen Tertiary (Antral) Fluid-filled antrum forms, dominant follicle selected Follicle Preovulatory Follicle LH surge triggers completion of meiosis I → secondary oocyte (arrested in metaphase II) Ovulation Oocyte released into fallopian tube, ready for fertilization Reproductive cloning The production of a genetically identical organism by somatic cell nuclear transfer (SCNT) A nucleus from a somatic cell is removed and then transferred to an enucleated oocyte The embryo is transferred to a surrogate mother for gestation Dolly Therapeutic cloning The production of embryonic stem cells using somatic cell nuclear transfer (SCNT) for medical treatments The stem cells produced will have the potential to differentiate into many different cell types to replace diseased ones Ethical dilemma Same process as SCNT Induced pluripotent stem cells Takahasi and Yamanaka (2006, 2007) induced adult mouse and human fibroblasts (cultured skin cells) to behave like embryonic cells by the introduction of four factors controlling pluripotency ○ C-myc, Oct4, Sox2, Klf4 They called these cells iPS cells, induced pluripotent stem cells Using iPS cells for patient replacement therapy avoids the ethical issues associated with using human embryos iPSCs can be reprogrammed from somatic cells (like skin or blood cells) and then differentiated into kidney organoids Summary of germ cells Genomic imprinting Maternal and paternal mammalian genomes are not equivalent Some genes have different patterns of expression. ○ Usually, one is silent while the other one is active This parental-specific gene expression is called genomic imprinting Overview of Genetic Imprinting in the Embryo Genetic imprinting is an epigenetic process where certain genes are expressed in a parent-of-origin-specific manner. This means that for some genes, only the allele inherited from either the mother or the father is active, while the other allele is silenced through DNA methylation and histone modifications. Key Features of Genetic Imprinting in the Embryo 1. Epigenetic Marks Are Established in Germ Cells ○ During gametogenesis (sperm and egg formation), DNA methylation patterns are set according to the sex of the parent. ○ These imprints are maintained after fertilization and throughout embryonic development. 2. Essential for Normal Development ○ Imprinted genes play critical roles in regulating growth, placental development, and metabolism. ○ Many imprinted genes are involved in nutrient acquisition from the mother, ensuring proper fetal development. 3. Parental Conflict Hypothesis ○ Evolutionarily, paternal genes tend to promote fetal growth, while maternal genes limit growth to balance resources for both the mother and offspring. ○ Example: IGF2 (Insulin-like Growth Factor 2) is paternally expressed and promotes fetal growth. IGF2R (Insulin-like Growth Factor 2 Receptor) is maternally expressed and limits growth by degrading IGF2. 4. Key Imprinted Gene Clusters ○ Chromosome 11p15 (IGF2/H19 locus) → regulates fetal growth. ○ Chromosome 15q11-q13 (Prader-Willi/Angelman region) → involved in neurological development. 5. Disorders Associated with Imprinting Defects ○ Prader-Willi Syndrome (PWS) → Loss of paternal genes at 15q11-q13 leads to developmental and metabolic issues. ○ Angelman Syndrome (AS) → Loss of maternal UBE3A gene at 15q11-q13 causes severe neurological impairment. ○ Beckwith-Wiedemann Syndrome (BWS) → Overexpression of IGF2 due to improper imprinting leads to overgrowth and cancer risk. Lecture 6 - Endocrine Disruptors Endocrine-disrupting chemicals are substances in the environment that interfere with endocrine signaling In insecticides, herbicides, fumigants, fungicides, and many other sources EDCs can cause the endocrine system of an animal to over- or underproduce a hormone, or simply mimic the hormone itself Altering the delicate chemical balance in the body can cause changes in a variety of behaviors, including sexual and reproductive behaviors, communication, movement, social behaviors, and cognition Molecular mechanisms of endocrine disruption ○ Mimic or block hormone action ○ Alter production, metabolism or secretion ○ Alter transport/ carrier protein binding or levels ○ Alter receptor levels ○ Alter receptor DNA binding or transcriptional activation ○ Epigenetic alterations Environmental endocrine disruptors ○ Water contaminated with dicofol, DDT, industrial chemicals ○ Reduced survival rates & egg hatching ○ Led to alligators having combo of male and female characteristics ○ Abnormal ovaries & testis, small penis ○ Males with elevated estrogen & females with elevated testosterone Effects of endocrine disruption ○ Interference with reproduction ○ Developmental malformations ○ Increased cancer risk ○ Disturbances in immune & nervous system Diethylstilbestrol (DES) ○ Between the 1940s and 1960s, millions of women were treated with DES ○ Correlation between DES (active orally) exposure in mothers and the occurrence of adenocarcinoma of the vagina reported in 1971 ○ High incidence of anatomic abnormalities of the genital tract that adversely affected their reproductive capacity ○ Women developed young vaginal cancer ○ High # of DES related reproductive abnormalilites DES & Mullerian development Disrupted Müllerian Duct Differentiation The Müllerian (paramesonephric) ducts give rise to the fallopian tubes, uterus, cervix, and upper vagina in female fetuses. DES exposure disrupts the normal estrogen signaling required for proper differentiation and fusion of the Müllerian ducts. This results in abnormal uterine shapes and structural defects. Common Müllerian Anomalies Associated with DES Exposure T-shaped Uterus: Narrow, hypoplastic endometrial cavity, leading to infertility and pregnancy loss. Arcuate or Hypoplastic Uterus: Incomplete development of the uterus, increasing miscarriage risk. Cervical Incompetence: Weakened cervix leading to preterm labor. Vaginal Adenosis: Presence of glandular epithelium in the vagina, increasing risk of clear cell adenocarcinoma of the vagina and cervix. Increased Risk of Infertility & Pregnancy Complications Women exposed to DES in utero often experience miscarriages, ectopic pregnancies, and infertility due to structural abnormalities. Higher risk of preeclampsia, preterm birth, and stillbirth. Epigenetic & Transgenerational Effects DES exposure alters gene expression in the reproductive tract, leading to long-term dysfunction. Some studies suggest second-generation effects, where daughters of DES-exposed women may also experience reproductive abnormalities. DES & HOX Genes Present in all Multicellular Animals Highly Conserved Between Species Encode transcription factors Essential Role in Embryonic Axial Developmental Patterning All estrogens increase uterine size in adults DES induces uterine HOXA10 expression ○ Effects of DES on HOXA10 Expression: ○ In Human Endometrial Cells: Short-term exposure to DES increases HOXA10 expression. ○ In Mice Exposed In Utero: DES exposure leads to hypermethylation of the Hoxa10 gene, resulting in reduced expression and an abnormal spatial distribution of Hoxa10 in the uterus ○ Therefore, the effect of DES on HOXA10 expression is context-dependent: ○ Short-Term Exposure: Increases HOXA10 expression ○ In Utero Exposure: Leads to epigenetic modifications (hypermethylation) that decrease HOXA10 expression Alters HOX axis T-shaped uterus (due to disrupted HOXA10 & HOXA11) Infertility and implantation failure Cervical incompetence → Increased risk of preterm labor Vaginal adenosis & cancer (HOXA13 dysregulation) ○ Vaginal adenosis is a condition where cells that are normally found in the cervix or uterus (endometrium) grow in the vagina ○ Cells can’t survive here & will form cancer Ectopic pregnancies (HOXA9 disruption) DES acts as morphogen DES exposure alters developmental programming DES exposure in utero permanently changes expression of Hox genes These changes in gene expression result in altered developmental identity Diethylstilbestrol (DES) as a Morphogen A morphogen is a signaling molecule that diffuses through tissues in a concentration-dependent manner to regulate gene expression and developmental patterning. DES acts as a morphogen by disrupting estrogen-responsive gene expression, particularly in the reproductive tract, through both direct and epigenetic mechanisms. 1. DES Mimics Estrogen and Alters Morphogen Gradients DES is a synthetic estrogen that binds to estrogen receptors (ERα and ERβ), leading to aberrant activation of estrogen-responsive genes. During fetal development, natural estrogen gradients regulate differentiation and organization of the Müllerian ducts into the uterus, cervix, and vagina. DES disrupts these gradients, leading to abnormal tissue differentiation and malformations. 2. DES Disrupts HOX Gene Patterning HOX genes (especially HOXA9–HOXA13) establish anterior-posterior patterning in the Müllerian ducts. Normal Development: ○ HOXA9 → Fallopian tubes ○ HOXA10 → Uterus ○ HOXA11 → Lower uterus ○ HOXA13 → Cervix & Vagina DES Exposure: ○ Alters HOX gene expression timing and spatial distribution, leading to: T-shaped uterus (HOXA10 misregulation). Cervicovaginal malformations (HOXA13 disruption). Vaginal adenosis (persistence of glandular tissue). 3. DES Acts as an Epigenetic Morphogen Alters DNA Methylation and Histone Modifications: ○ HOXA10 becomes hypermethylated, reducing its expression in the uterus. ○ Aberrant histone acetylation affects estrogen-responsive genes, leading to permanent developmental changes. Long-Term Effects on Fertility & Cancer Risk: ○ Persistent epigenetic changes contribute to infertility, pregnancy loss, and increased risk of clear cell adenocarcinoma in DES-exposed individuals. Summary: How DES Acts as a Morphogen Mechanism Effect Mimics Estrogen Disrupts estrogen gradients needed for reproductive tract differentiation. Alters HOX Gene Misregulates HOXA9–HOXA13, causing uterine and vaginal Expression malformations. Epigenetic Induces DNA methylation & histone changes, leading to Modifications long-term effects on gene expression. DES changes where genes are expressed (black bar graphs) EDC exposure Methoxychlor Weak estrogen in adults DMSO = dimethyl sulfoxide Neonatal MXC decreases adult Hoxa10 expression Neonatal MXC exposure on adult Hoxa10 expression in vivo: Bisphenol A Endocrine disruptor Weakly estrogenic Stimulates uterine growth 10^-4 that of estradiol in adult animals In hard plastic water bottles (lined with BPA bc it makes them rigid, tin, dentist? In vitro, or in adult, BPA is like weak estrogen But distinct from DES, in utero exposure increased Hoxa10 ○ Leads to demethylation of Hoxa10 ER, enhanced ER binding & increased transcriptional activity ○ ERE= estrogen response element Humans widely exposed ○ In highest level orders: placenta, maternal blood, fetal blood While many xenoestrogens (estrogen mimics) appear to increase HOXA10 expression in the adult and vary only in potency, their effects are paradoxical when exposure occurs in utero Persistent effects long after exposure Not simply an estrogen agonist/antagonist effect Epigenetic alterations Possible explanation for paradoxical affects DNA methylation ○ Methyl marks added to certain DNA bases to repress gene activity Histone modification ○ Combination of different molecules can attach to the tails of histones ○ Alter DNA activity Euchromatin - activation Heterochromatin - silenced Developmental programming of estrogen sensitivity Selective methylation of EREs Dual Mechanism of Action “Classic” estrogen action in the adult and in vitro DES is a strong estrogen Endocrine disruptors are often “weak estrogens.” Altered developmental programming through epigenetic alterations ○ Does not necessarily depend on estrogenicity Are there naturally occurring estrogens that play a role in epigenetic programming? What do fetal estrogens tell us about endocrine disruption? E3 E1, E2, E3 pathways E3 Effect on reproduction Estriol (E3) and Its Effects on Reproduction Estriol (E3) is a weak estrogen primarily produced during pregnancy by the placenta. Unlike estradiol (E2) and estrone (E1), E3 has a lower binding affinity for estrogen receptors and plays a distinct role in pregnancy maintenance, fetal development, and maternal physiology. 1. Estriol in Pregnancy A. Source and Production Non-pregnant state: Very low levels, primarily from the liver. Pregnancy: Produced in large amounts by the placenta using fetal adrenal precursors (DHEA-S). B. Functions During Pregnancy Promotes Uteroplacental Blood Flow → Supports fetal growth. Prepares the Myometrium for Labor → Increases oxytocin receptor expression. Stimulates Mammary Gland Development → Prepares for lactation. Regulates Immune Tolerance → Prevents maternal rejection of the fetus. 2. Estriol and Fertility Limited Role in Ovulation: ○ Unlike estradiol (E2), E3 has a weak effect on the hypothalamic-pituitary-ovarian (HPO) axis and does not significantly trigger LH surges for ovulation. Endometrial Effects: ○ May promote limited endometrial growth, but not enough to fully support implantation like E2. 3. Clinical Uses and Effects on Reproduction A. Estriol as a Biomarker in Pregnancy Low Estriol Levels may indicate placental insufficiency, fetal distress, or Down syndrome (as part of the triple/quad screen). B. Estriol Therapy Used in Hormone Replacement Therapy (HRT) → Mainly for postmenopausal women to reduce vaginal atrophy and urinary symptoms (without strong systemic estrogenic effects). May Be Used in Infertility Treatment → Occasionally in estrogen priming protocols to improve endometrial receptivity. 4. Estriol vs. Other Estrogens in Reproduction Feature Estriol (E3) Estradiol (E2) Estrone (E1) Potenc Weakest Most potent Intermediate y Main Pregnancy Ovulation & cycle Postmenopa Role maintenan regulation usal ce estrogen Effect Minimal Essential for Limited on impact ovulation & Fertility implantation Conclusion Estriol (E3) is primarily important during pregnancy, supporting placental function, labor, and fetal well-being. Unlike estradiol (E2), E3 has a weaker effect on ovulation and fertility. Clinically, estriol is used in pregnancy monitoring and menopausal therapy. E3 also hypo-methylated 2,252 genes and hypermethylated 2,620 genes Also affects behaviour - less anxiety, more exploring? Also changes gene expression on brain ○ Changes methylation patterns E3 in mice ○ Higher number of pregnant mice ○ Good pups & gestation period ○ No effect on uterus physiology ○ But did change gene expression in adults by changing methylation ○ E3 changed brain gene methylation Epigenetic modifiers bind directly to ER (estrogen receptor) in a ligand dependant fashion ○ E3 binds to epigenetic modifier, altering epigenetic programming ○ LSD 1: Lysine specific demethylase; SUZ12: Suppressor of Zeste; CBP: CREB-binding protein; DNMT: DNA methyltransferase Nuclear receptor signaling ○ Selective estrogen receptor modulators (SERMs) Fetal Estrogens are SERMs that activate Epigenetic Programming Model of E3 action Conclusions: The normal role of E3 in pregnancy includes epigenetic programming Endocrine disruption alters the developmental programming of the female reproductive tract through lasting epigenetic alterations Xenoestrogens interfere with E3 action Endocrine disruptor Phthalates Endocrine disruptor Atrazine Endocrine disruptor Organophosphate Organophosphates are pesticides that target the CNS of insects Linked to brain development and reproductive defects Can alter testosterone and thyroid signaling Ways to avoid: ○ Limit your exposure to all pesticides ○ Buy organic foods Endocrine disruptor Perfluorinated chemicals Lecture 7 - Steroid hormones Hormones serve as a major form of communication between different organs and tissues Hormones allow specialized cells to respond in a coordinated manner to changes in the internal or external environments Classes of hormones: Steroid ○ Estrogens, ie ○ Made from cholesterol ○ Bind intracellular to receptors ○ Not coded in genome directly Peptide ○ Made from amino acids ○ Bind to receptors on cell surface because can’t through membrane Monamines ○ Ie adrenaline Steroid function ○ Progestogens Ie progesterone Needed for implantation, maintenance of pregnancy Made by corpus luteum & placenta ○ Androgens Ie testosterone Needed for development for male secondary sex characteristics Mainly made in testis ○ Estrogens Estradiol ie Needed for development of female secondary sex characteristics Mainly made in ovary ○ Glucocorticoids Cortisol ie Needed for adaptation to stress Made in adrenal cortex Inner regions: zona reticularis, zona fasciculata ○ Mineralocorticoids Aldosterone ie Needed for reabsorption of Na+ & excretion of K+ & H+ in kidneys Made in adrenal cortex (outer region: zona glomerulosa) Steroids: synthesis is dynamic process of enzymatic conversions Regulation of steroid synthesis is organ specific ○ Based on available enzymes ○ Can be made in 1 organ, modified in another to be more or less potent Hormone receptors in target tissues needed to promote their response ○ Target organs regulate effect of hormone by changing the amount receptor All steroid hormones derived from cholesterol ○ Chlosteron = 4 fused hydrocarbon rings & hydrocarbon tail (27 carbons) ○ Small and Lipophilic, Hydrophobic; ○ steroid hormones can pass through the plasma membranes and enter the cell (contrast with protein hormones which can’t pass through the cell and elicit response through membrane receptors ○ Steroid hormones are NOT encoded in the genome derived from dietary, environmental, and metabolic precursors Cholesterol sources ○ Endogenous: Cholesterol is synthesized from acetate by virtually all tissues in humans (HMG CoA reductase) Liver, intestine, adrenal cortex, and reproductive organs make the largest contributions to cholesterol pool ○ Diet: cholesterol from animal source PROGESTERONE IS LARGEST OF STEROIDS Adrenal gland, ovary, testes, & placenta have the enzymes to make reproductive steroid hormones ○ Hypothalamus & pituitary glands are master regulators of glands Hypothalamic-Pituitary-Adrenal (HPA) Axis and Steroid Hormone Biosynthesis ○ Cortisol down regulates CRH (Corticotropin-releasing hormone)when cortisol levels are high ○ Normally: Corticotropin-Releasing Hormone (CRH) directly stimulates the release of cortisol, acting as the primary trigger in the stress response pathway by signaling the pituitary gland to produce Adrenocorticotropic Hormone (ACTH), which then prompts the adrenal glands to secrete cortisol; essentially, CRH is the "upstream" hormone that initiates the cascade leading to cortisol production. ○ Block ACTH - feedback loop shifts down Enzymatic Inter-conversion of Steroid Hormones: Hydroxylation and Clipping ○ Steroid Hormones are synthesized in a series of chemical modifications by specific enzymes which hydroxylate (cytochrome P450) and clip the cholesterol structure Estradiol biosynthesis (aromatase enzyme) ○ Aromatase creates an aromatic (phenolic) A-ring in the steroid nucleus - removes the C-19 methyl group Only enzyme in mammals that can synthesize aromatic ring ○ Ovary has reductive 17B-dehydrogenase that’s specific for estrogens & synthesizes estradiol Adipose tissue (present in overweight or post-menopausal people) has aromatase but not 17B-HSD ○ Estrone is the predominant estrogen in postmenopausal women from adrenal production of androstenedione and adipose conversion to estrone ○ For people with obesity (30-60% of body weight is adipose), estrone levels are high and can have significant estrogenic effects (ex. Gynecomastia/breast development in men) Enzyme deficiencies can cause disorders of sexual maturation ○ Congenital Adrenal Hyperplasia Altered pathway of steroid biosynthesis in adrenal cortex ○ 5α-Reductase Deficiency (Androgen Insensitivity) Androstenedione & testosterone are produced by the adrenal gland, and converted to more potent DHT at target organs by enzyme Baby boys, 46XY, may be born with ambiguous genitalia due to lack of virilization in utero At puberty, large amounts of testosterone are made, and sexual maturation occurs Described by Dr. Imperato-McGinley (Cornell) in 1974, observing a cohort of families in the Dominican Republic Hormone activity can be regulated by controlling receptor expression and activity ○ A hormone can not promote a response in the absence of its cognate receptor ○ Receptor expression can vary in different tissues Structural organization of nuclear receptors Testosterone: Mechanism of Action ○ 1. Testosterone passes across cell lipid membrane of a cell ○ 2. Testosterone is converted to more potent Dihydrotestosterone by 5α reductase ○ 3. DHT binds to Androgen Receptor (AR) ○ 4. AR dimerizes (2 hormone-bound receptor units come together) ○ 5. DHT-AR dimerized complex binds to DNA at the site of an Androgen-response element ○ 6. Transcription is turned on, making new mRNA ○ 7. Changes in transcription leads to powerful reprogramming of cells Androgen insensitivity syndrome ○ Due to inactivating mutation in Androgen receptor ○ 46 XY male who has high androgen levels ○ but the androgen receptor is not working due to mutation ○ female appearance (breast development, but short vaginas & no genital hair) ○ Undescended testes & high androgen levels Estrogen receptor action (canonical pathway) Mechanism of action of cortisol & aldosterone Apparent Mineralocorticoid Excess ○ 1. Rare genetic disorder in which a high mineralocorticoid state (hypertension, hypokalemia, suppressed plasma renin) is manifested. However, serum aldosterone levels are paradoxically low. ○ 2. The major abnormality in steroid hormone levels is a markedly elevated ratio of cortisol to cortisone. ○ 3. It was suggested that a deficiency of 11β-OHSD at mineralocorticoid target tissues precluded the inactivation of glucocorticoids at these sites leading to persistent hypermineralocorticism (glucocorticoids ○ circulate at very high levels and bind to the MR). That is high levels of glucocorticoid bring about mineralocorticoid-dependent effects in the absence of 11β-OHSD activity. ○ 4. An AME-like state was also observed in individuals following the ingestion of large amounts of licorice or from treatment with carbenoxalone, a drug used in peptic ulcer disease An explanation for this lies in the demonstration that glycyrrhetinic acid, the active ingredient in licorice and carbenoxalone both inhibited 11β-OHSD and lead to a condition like AME ○ 5. Cloning of the kidney form of 11β-OHSD has facilitated genetic studies demonstrating that individuals with AME have loss of function mutation in 11β-OHSD Steroid summary Breast cancer & estrogens ○ Lots of breast tumors contain the estrogen receptor & are stimulated by estrogens ○ Tumor growth stopped by intervention with: ○ Antiestrogens Block activation of estrogen receptor blocking its transcriptional activation of genes SERMs, selective estrogen receptor modulators ○ aromatase inhibitors Block synthesis of estrogens Altered pattern of steroid hormone synthesis in pregnancy Steroid summary ○ Synthesis: Dynamic process of enzymatic conversions-hydroxylation and clipping of steroid backbone Enzyme deficiencies may lead to disorders of sexual maturation (21-hydroxylase or 5α-Reductase) ○ 2. Regulation of steroid synthesis is organ-specific, based on available ○ Enzymes A steroid hormone can be made in one organ, & modified by a different organ to make the hormone more or less potent Ovaries can make androstenedione, estrone, and estradiol Adrenal glands make androstenedione & adipose tissue converts it to estrone ○ 3. Target organs regulate the effect of hormone by changing the amount steroid or intracellular receptor A hormone can not act without its receptor (Androgen Insensitivity Syndrome) Apparent Mineralocorticoid Excess SERMS can be used to treat Estrogen Receptor positive Breast Cancer Fetal HPA axis PG regulate uterine contraction CRH can directly mediate labor ○ CRH enhances prostaglandin (PG) production in decidua, amnion, chorion, & placenta ○ PG enhances placental CRH expression ○ PG’s bind to receptors in uterine fundus to trigger contractions, in the lower uterine segment to trigger relaxation, and in the cervix to trigger release of matrix metalloproteinases (MMP) that promote cervical dilation & shortening Cortisol can directly mediate labor ○ Cortisol also leads to prostaglandin prosecution by: Inducing clyclo-oxygenase-2 (COX-2) expression in amnion to make PG Inhibiting prostaglandin dehydrogenase (PGDH) expression in chorion to reduce PG breakdown Estrogen can indirectly mediate labor ○ CRH directly & indirectly (vita ACTH) stimulates of fetal adrenal cortisol & DHEAS production ONLY when fetal zone has developed (> 24-28 weeks) Dehydroepiandrosterone sulfate (DHEAS) is a hormone that plays a key role in reproduction in all sexes produced by the adrenal glands and is converted into estrogen and androgens like testosterone DHEA and DHEA-S may help with ovarian function, pregnancy rates, and miscarriage rates DHEA and DHEA-S may also help with in vitro fertilization (IVF) outcomes DHEA and DHEA-S may help with calcium oscillations during oocyte maturation High levels of DHEAS may indicate congenital adrenal hyperplasia, an adrenal tumor, or polycystic ovary syndrome (PCOS) Low levels of DHEAS may indicate Addison disease or hypopituitarism ○ DHEAS converted to estrone (E1), estradiol (E2), & estriol (E3) in placenta ○ Estrogen enhances expression of contraction associated proteins (CAPs): Connexin 43 (gap junctions), MLCK, COX-2, OT receptor, PG receptors (FP & EP1,3 in fundus; EP2,4 in LUS) Does functional progesterone withdrawal occur in human parturition (childbirth) ○ RT-PCR, immunohistochemistry & immunoblotting indicate that PGs decrease uterine fundal myometrial expression of Progesterone receptor (PR) mRNA & protein ○ Resulting decrease in expression of PR-responsive genes increases sensitivity of uterus to contractile stimuli Summary again Steroid Hormones– Key Points (cont’d) ○ 4. Parturition regulated by the coordinated action of CRH, cortisol, PGs, and estradiol ○ Increased cortisol leads to increased placental CRH levels ○ CRH and cortisol stimulate PG action by increasing PG receptors and decreasing PG breakdown ○ Estradiol promotes myometrial contraction by increasing CAPs, myometrial OT receptor, MLCK, PG receptors and COX-2 ○ Functional progesterone withdrawal in humans through decreased fundal PR levels? ESTROGENS AND ANDROGENS ○ Estrogens—drive female pubertal maturation, bone development ○ Androgens—virilization of males ○ Men & women have both estrogens & androgens Lecture 8 - Hypothalamus-Pituitary-Gonadal Axis (HPG Axis) Concepts in hormone action Hormone (ligand) - substance that exerts its effects at sites remote from its origin Receptor - allows receipt of hormone signal by the target cell & helps broadcast the signal Autocrine (influence self), paracrine (influence neighbors), endocrine (influence remote target) HPG Axis Hypothalamus makes GnRH GnRH stimulates pituitary gland to make LH & FSH LH stimulates testes to make testosterone FSH stimulates FSH to make estrogen & progesterone Here's a more detailed summary of the Hypothalamic-Pituitary-Gonadal (HPG) Axis: 1. Hypothalamus: Releases gonadotropin-releasing hormone (GnRH) in a pulsatile manner. 2. Anterior Pituitary Gland: GnRH stimulates the release of luteinizing hormone (LH) and follicle-stimulating hormone (FSH). 3. Gonads (Testes & Ovaries): ○ In males, LH stimulates Leydig cells in the testes to produce testosterone, which supports spermatogenesis and secondary sex characteristics. ○ In females, LH triggers ovulation and stimulates the corpus luteum to produce progesterone, while FSH promotes follicle growth and estrogen production from granulosa cells. 4. Negative Feedback: ○ In males, testosterone and inhibin (from Sertoli cells) inhibit GnRH, LH, and FSH secretion. ○ In females, estrogen and progesterone regulate the cycle through both negative and positive feedback depending on the phase of the menstrual cycle.The hypothalamic-pituitary-gonadal (HPG) axis is regulated by both negative and positive feedback mechanisms, which vary depending on the phase of the menstrual cycle. More about negative & positive feedback depending on menstrual cycle Negative Feedback (Most of the Cycle) Early Follicular Phase: ○ The hypothalamus releases GnRH, stimulating the pituitary gland to release FSH and LH. ○ FSH promotes follicle growth in the ovary, leading to the production of estrogen. ○ Rising estrogen levels inhibit GnRH, FSH, and LH secretion through negative feedback, preventing excessive hormone production. Luteal Phase: ○ After ovulation, the corpus luteum produces progesterone and estrogen. ○ High progesterone and moderate estrogen suppress GnRH, FSH, and LH secretion through negative feedback, preventing another ovulation. ○ If fertilization doesn’t occur, the corpus luteum degenerates, progesterone and estrogen levels drop, and the cycle restarts. Positive Feedback (Ovulation) Late Follicular Phase (Ovulatory Surge): ○ When estrogen levels rise sharply, they switch from negative to positive feedback. ○ High estrogen stimulates the hypothalamus and pituitary to release a surge of LH (and a smaller FSH surge). ○ This LH surge triggers ovulation, causing the dominant follicle to release an oocyte. Cycle Reset If pregnancy doesn’t occur, progesterone and estrogen levels drop, removing the negative feedback. This allows GnRH, FSH, and LH to rise again, starting a new cycle with follicular recruitment. This intricate feedback system ensures proper timing of ovulation, preparation of the uterus for implantation, and regulation of hormone levels. The hypothalamic-pituitary-gonadal (HPG) axis is regulated by both negative and positive feedback mechanisms, which vary depending on the phase of the menstrual cycle. Negative Feedback (Most of the Cycle) Early Follicular Phase: ○ The hypothalamus releases GnRH, stimulating the pituitary gland to release FSH and LH. ○ FSH promotes follicle growth in the ovary, leading to the production of estrogen. ○ Rising estrogen levels inhibit GnRH, FSH, and LH secretion through negative feedback, preventing excessive hormone production. Luteal Phase: ○ After ovulation, the corpus luteum produces progesterone and estrogen. ○ High progesterone and moderate estrogen suppress GnRH, FSH, and LH secretion through negative feedback, preventing another ovulation. ○ If fertilization doesn’t occur, the corpus luteum degenerates, progesterone and estrogen levels drop, and the cycle restarts. Positive Feedback (Ovulation) Late Follicular Phase (Ovulatory Surge): ○ When estrogen levels rise sharply, they switch from negative to positive feedback. ○ High estrogen stimulates the hypothalamus and pituitary to release a surge of LH (and a smaller FSH surge). ○ This LH surge triggers ovulation, causing the dominant follicle to release an oocyte. Cycle Reset If pregnancy doesn’t occur, progesterone and estrogen levels drop, removing the negative feedback. This allows GnRH, FSH, and LH to rise again, starting a new cycle with follicular recruitment. This intricate feedback system ensures proper timing of ovulation, preparation of the uterus for implantation, and regulation of hormone levels. Corpus luteum: The corpus luteum is a temporary endocrine structure that forms from the ruptured follicle after ovulation. It plays a crucial role in the luteal phase of the menstrual cycle by producing hormones necessary for maintaining a potential pregnancy. Formation of the Corpus Luteum: 1. Ovulation (Day 14 of a 28-day cycle) ○ A surge in LH triggers the release of the mature oocyte from the dominant follicle. ○ The remnants of the follicle collapse and reorganize into the corpus luteum. 2. Hormone Production (Luteal Phase, Days 15–28) ○ The corpus luteum secretes progesterone, estrogen, and inhibin. ○ Progesterone is the dominant hormone, preparing the uterus for implantation by thickening the endometrium and inhibiting further ovulation. ○ Negative feedback from progesterone suppresses GnRH, FSH, and LH release, preventing new follicle development. 3. If Fertilization Occurs: ○ The implanted embryo secretes hCG (human chorionic gonadotropin), which maintains the corpus luteum. ○ The corpus luteum continues producing progesterone until the placenta takes over hormone production around 10–12 weeks of pregnancy. 4. If Fertilization Does Not Occur: ○ The corpus luteum degenerates into the corpus albicans (a scar-like structure). ○ Progesterone and estrogen levels drop, leading to menstruation and the start of a new cycle. The corpus luteum is essential for early pregnancy support, and its failure to function properly can lead to luteal phase defects, which may cause infertility or early pregnancy loss. Hypothalamic signals Stimulatory (releasing factors) ○ Gonadotropin releasing factor (GnRH) ○ Thyrotropin releasing factor (TRH) ○ Growth hormone releasing hormone (GHRH) ○ Corticotropin releasing hormone (CRH) Inhibitory (blocking factors) ○ Prolactin inhibitory factor (dopamine) ○ Somatostatin Hypothalamic-Pituitary-Gonadal (HPG) Axis & Its Interactions with Other Hormonal Pathways The HPG axis regulates reproductive function through a cascade of hormone signals originating from the hypothalamus and acting on the pituitary and gonads. However, it does not operate in isolation—several other hypothalamic factors influence or interact with the HPG axis. Core HPG Axis Regulation 1. Gonadotropin-Releasing Hormone (GnRH) ○ Secreted by the hypothalamus in a pulsatile fashion. ○ Stimulates the anterior pituitary to release luteinizing hormone (LH) and follicle-stimulating hormone (FSH). ○ LH: Stimulates Leydig cells in males to produce testosterone and theca cells in females to produce androgens (which are converted into estrogen). ○ FSH: Stimulates Sertoli cells in males for spermatogenesis and granulosa cells in females for follicular development & estrogen production. ○ Regulated by negative & positive feedback: Negative feedback: Testosterone, estrogen, and inhibin suppress GnRH, LH, and FSH secretion. Positive feedback: High estrogen levels before ovulation trigger an LH surge for ovulation. Other Hypothalamic Hormones & Their Effects on the HPG Axis 2. Thyrotropin-Releasing Hormone (TRH) ○ Stimulates TSH (thyroid-stimulating hormone) and prolactin release from the pituitary. ○ Prolactin inhibits GnRH, meaning that excessive TRH (e.g., in hypothyroidism) can suppress reproductive function. 3. Growth Hormone-Releasing Hormone (GHRH) ○ Stimulates release of growth hormone (GH). ○ GH indirectly influences reproduction by supporting gonadal development, gametogenesis, and steroidogenesis. 4. Corticotropin-Releasing Hormone (CRH) ○ Stimulates ACTH (adrenocorticotropic hormone) release, which promotes cortisol production. ○ High cortisol suppresses GnRH, leading to hypogonadism in chronic stress or conditions like Cushing’s syndrome. Inhibitory (Blocking) Factors & Their Role in Reproduction 5. Prolactin Inhibitory Factor (Dopamine) ○ Inhibits prolactin secretion. ○ Prolactin negatively regulates the HPG axis by suppressing GnRH. ○ Hyperprolactinemia (e.g., from a pituitary tumor) can cause amenorrhea and infertility. 6. Somatostatin (Growth Hormone-Inhibiting Hormone) ○ Inhibits GH, TSH, and some gastrointestinal hormones. ○ While not a primary regulator of reproduction, GH suppression can indirectly affect gonadal function. Summary: Interplay of Hypothalamic Hormones on Reproduction HPG axis is tightly controlled by GnRH, with feedback from sex hormones. TRH and CRH can inhibit reproduction (via prolactin and cortisol, respectively). GHRH and GH support gonadal function, while somatostatin suppresses GH. Dopamine maintains HPG function by keeping prolactin in check. These complex hormonal interactions help balance reproduction, metabolism, growth, and stress responses in the body. Pulsatile GnRH secretion is essential Summary of anterior pituitary key performers The gonad: instrument Ovary ○ Follicle Oocyte (germ cell) surrounded by granulosa cells ○ Granulosa cells (follicular endocrine cells) ○ estrogen (in response to FSH) ○ progesterone (in response to LH and hCG) ○ inhibin (A and B) and anti-mullerian hormone (AMH) Thecal cells (stromal endocrine cells) ○ Androgens in response to LH Ovarian steroidogegenic pathway 2 cell 2 gonadotropin theory ○ Follicular phase ○ Luteal phase/pregnancy Ovarian steroid hormones regulate endometrial development HPO function dictates menstrual cycle ○ hypothalamic-pituitary-ovarian (HPO) Testes Spermatocyte ○ Germ cell of testis Sertoli cells ○ Pituitary FSH binds Sertoli cells to control sperm production Leydig cells (stromal) ○ Make testosterone in response to pituitary LH to support spermatogenesis and full reproductive function Synchrony between the HPG axis players is critical to normal reproductive physiology Case 1 - 28 year old male with: ○ Kallman syndrome (absence of GnRH neurons) ○ azoospermia (absent sperm in ejaculate) ○ poor masculinization ○ anosmia (impaired sense of smell) Case 2 - 2 year old infertile woman ○ functional hypothalamic amenorrhea (disturbance in hypothalamic GnRH secretion) ○ 2 years of infertility; inquiring about options to conceive ○ Pulsatile GnRH pump or FSH/LH replacement Case 3 28 year old female with a history of ○ hemorrhage after delivery ○ inability to establish breast milk flow ?