Material Science Notes (4) PDF
Document Details
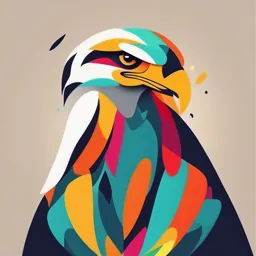
Uploaded by ResoluteLotus1932
University of Stellenbosch
Lucy Van Der Meulen
Tags
Summary
These notes detail material classifications such as metals, ceramics, polymers, and composites. Different types of atomic bonding are also explained, including, ionic, covalent, and metallic bonds. The structure of crystalline solids, including metallic crystal structures like FCC and BCC, is discussed, along with other topics such as coordination number and atomic packing factor.
Full Transcript
MATERIAL SCIENCE 244 NOTES LUCY VAN DER MEULEN CHAPTER 2: Classification of materials: Material Characteristics: Metals Composed of one or more metallic elements...
MATERIAL SCIENCE 244 NOTES LUCY VAN DER MEULEN CHAPTER 2: Classification of materials: Material Characteristics: Metals Composed of one or more metallic elements Might contain non-metallic elements in small amounts Atoms arranged in orderly manner Contain delocalised electrons which are not bound to particular atoms Characteristics: stiff, strong, ductile, fracture-resistant Ceramics Metallic and nonmetallic element compounds Characteristics: stiff, strong, hard, insulative, (used to be: brittle, fracture easily), some are metallic and can be either transparent, translucent or opaque Polymers Large molecular structures, often chainlike made of carbon, hydrogen and other nonmetallic elements Characteristics: low density, not stiff, not strong, ductile, pliable, chemically inert, unreactive Disadvantages: tend to soften and decompose at modest temperatures, low electrical conductivity, nonmagnetic Composites Composite of two or more individual materials from metals, ceramics and polymers Aim to achieve a combination of properties not displayed by a single material Advanced materials Semiconductors Electrical properties that are intermediate between electrical conductors and insulators Extremely sensitive electrical characteristics to impurity atoms Biomaterials Used in human bodies to replace damaged body parts Do not produce toxic substances and are compatible with body tissues Smart materials Able to sense changes in their environment Nanomaterials Very small materials of any classification Atomic structure: Atomic number (Z) - number of protons in the nucleus Atomic mass (A) - sum of masses of protons and neutrons in the nucleus Isotopes - different amounts of neutrons cause different atomic masses Atomic weight (A bar) - weighted average of all atomic masses (measured in amu) 1 Quantum numbers: Energy levels are characterised by quantum numbers Valence electrons occupy the outermost shell of the electron Atomic bonding Net force - Fa + Fr = Fn Net force = 0, Fa = Fr (attraction = repulsion) Net potential energy - Ea + Er = En Bonding energy (Eo) - energy required to move atoms infinitely apart Ionic bonding - Metallic and nonmetallic elements - Atoms of metallic elements give up valence electrons easily to nonmetallic elements - All atoms acquire stable configurations - Coulombic bonding forces (positive and negative atoms are attracted to each other) Covalent bonding - Atoms with small differences in electronegativity - Each atom donates one or more electron to the bond which is shared Metallic bonding - A sea of free valence electrons surround a positive kernel - Electrons are free to move, thus metals are good conductors of heat and electricity and are ductile 2 Atoms, molecules, compounds and ions - Atoms - single neutral particles - Molecules - neutral particles made of two or more atoms bonded together - Compounds - special types of molecules where two or more different atoms are bonded together - Ion - a positively or negatively charged particle Intermolecular bonding - Van der Waal’s forces - weak bonding forces that occur between atoms within molecules when coulombic attraction occurs between positive and negative ends of adjacent dipoles - Dipoles - a separation of positive and negative portions of an atom or molecule Types of intermolecular forces - London dispersion forces: weakest intermolecular forces in which there is temporary attraction between dipoles that occur when electrons in two adjacent non-polar molecules and atoms occupy positions that induce temporary, momentary dipoles - Dipole-Dipole: permanent dipole moments occur in molecules due to asymmetrical arrangement of positively and negatively charged regions (polar molecules). This attraction results in permanent attraction between atoms and molecules which are stronger than London dispersion forces. - Hydrogen Bonding - coulombic forces exist between adjacent polar molecules. Hydrogen bond forces occur between molecules in which hydrogen is covalently bonded to fluorine, oxygen or nitrogen (HF, H2O, NH3) in which the single hydrogen electron is shared with the other atom, thus the highly positive charged end of the molecule strongly attracts the negative end of its adjacent molecule. This is the strongest intermolecular force 3 Mixed bonding - Many real materials contain bonding of two or more types. Thus they fall in the spectrum between different bonding types and contain properties from both - Percentage ionic character can be evaluated as follows: - 2 %𝐼𝐶 = {1 − 𝑒𝑥𝑝[− (0. 25)(𝑋𝑎 − 𝑋𝑏) ]} × 100 CHAPTER 3: CRYSTAL STRUCTURES Crystalline material - atoms are situated in a repeating or periodic array over large atomic distances Crystal structure - the manner in which atoms, ions or molecules are spatially arranged Lattice - three-dimensional array of points coinciding with atom positions Unit cells - the basic structural unit of a crystal structure, generally defined in terms of atom or ion positions within parallelepiped volume Crystal systems - groups of crystal structures according to unit cell configurations and/or atomic arrangements Lattice parameters - the combination of unit cell edge lengths and interaxial angles that defines the unit cell geometry 4 Point coordinate indices qa - lattice position referenced to the x-axis rb - lattice position referenced to the y-axis sc - lattice position referenced to the z-axis Crystallographic direction - a line directed between two points (a vector) Method of determining crystallographic directions: 1. A right handed x-y-z coordinate system is constructed with origin located at unit cell corner 2. The coordinates of two points that lie on the direction vector are determined 3. Tail point coordinates are subtracted from head point components (x2-x1, y2 - y1, z2 - z1) 4. The coordinate differences are normalised (x2-x1/a, y2 - y1/b, z2 - z1/c) 5. Answers are multiplied/divided by a common factor to reduce to smallest integer values 6. Resulting indices are enclosed in square brackets (not separated by commas) ) (negative values are shown with a line on top of number) 𝑥2,𝑦2,𝑧2 − 𝑥1,𝑦1,𝑧1 𝑢, 𝑣, 𝑛 = 𝑛( 𝑎,𝑏,𝑐 5 Hexagonal crystals - Four-axis system is used - Formulas: 𝑢 = 1 3 (2𝑈 − 𝑉) - 1 𝑣 = 3 (2𝑉 − 𝑢) - 𝑡 = − (𝑢 + 𝑣) - 𝑤 = 𝑊 Crystallographic planes 1. If the plane passes through the selected origin, another parallel plane must be constructed or a new origin must be established at the corner of another unit cell 2. The crystallographic plane either intersects or parallels each of the three axis, the coordinate of the intersection with the crystallographic plane with each axis is determined (A,B,C) 3. The reciprocals of these numbers are taken 4. Normalise (a/A, b/B, c/C) 5. Multiply by common factor to get lowest integer value 6. Integer indices enclosed within round brackets (no commas) 7. ,𝑘 = ,𝑙 = 𝑛𝑎 𝑛𝑏 𝑛𝑐 ℎ = 𝐴 𝐵 𝐶 Hexagonal Crystals - 𝑖 = − (ℎ + 𝑘) 6 CRYSTALLINE VS NON CRYSTALLINE MATERIALS Single crystals - Single crystals: periodic or repeated arrangement of atoms is perfect or extends throughout the entire specimen without interruption - Unit cells interlock in the same way and have the same orientation - Regular geometric shape with flat surfaces Polycrystalline materials - Polycrystalline materials: crystalline solids composed of a collection of small crystals (grains) - Crystallographic orientation varies from grain to grain - Grain boundary - atomic mismatch within the region where two grains meet - Formation: small nuclei with random crystallographic orientations form at various positions. Grains grow by the successive addition from the surrounding liquid of atoms to the structure. Extremities impinge on each other as the solidification process approaches completion. Anisotropy - the physical properties of single crystals of some substances depend on the crystallographic direction in which measurements are taken (eg. elastic modulus, electrical conductivity, index of refraction). This is associated with the variance of atomic or ionic spacing with crystallographic direction. Varying properties in different directions. - The degree of anisotropy increases with decreasing structural symmetry Isotropic - substances in which measures properties are independent of the direction of measurement Polycrystalline materials directional properties are averaged over the whole specimen. Noncrystalline solids - solids that lack a systematic and regular arrangement of atoms over relatively large atomic distances (called amorphous) 7 CHAPTER 4: THE STRUCTURE OF CRYSTALLINE SOLIDS METALLIC CRYSTAL STRUCTURES - Contains non directional metallic bonding - Minimal restrictions to number and position of nearest-neighbour atoms - Large numbers of of nearest neighbours and dense atomic packings FACE-CENTRED CUBIC CRYSTAL STRUCTURE - Atoms located at each on the corner and the centres of all the cube faces Relationship between cube length (a) and atomic radius R Atoms in a unit cell Coordination number - number of touching atoms Atomic packing number (ATP) - the sum of sphere volumes of all atoms within a unit cell divided by the unit cell volume - Coordination number - 12 - APF of 0.74 - Metals have a large atomic packing factors to maximise the shielding provided by the free electron cloud BODY-CENTRED CUBIC CRYSTAL STRUCTURE - Atoms located at all eight corners and a single atom at the cubic centre Relationship between cube length (a) and atomic radius R - Coordination number - 8 - APF of 0.68 - Atoms situated only on corners: simple cubic crystal structure. No metals, only polonium HEXAGONAL CLOSE-PACKED CRYSTAL STRUCTURE Atoms in a unit cell 𝑐 𝑎 = 1. 633 - Coordination number - 12 - ATP of 0.74 8 DENSITY COMPUTATIONS-METALS CERAMIC CRYSTAL STRUCTURES Ionic arrangement geometries: - Cations and anions bonded together - Crystal structure influenced by magnitude of electrical charge and relative sizes of ions Name Coordination number Cation-Anion Radius Coordination geometry Ratio (rc/ra) Linear 2 diamond) - High thermal conductivity - Uses: lubricants, pencils, brake shoes, heating elements Carbon fibres - Small-diameter, high-strength, high-modulus fibres composed of carbon are used as reinforcements in polymer-matrix composites - Consists of graphene layers: Graphitic fibres take on the structural arrangement of graphite, Turbostratic fibres take on a more disordered structure due to randomly folded, tilted and crumpled sheets - Highly anisotropic - stronger and stiffer along fibre than perpendicular to it - Expensive Advanced ceramics (still being developed and advanced) - Nanocarbons < 100nm - Fullerenes: 60 carbon atoms bonded into spherical hollow clusters which can attach or encapsulate other atoms. Used in antioxidants, catalysts, biopharmaceuticals - Carbon nanotubes: single sheet of graphite rolled into a tube which are extremely strong, stiff, relatively ductile and low density. Used: structural applications, reinforcements - Graphene: single-atomic-layer composed of hexagonally bonded carbon atoms. Perfect order, unbonded electrons move faster than conducting electrons in ordinary metals and semimetals 19 CHAPTER 6 Imperfections in solids Crystalline defect - a lattice irregularity having one or more of its dimensions on the order of an atomic diameter Point defects in metals: - Defects associated with one or two atomic positions - Vacancy: one normally occupied but from which an atom is missing. It is not possible to create a material that is free of these defects - The presence of vacancies increases the entropy of the crystal Equilibrium number of vacancies - N: total number of atomic sites - Qv: the energy required for the formation of a vacancy - T:the absolute temperature in kelvin - K: Boltzmann constant (1.38X10-23 J/atom.K or 8.62 X10-5 eV/atom.K) The total number of vacancies increases exponentially with temperature Self interstitial: an atom from the crystal that is crowded into an interstitial site interstitial site - a small void space that under ordinary circumstances is not occupied Point defects in ceramics: - Defect structure: designates the types and concentrations of atomic defects in ceramics - Electroneutrality: the state that exists when there are equal numbers of positive and negative charges from ions. Therefore defects in ceramics occur in pairs to maintain this electroneutrality - Frenkel defect: defect involving cation-vacancy and cation-interstitial pair - Schotty defect - cation vacancy-anion vacancy pair - Stoichiometry: a state for ionic compounds wherein there is the exact ratio of cations to anions as predicted by the chemical formula. - Nonstoichiometry may occur for some ceramic materials in which two valence states exist for one of the ion types Impurities in metals: Alloy: impurity atoms have been added intentionally to impart specific characteristics to the material Solvent: the element or compound that is present in the greatest amount Solute: an element or compound present in a minor concentration Solid solutions - Forms when solute atoms are added to a host material, but the crystal structure is maintained and no new structures are formed - Solid solutions are compositionally homogeneous, the impurity atoms are randomly and uniformly dispersed within the solid 20 Metal impurity point defects: Substitutional - solute or impurity atoms replace or substitute the host atoms Interstitial - an atom is added in the interstitial space Hume-Rothery rules: 1. Atomic size factor: difference in atomic radii is less than +-15% 2. Crystal structure: must be the same 3. Electronegativity factor: the greater the electronegativity difference, the more likely a intermetallic compound will form instead of a substitutional solid solution 4. Valences: a metal with high valency will dissolve easier than a metal with low valency Impurities in ceramics - Impurity atoms can form solid solutions in ceramic materials - Solid solutions of both substitutional and interstitial types are possible - For an interstitial, the ionic radius of the impurity must be relatively small in comparison to the anion - Ionic size and charge must be very nearly the same, otherwise crystal defects are formed to compensate Point defects in polymers - Point defects are found in crystalline regions of polymeric materials, these include vacancies and interstitial atoms and ions - Chain ends are considered defects as they are chemically dissimilar to normal chain units, vacancies are also associated with chain ends - Additional defects can result from branches in the polymer chain - A chain selection can leave a polymer crystal and re-enter it at another point, creating a loop, or can enter a second crystal to act as a tie molecule - Screw dislocations occur in polymer crystals - Impurity atoms/ions or groups of atoms/ions may be incorporated in the molecular structure as interstitials - The surfaces of chain-folded layers are considered interfacial defects and so are boundaries between two adjacent crystalline regions Specification of composition - Composition: constituent elements within a substance Mass percentage: Atom percentage: Mass to atom %: Atom to mass%: 21 Determine the density and atomic mass of binary alloy, given the composition of either mass percent or atom percent —> MISCELLANEOUS IMPERFECTIONS MICROSCOPY: 22 CHAPTER 7: DIFFUSION 23 Chapter 8 - Mechanical Properties of Metals Tensile testing: - “Dogbone” configuration - circular cross section with rectangular ends confines deformation the narrow central region - Standard dimensions: diameter = 12.8mm, reduced section length = 60mm, gauge length = 50mm Engineering Stress-Strain: 24 - Proportional limit - initial departure from linearity of the stress-strain curve - Yield point (Y) - the point at which material begins to yield, typically defined as the stress at which a strain offset of 0.2% (0.002) from the straight line has occurred. Yield point is called yield strength, yield stress or elastic limit. After the yield point is reached, hooke’s law is no longer applicable - The upper yield point, plastic deformation is initiated with an apparent decrease in engineering stress - The lower yield point is the continued fluctuation about the constant stress and the stress subsequently rises with equal strain - Yield strength (N) - a material’s resistance to plastic deformation - Tensile Strength (TS) - the engineering stress at the point at which the applied load reaches a maximum value (MPa) 𝐹𝑚𝑎𝑥 𝑇𝑆 = 𝐴𝑜 - Necking - localised elongation in which the diameter of a specimen is reduced - Fracture stress - the stress calculated immediately before failure - Ductility - the amount of strain that a material can endure before failure (elongation or area reduction) (mm) (mm2) 𝐿𝑓−𝐿𝑜 𝐴𝑜−𝐴𝑓 𝐸𝑙𝑜𝑛𝑔𝑎𝑡𝑖𝑜𝑛 = 𝐿𝑜 𝐴𝑟𝑒𝑎 𝑅𝑒𝑑𝑢𝑐𝑡𝑖𝑜𝑛 = 𝐴𝑜 Modulus of elasticity (Hooke’s law, see before) Anelasticity - time-dependent elastic strain component in which elastic deformation continues after stress application for some finite time Plastic deformation - a material is deformed beyond the yield point and so the stress is no longer proportional to the strain and permanent, nonrecoverable deformation occurs - Corresponds to the breaking of bonds with original atom neighbours and forming bonds with with new neighbours - Crystalline solids - deformation is accomplished by means of slip, which involves the motion of dislocations. - Non-crystalline solids - deformation occurs by viscous flow 25 Resilience: - The capacity of a material to absorb energy when it is deformed elastically and then to recover this energy after unloading Toughness: - A property that indicates a material's resistance to fracture when a crack is present or a material’s ability to absorb energy and plastically deform before fracturing (area under entire curve until fracture point, use mod of resilience formula) True Stress-Strain Strain hardening - a metal becomes stronger as strain increases 𝑛 σ = 𝐾𝑒 26 Hardness Tests: - Hardness: a measure of a material’s resistance to localised plastic deformation - Used for assessing material properties as they are quick, convenient, simple, inexpensive, nondestructive and other mechanical properties can be estimated from hardness data 27 Chapter 9 - Dislocations and strengthening mechanisms Dislocations and plastic deformation Edge dislocation - localised lattice distortion exists along the end of an extra half-plane of atoms Screw dislocation - results from shear distortion in which its dislocation line passes through the centre of a spiral Mixed dislocations - dislocations in crystalline materials that have both edge and screw dislocations Plastic deformation - the motion of large numbers of dislocations Slip - the process by which plastic deformation is produced by dislocation motion Slip line - the crystallographic plane along which the dislocation line transverses - When a shear stress is applied to a material, a plane shifts one unit, which in turn shifts the neighbouring atoms. If the applied force is sufficient in breaking the interatomic bonds between atoms, an extra half plane forms parallel to the structure Dislocation density - total dislocation length per unit volume Characteristics of dislocations: Lattice strains - regions in which compressive, tensile and shear strains are induced due to the the presence of the extra half-plane of atoms Slip systems: - Dislocations do not move with the same degree of ease on all crystallographic planes in all crystallographic directions - Slip plane and slip directions - a preferred plane and specific directions along which dislocation motion occurs - Slip system - combination of slip plane and slip direction (the slip plane is the plane that has the densest atomic packing in crystal structures, therefore different for each material) 28 Slip in single crystals: Plastic deformation of polycrystalline materials: - Slip varies from one grain to another due to the random crystallographic orientations of the numerous grains - Polycrystalline materials are stronger than their single-crystal equivalents - Individual grains are constrained by neighbouring grains such that they can only slip if their neighbours are also in the correct orientation Mechanisms of strengthening in metals: - Ability of a metal to deform plastically depends on the ability of dislocations to move - Reducing the mobility of dislocations enhances the mechanical strength of the metal Strengthening by grain size reduction - Grain boundaries act as barriers to dislocation motion - 1. A dislocation must change its direction of motion in order to pass through a grain of different orientation. This becomes more difficult as crystallographic misorientation increases - 2. Atomic disorder within a grain boundary region results in a discontinuity of slip planes form one grain to another - A fine-grained material is harder, stronger and tougher than a coarse grained material as it has a greater total grain boundary area which slows dislocation - Hall-petch equation: d - average grain diameter Oo and Ky- constants Solid-solution strengthening: - Involves alloying a metal with impurity atoms that go into either substitutional or interstitial solid solution - High purity metals are almost always softer and weaker than alloys - Increasing the concentration of impurity, increases tensile and yield strengths as dislocation movement is restricted 29 Strain-hardening: - The phenomenon by which a ductile metal metal becomes harder and stronger as it is plastically deformed - Percent cold work: Ao - original area of cross section that experiences deformation Ad - the area after deformation - Results in closer positioned dislocations which hinder the presence of other dislocations Recovery, recrystallization and grain growth: Recovery - some of the stored internal strain energy is relieved by virtue of dislocation motion resulting in a reduction in the number of dislocations and dislocation configurations are produced having low strain energies. This occurs as a result of heat, which enhances atomic diffusion. - Electrical and thermal conductivities revert back to pre cold-worked state Recrystallization - the formation of a new set of strain-free and equiaxed grains (equal dimensions in all directions) that have low dislocation densities and are characteristic to pre cold-worked condition - Driven by the difference in internal energy between strained and unstrained material - The new grains form as small nuclei which grow until they completely consume the parent material - Extent of recrystallisation depends on time and temperature - Recrystallisation temperature - the temperature at which recrystallisation just reaches completion in one hour - Increasing the percent cold work enhances the rate of recrystallisation - Recrystallization proceeds more rapidly in pure metals than in alloys as impunity atoms preferentially segregate at and intersect with recrystallized grain boundaries so as to diminish their mobilities, thus resulting in decreased recrystallization - Metals - recrystallization temperature = 0.4Tm (™ is the absolute melting temperature) - Alloys = 0.7Tm Grain growth - after recrystallisation, the strain-free grains will continue to grow if the metal is left at the elevated temperature - Does not need to be preceded by recovery and recrystallisation - As grains increase in size, the total boundary area decreases thus reducing the total energy - Grain growth occurs by the migration of grain boundaries - Average grain growth increases with time and at any particular time, a range of grain sizes exists (as large grains grow at the expense of small grain shrinkage) - Variation of grain diameter with time - do - initial grain diameter at t=0 - K and n are time dependent constants - t - time - Grain growth occurs more rapidly as temperature increases 30 Chapter 11 - Phase Diagrams Component - pure metals and/or compounds of which an alloy is composed Solute - a component or element of a solution present in minor concentration which is dissolved in the solvent. Solvent - component of a solution present in the greatest amount in which the solute is dissolved System -a specific body of material under consideration or the series of possible alloys consisting of the same components but without regard to alloy composition Solubility Limit - the maximum concentration of solute atoms that may dissolve in the solvent to form a solid solution at some specific temperature Solid solution - homogeneous crystalline phase that contains two or more chemical species, namely substitutional and interstitial solutions. If solute atoms are added in addition, the excess results in the formation of another distinct solid solution or compound with a different chemical composition. The point where the temperature and solubility line meet is the solubility limit at a specific temperature Phase - a homogeneous portion of a system that has uniform physical and chemical characteristics Phase diagram - graphical representation of the relationships among environmental constraints, compositions and regions of phase stability. Homogenous - a single-phase system Heterogeneous - systems composed of two or more phases (can also be called a mixture) Microstructure: - Determines physical properties and/or mechanical behaviour - Observed using optical or electron microscopes - Preparation - grind, polish, etch - Microstructure of a metal - characterised by: number of phases present, their proportions and the manner in which they are distributed and arranged - Microstructure of an alloy - characterised by alloying elements present, their concentrations and the heat treatment of the alloy Phase equilibria: - Equilibrium - a system is in equilibrium if its free energy is at a minimum under some specified combination of temperature, pressure and composition. This means that the characteristics of a system are stable with time unless there is a change in energy within the system (temperature, pressure or composition) - Free energy - a function of the internal energy of a system and also the randomness or disorder of the atoms or molecules present (entropy) - Phase equilibrium - equilibrium as it applies to systems in which more than one phase may exist, reflected by a constancy of phase characteristics of a system with time - Metastable state - when the rate of approach to equilibrium is extremely slow One-component (unary) phase diagrams: - Composition is held constant and pressure and temperature are varied - Represented as a 2D plot of pressure (y-axis) vs temperature (x-axis), in which the pressure axis is scaled logarithmically 31 Eg: H2O phase diagram: - Three different phases are shown which exist under equilibrium conditions - ao, bo and co are phase boundaries at which the two phases on either side of the respective curve can coexist with one another - At point o, the three phases coexist (triple point) Binary phase diagrams: - Pressure is held constant (usually 1 atm) and temperature and composition are varied - Represent the relationships between temperature and the compositions and quantities of phases at equilibrium which influence the microstructure of an alloy Binary isomorphous systems: - Isomorphous - have the same structure (same crystal structure/complete solid solubility for all compositions) - Binary - two components - Helpful in predicting phase transformations and the resulting microstructures, which may have equilibrium or nonequilibrium character Eg: Copper-nickel system - Temperature is plotted (y-axis) vs composition of the alloy (x-axis) in weight percent and atom percent of nickel - Three different phase regions (fields) are represented by alpha - solid, L - liquid, and a two-phase alpha + L - Liquidus line - line separating L and alpha + L phase fields - Solidus line - line between alpha + L and alpha phases - Liquidus and solidus lines indicate the melting temperatures for copper-nickel diagrams, any other compositions the melting temperature is a range Interpretation of phase diagrams: - Information available: phases present, compositions of present phases and percentage/fractions of the phases - Phases present: locate temperature-composition point on the diagram and note the phases present - Compositions of phases: 1. Follow the same procedure as in phases present 2. If only one phase is present, the composition of the phase is equal to the overall composition of the alloy 3. If two phases are present, first construct a tie line across the two-phase region at the temperature of the alloy (horizontal line). The intersection of the tie line and the phase boundaries on either side must be noted. Perpendicular lines are drawn from these intersections from which the composition of each of the respective phases is read 32 - Phase amounts: 1. If only one phase is present, the alloy is composed entirely of that phase and thus has a phase fraction of 1 or percentage of 100% 2. If two phases are present, a tie line must be used in conjunction with the level rule Development of microstructure in isomorphous alloys: Cooling occurs very slowly, thus equilibrium is continuously maintained - Example: copper-nickel system, cooling corresponds to moving down the vertical dashed line - a - alloy is completely liquid (35 wt% Ni, 65 wt% Cu) - b - the first solid alpha forms (Solid: 46 % Ni (tie line) and 54% wt% Cu, liquid composition stays the same - c - calculated in similar manner - d - solidification process complete (solid composition – 35% Ni, with very little liquid left over) - e - once crossing the solidus line, all the liquid solidifies Mechanical properties of isomorphous alloys: - Temperatures and compositions below melting temperature: single solid phase exists, thus each component experiences solid-solution strengthening (increase in strength and hardness due to the addition of other alloying components) Binary eutectic systems (copper-silver phase diagram): - Three single phase regions are represented: alpha, beta, L - Alpha - solid solution rich in copper with silver as a solute (also pure copper) and an FCC crystal structure, Beta -solid solution rich in silver with FCC structure and copper as a solute (also pure silver), L - liquid phase - Solubility of each phase is limited - Solvus line - solubility line separating ∝ and ∝ + β fields - Solidus line - solubility line separating ∝ and ∝ + L fields 33 - Eutectic reaction - reaction in which, upon cooling, a liquid phase transforms isothermally and reversibly into two mixed solid phases (at E) - 𝐿⇔𝛼+𝛽 Development of microstructure in eutectic alloys: - When crossing the liquidus and solidus lines, the same process occurs as with isomorphous alloys (d-f) - When the solvus line is crossed and 𝛼 solid solubility is exceeded, small 𝛽-phase particles form which will grow as cooling proceeds (shown at g) - When crossing the eutectic point, the liquid transform into alternating solid lamellae (1.) (at E on the diagram on the previous page) - For cooling taking place across the eutectic isotherm, (between 𝛼 + L or 𝛽 + L and 𝛼 + 𝛽) both eutectic and primary 𝛼-phases are Present. Below this line, the liquid phase transforms into a eutectic structure and the alpha particles that have already formed stay the same (2.) 1. 2. - Microconstituents - an element of the microstructure having an identifiable and charismatic structure Determining relative amounts of primary and eutectic phases: - Fraction of primary liquid: 𝑃 𝑊𝑒 = 𝑊𝑙 = 𝑃+𝑄 - Fraction of primary 𝛼: 𝑄 𝑊𝛼' = 𝑃+𝑄 - Fraction of total 𝛼: 𝑄+𝑅 𝑊𝛼 = 𝑃 + 𝑄+𝑅 - Fraction of total 𝛽: 𝑃 𝑊𝛽 = 𝑃+𝑄+𝑅 Equilibrium diagrams having intermediate phases or compounds: Terminal solid solutions - exist over composition ranges near the concentration extremes of a phase diagram Intermediate solid solution - have a compositional range which does not extend to either of the pure components of a system Intermetallic compounds - compounds of two metals with distinct chemical formulae that form an intermediate phase over a very narrow range of compositions 34 Eutectoid and peritectic reactions: Eutectoid reaction - reaction in which, upon cooling, one solid phase transforms isothermally and reversibly into two new solid phases 𝑠𝑜𝑙𝑖𝑑 𝑝ℎ𝑎𝑠𝑒 ⇔ 𝑠𝑜𝑙𝑖𝑑 𝑝ℎ𝑎𝑠𝑒 𝑛𝑒𝑤 1 + 𝑠𝑜𝑙𝑖𝑑 𝑝ℎ𝑎𝑠𝑒 𝑛𝑒𝑤 2 Peritectic reaction - an invariant reaction involving three phases at equilibrium. It is a reaction in which, upon cooling, a solid and a liquid phase transform isothermally and reversibly into a solid phase of different composition 𝑠𝑜𝑙𝑖𝑑 𝑝ℎ𝑎𝑠𝑒 + 𝑙𝑖𝑞𝑢𝑖𝑑 𝑝ℎ𝑎𝑠𝑒 = 𝑠𝑜𝑙𝑖𝑑 𝑝ℎ𝑎𝑠𝑒 𝑛𝑒𝑤 Congruent phase transformations: - Congruent Phase transformations - phase transformations in which there is no change in composition for the phases involved Eg. melting of pure metals and allotropic transformations - Incongruent Phase transformations - phase transformations in which at least one of the phases experience a change in composition Eg. eutectoid and eutectic reactions, melting of an alloy that belongs to an isomorphous system The iron-iron carbide phase diagram (NB) 35 - Ferrite - ∝-iron, the stable form of pure iron at room temperature which has a BCC crystal structure - Austenite - γ-iron, ferrite experiences a polymorphic transformation to FCC structure at 912 ℃ - At 1394 ℃, the austenite reverts back to BCC crystal structure. Called δ-phase which is only stable at relatively high temperatures - The δ-phase melts at 1538 ℃ - The composition axis only extends to 6.7%, at which cementite (Fe3C) is formed, this diagram is considered instead of the full weight percentage diagram due to the fact that all steels and cast irons contain less than 6.7% carbon in practice - Carbon is an interstitial impurity in iron. - In the BCC-∝-ferrite, only small concentrations of carbon are soluble. The max solubility is 0.022% wt% at 727 ℃ (which is explained by the BCC interstitial positions which make it difficult to accommodate the carbon atoms) - Effect of carbon on structure: BCC-∝-ferrite is soft, may be made magnetic at temperatures below 768℃ and has a density of 7.88 g/cm3 - Austenite (γ-iron), is not stable below 727℃ when alloyed with carbon alone. Its max solubility of carbon in austenite is 2.14 wt% at 1147℃ due to the FCC octahedral sites which are larger than BCC tetrahedral sites, which impose less strains on surrounding iron atoms - Austenite is non-magnetic - Cementite forms when the solubility limit in ∝-ferrite is exceeded below 727℃ which allow for ∝ + Fe3C compositions - Fe3C + γ also exist between 727℃ and 1147℃ - Cementite is very hard and brittle, thus increasing the strengths of steels, but it is metastable - Metastable - remains a compound indefinitely at room temperature - Eutectic - 4.30 wt% C and 1147℃ (𝐿 ⇔ γ + 𝐹𝑒3𝐶) - Eutectoid invariant point - 0.76 wt% C and 727℃ (γ ⇔ α + 𝐹𝑒3𝐶) - Commercial iron - contains less than 0.008 wt% C, 0.008 wt% - 2,14 wt% C are classified as steels (have α 𝑎𝑛𝑑 𝐹𝑒3𝐶 phases), but usually only contain less than 1.0 wt% C. Cast irons contain between 2,14 and 6.70 wt% C, but commercially less than 4.5 wt% C Development of microstructure in iron carbon alloys At eutectoid composition: - Austenite changes into ferrite and cementite packed in a pearlite structure - Pearlite - 2-phase microstructure consisting of alternating layers (lamellae) of 𝛼-ferrite and cementite - Carbon is redistributed by diffusion Hypoeutectoid alloys: - Solute concentration is less than the eutectoid composition - Contains between 0.022 and 0.76 wt% C - Proeutectoid ferrite - primary ferrite that exists in addition to pearlite for hypoeutectoid steels 36 Hypereutectoid alloys: - Solute concentration is greater than the eutectoid composition - Contains between 0.76 and 2.14 wt% C - Proeutectoid cementite - primary cementite that exists in addition to pearlite for hypereutectoid steels Non-equilibrium cooling: - Iron-carbon alloys have been given sufficient time to adjust phase compositions - This is impractical as cooling rates are slow and unnecessary - Nonequilibrium effects: - The occurrence of phase changes or transformations at temperatures other than those predicted by phase boundary lines on the phase diagram - The existence at room temperature of nonequilibrium phases that do not appear on the phase diagram Influence of other alloying elements: - Alloying elements (Cr, Ni, Ti etc) bring about significant changes in the iron-iron-carbide phase diagram. The extent of which depend on each individual alloying element - Causes a shift in position of the eutectoid with respect to temperature and eutectoid composition. Thus alloying elements alter temperature of eutectoid reactions as well as relative fractions of pearlite and proeutectoid phases that form - Steels are alloys to improve corrosion resistance or to render them amenable to heat treatment Limitation of phase diagrams: inability to indicate the time period required for the attainment of equilibrium 37 CHAPTER 12 - Phase transformations Phase transformation - involve some alteration of the microstructure in which a change in the number and/or characteristics of phases of an alloy occurs Transformation rate - dependance of reaction progress on time Simple diffusion dependent transformations - no change in number or composition of phases present eg. solidification, recrystallization or grain growth Diffusion dependent transformations - some alteration in the number or composition of phases present eg. eutectoid reaction Diffusionless transformations - a metastable phase is produced eg. martensite The kinetics of phase transformations: - Normally, at least one new phase is formed which has different physical/chemical characteristics and/or different structure than the parent phase - Phase transformations do not occur instantaneously - Nucleation - the initial stage of phase transformations evident by the formation of small particles of the new phase that are capable of growth - Growth - the increase in size of a particle of a new phase Kinetic considerations of solid-state transformations: - Kinetics - the time dependance of rate. The fraction of reaction that has occurred is measured as a function of time while the temperature is maintained constant. - Data is plotted as the function of transformed material versus the logarithm of time Metastable versus equilibrium states: - Phase transformation are induced by temperature changes and equilibrium conditions are not held - Transformations are shifted to lower/higher temperatures - Superheating - other-than-equilibrium heating, transformation are shifted to higher temperatures - Supercooling - for other-than-equilibrium cooling, transformations are shifted to lower temperatures - Degree of superheating/supercooling depends on the rate of temperature change. The more rapid, the greater the degree. 38 Microstructural and property changes in iron-carbon alloys: Isothermal transformation diagrams - plot temperature vs logarithm of time for steel of definite composition Used to predict the start and end of transformations for an isothermal heat treatment of a austenite alloy Three curves are drawn - the first represents the time required at various temperatures for transformation to occur, the other represents the time taken for transformation to be completed and the dashed curve indicated 50% of the transformation Pearlite: Bainite: - Formed during the cooling of austenite over the - Microconstituent product of the austenitic eutectoid temperature transformation. Bainite consists of ferrite and cementite - Coarse pearlite - formed when austenite is cooled at phases a higher temperature as diffusion rates are high which - Formed as needles/plates, depending on temperature. No results in the formation of thick lamellae proeutectoid phase - Fine pearlite - formed when austenite is cooled at a - Can only be observed using an electron microscope lower temperature due to the decrease in carbon - Composed of a ferrite matrix with elongated particles of diffusion rate Fe3C - Found in some steels and cast irons - Cannot transform between pearlite and bainite, need to reheat back to austenite Spheroidute: Martensite: - If steel containing pearlitic or bainitic microstructures - Forms when austenitized iron-carbon alloys are rapidly is heated to, and left at, a temperature below the cooled (quenched) to a relatively low temperature. The eutectoid for a sufficiently long period (hrs), product of a diffusionless transformation from austenite. spheroidite will form Quenching rate must be fast enough to prevent carbon - Sphere-like particles embedded in a continuous diffusion alpha-phase matrix - Needle-shaped grains usually in a white austenite matrix - Driven by the reduction in the alpha-Fe3C phase - FCC austenite -> Body centred tetrahedral boundary area - Athermal transformation - transformation that is independent of time, it is a function of temperature - Competes with pearlite and bainite 39 Continuous-cooling transformation diagrams: - Isothermal heat treatments are impractical as alloys need to be rapidly cooled to and maintained at a temperature above eutectoid. This is both difficult and expensive. - Isothermal transformation diagrams - must be at constant temperature - In reality, continuous cooling takes place in which the time required for a reaction to begin and end is delayed. They are used to determine when respective transformations take place as austenite is cooled at a specific rate (changing temperature) - Fine pearline corresponds to rapid cooling whereas coarse pearlite corresponds to slow cooling rates - Critical quenching rate - rate at which the minimum quenching is required to produce a totally martensitic product Mechanical behaviour of iron-carbon alloys: Pearlite: Bainite: - Cementite is harder, but more brittle than ferrite - Bainite has a finer structure, it is stronger and harder - Increasing the cementite in a steel alloy will result in than pearlitic steels a harder, stronger material - Have a desirable combination of strength and - However, increased cementite decreases ductility and ductility toughness (impact energy) - Fine pearlite is stronger than coarse pearlite - cementite has strong and rigid phase boundaries, thus more phase boundaries, restricts more dislocation and thus is stronger. Fine pearlite has more grain boundaries than coarse pearlite, thus is stronger Spheroidite: Martensite: - Less hardness and strength than pearlitic - Hardest and strongest, but the most brittle microstructures as they have less boundary are per - Has negligible ductility unit volume - Strong due to their interstitial carbon atoms which - Plastic deformation is not nearly as constrained, hinder dislocation motion and relatively few slip which results in a soft and weak material systems for the BCT crystal structure - Very ductile and notably tough as cracks encounter a small fraction of brittle cementite in the ferrite matrix Tempered martensite: - Ductility and toughness is enhanced during the tempering process as internal stresses are relieved - Tempering - accomplished by heating a martensitic steel to a temperature below the eutectoid for a specific time period (between 250 and 650℃, to as low as 200℃) - 𝑚𝑎𝑟𝑡𝑒𝑛𝑠𝑖𝑡𝑒(𝐵𝐶𝑇, 𝑠𝑖𝑛𝑔𝑙𝑒 𝑝ℎ𝑎𝑠𝑒)→ 𝑡𝑒𝑚𝑝𝑒𝑟𝑒𝑑 𝑚𝑎𝑟𝑡𝑒𝑛𝑠𝑖𝑡𝑒 (∝ + 𝐹𝑒3𝐶 𝑝ℎ𝑎𝑠𝑒𝑠) - Tempered martensite consists of very small cementite particles in an alpha-ferrite matrix 40 41 CHAPTER 17 - Fabrication and processing of engineering materials Forming operations: - Shape of a metal piece is changed by plastic deformation which is induced by an external force or stress which does not exceed the yield strength of the material - Most metallic materials are amenable due to their ductility which allow for permanent deformation without cracking or fracturing Hot working - deformation is achieved at a temperature above that Cold working - deformation is achieved at a temperature below that at which recrystallization occurs at which recrystallization occurs - Large deformations are possible which can be successively - Increases strength but decreases ductility (strain hardening) repeated - high-quality surface finish, better and greater variety of - Deformation energy requirements are less than for cold mechanical properties, closer dimensional control working - Total deformation is accomplished in a series of steps in - Metals experience some surface oxidation, thus material which the piece is successively cold worked a small amount loss and a poor final surface finish and then process annealed - expensive and inconvenient procedure Forging: Rolling: - Mechanically working or deforming a single piece of a usually - Passing a piece of metal between two rolls; a reduction in hot metal; this may be accomplished by the application of thickness results from compressive stresses exerted by successive blows or by the rolls continuous squeezing - Cold rolling produces sheet, strip, - Either closed or open die and foil with a high-quality - Wrenches, automotive surface finish. crankshafts and piston - Circular shapes, I-beams and connecting rods railroad rails made using grooved rolls Extrusion: Drawing: - A bar of metal is forced through a die orifice by a compressive - Pulling of a metal piece through a die having a tapered force that is applied to a ram; the extruded piece that bore by means of a tensile force that is applied on the exit emerges has the desired shape and a reduced cross-sectional side. A reduction in cross section and increase in length area. - Rod, wire and tubing products - Rods, tubing that have rather complicated cross-sectional geometries and seamless tubing 42 Casting: - A fabrication process in which a completely molten metal is poured into a mould cavity having the desired shape. Upon solidification, the metal takes the shape of the mould but experiences some shrinkage - Method is used when: the finished shape is large or complicated, a particular alloy is low in ductility and when casting is the most economical Sand casting: Die Casting: - Ordinary sand is used as the mould material from which a - Liquid metal is forced into a mould under pressure and at a two-piece mould is formed by packing sand around a pattern relatively high velocity and allowed to solidify with the pressure - A gating system aids the flow of molten metal into the cavity maintained. Uses a two-piece permanent steel mould or die which and minimises internal casting defects is clamped together to form the desired shape - Automotive cylinder blocks, fire hydrants, large pipe fittings. - Rapid casting thus inexpensive method - Single set of dies = thousands of castings - Only produces small pieces with alloys of zinc, aluminium and magnesium, which have low melting temperatures. Investment Casting: (lost wax) Lost-Foam Casting: (expendable pattern) - Pattern is made from a wax/plastic that has a low melting - Pattern is a foam that can be formed by compressing polystyrene temperature. Around the pattern a fluid slurry is poured that beads into the desired shape and bonded together by heating or is set to form a solid mould. The mould is then heated and cut from sheets and assembled with glue. Sand is packed around the pattern melts, leaving behind a mould cavity having the the pattern to form the mould. As the molten metal is poured into desired shape the mould, it replaces the foam which evaporates. - High dimensional accuracy, reproduction of fine detail, and - Complex geometries and tight tolerances, simple, quick, an excellent finish inexpensive and fewer environmental wastes. - Jewellery, dental crowns, blades for gas turbines and jet - Automobile engine blocks, cylinder heads, crankshafts, marine engine impellers engine blocks and electric motor frames. Continuous Casting: - Refined and molten metal is cast directly into a continuous strand that may have either a rectangular or circular cross section. Solidification occurs in a water-cooled die having the desired cross-sectional geometry. This product is then transformed using a forming process - Uniform chemical composition and mechanical properties - Highly automated and efficient. Miscellaneous techniques: Powder Metallurgy: - The compaction of powdered metal followed by a heat treatment to produce a denser piece - Economically produces nonporous pieces with very close dimensional tolerances - Low ductility metals and metals with high melting temperatures can be used Welding: - Two or more metal parts are joined metallurgically to form a single piece when one-part fabrication is expensive or inconvenient - Similar and dissimilar metals may be welded Laser beam welding: noncontact process which eliminates mechanical distortion, rapid, highly automated, low energy input, small and precise welds, large variety of metals can be joined, porosity-free 43 Thermal processing of metals: - Altering the mechanical characteristics of metals and alloys when appropriate heat treatments or thermal processes are used Annealing Processes: - A heat treatment in which a material is exposed to an elevated temperature for an extended time period and then slowly cooled. - Used to relieve stresses, increase softness, ductility, and toughness and/or produce a specific microstructure - Process: 1. Heating to the desired temperature 2. Holding or “soaking” at that temperature 3. Cooling at room temperature. - If the rate of temperature change is too great, temperature gradients and internal stresses may be induced that may lead to warping or even cracking - Annealing time must be long enough to allow for all necessary transformation reactions - Annealing may be accelerated by increasing the temperature because diffusional processes are normally involved. Process Annealing: - Heat treatment used to negate the effects of cold work (soften and increase the ductility of a previously strain-hardened metal) - Allow for a continuation of deformation without fracture or excessive energy consumption. Recovery and recrystallization processes are allowed to occur, but a fine-grained microstructure is desired thus the process is stopped before grain growth occurs - Prevents surface oxidation by annealing at a relatively low temperature (but above the recrystallization temperature) or in a non oxidising atmosphere Stress Relief: - Internal residual stresses may develop in metal pieces due to plastic deformation processes, non uniform cooling and phase transformations. These internal residual stresses may lead to distortion and warpage - Stress relief annealing - heat treatment in which a piece is heated to the recommended temperature, held there long enough to attain a uniform temperature and cooled to room temperature in air. - The annealing temperature is typically relatively low such that cold working and other heat treatments are not reversed Annealing of Ferrous Alloys: Normalising - Normalising is used to refine the grains, which occur in plastic deformation, and produce a more uniform and desirable size distribution; fine-grained pearlitic steels are tougher than coarse-grained ones. - Accomplished by heating at least 55°C above the upper critical temperature. After sufficient time has been allowed for austenitizing, the treatment is terminated by cooling in air Full Anneal - To prepare low-and medium-carbon steels for plastic deformation. - The alloy is treated by heating to a temperature of about 50°C above eutectoid - The alloy is then furnace cooled - Produces coarse pearlite (in addition to any proeutectoid phase) that is relatively soft and ductile Spheroidizing - Prepared Medium-and high-carbon steels that have a microstructure containing coarse pearlite for plastic deformation - Spheroidized steels have a maximum softness and ductility and are easily machined or deformed - Produced: - Heating the alloy at a temperature just below the eutectoid for multiple hours 44 - Heating to a temperature just above the eutectoid temperature and then either cooling very slowly in the furnace or holding at a temperature just below the eutectoid temperature - Heating and cooling alternately within about ± 50°C of the eutectoid - The rate of spheroidite formation depends on prior microstructure (the finer the pearlite, the more rapid the rate). Also, prior cold work increases the spheroidizing reaction rate Heat treatment of steels: - Attempts to form a high content martensite structure - Success depends on: composition of alloy, type and character of quenching medium and the size and shape of the specimen Hardenability: - The influence of alloy composition on the ability of a steel alloy to transform to martensite for a particular quenching treatment - Hardenability is a qualitative measure of the rate at which hardness drops off with distance into the interior of a specimen as a result of diminished martensite content (high hardenability - steel alloy which forms martensite to a large degree throughout the entire interior) - Jominy End-Quench Test - all factors except for alloy composition are held constant on a d25mmx100mm cylinder during austenitization. The lower end of the cylinder is then quenched by a jet of water. Once cooled, Rockwell hardness readings are taken and plotted as a function of position to form a hardenability curve - Jominy distance unit = 1.6mm - The cooling rate decreases with distance from the quenched end, thus softer pearlite forms further from the end. This is due to the increased time which allows for carbon diffusion (may be mixed with martensite and bainite) - Influence of Quenching Medium, specimen size and geometry: - Most rapid quenching: 1. Water, 2. Oil, 3. Air - The larger the surface area to mass ratio of the specimen, the more rapid quenching occurs and the more hardening takes place Precipitation hardening: - the formation of extremely small, uniformly dispersed particles of a second phase within the original phase matrix which is accomplished by phase transformations that are induced by appropriate heat treatments and alloying elements - Requisite features: - an appreciable maximum solubility of one component in the other - a solubility limit that rapidly decreases in concentration of the major component with temperature reduction - Solution heat treatment - all solute atoms are dissolved to form a single phase solid solution, followed by rapid cooling or quenching - Precipitation heat treating - the supersaturated alpha solid solution is heated ordinarily to an intermediate temperature within the alpha + beta two phase region where diffusion rates become appreciable. The beta precipitate phase begins to form as finely dispersed particles (ageing). The alloy is then cooled - Overaging - With increasing time, the strength or hardness increases, reaches a maximum, and finally diminishes. Thus a reduction in strength and hardness occurs after long time periods 45 Fabrication and processing of ceramics: - Becomes more and more viscous in a continuous manner with decreasing temperature - No definite temperature at which the liquid transforms into a solid as with crystalline materials - Glass transition temperature - volume decreases continuously with temperature reduction and a slight decrease in slope of the curve occurs. Below this temperature, the supercooled liquid is called a glass Melting point Viscosity is 10 Pa·s (100 P) The glass is fluid enough to be considered a liquid. Working point Viscosity is 103 Pa·s (103 P) The glass is easily deformed at this viscosity Softening point Viscosity is 4 x 106 Pa·s (4 × 107 P) The maximum temperature at which a glass piece may be handled without causing significant dimensional alterations Annealing point Viscosity is 1012 Pa·s (1013 P) Atomic diffusion is sufficiently rapid that any residual stresses may be removed within about 15 min Strain point 3 x 1013 Pa·s (3 x 1014 P) For temperatures below the strain point, fracture will occur before the onset of plastic deformation. The glass transition temperature will be above the strain point - Most glass-forming operations take place between the working and softening temperatures Glass forming: - Formability of glass - tailored by its composition - Raw materials are heated to above melting point and then formed - Most glasses are of the silica-soda-lime variety - When optical transparency is important - Homogeneity - achieved by complete melting and mixing of raw ingredients - Porosity - results from small gas bubbles which are produced. These need to be absorbed into the melt/eliminated by the proper adjustment of the viscosity of the molten material Methods of glass forming: 1. Pressing - fabrication of relatively thick-walled pieces in which the glass piece is formed by pressure application in a heated graphite-coated cast iron mould having the desired shape 2. Blowing - From a raw gob of glass, a parison (temporary shape) is formed by mechanical pressing in a mould which is then blown to conform to the mould contours. 3. Drawing - follows the general drawing process, long pieces with constant cross-sectional shape are formed 4. Sheet and fibre forming - molten glass passes (on rollers) from one furnace onto a bath of liquid tin located in a second furnace allowing for a flat surface 46 Heat-treating glasses Annealing - Thermal stress - internal stresses as a result of the difference in cooling rate and thermal contraction between the surface and interior regions. These stresses weaken the material or lead to fracture (thermal shock) - Annealing aims to eliminate thermal stresses by heating the glass and cooling it slowly to room temperature Glass Tempering - Strength of a glass piece is enhanced by intentionally inducing compressive residual surface stresses during heat treatment - Glassware is heated to a temperature above the glass transition region but below the softening point. It is then cooled to room temperature in a jet of air/oil bath. - Residual stresses arise from differences in cooling rates for surface and interior regions. Initially, the surface cools more rapidly and becomes rigid. With continued cooling, the interior attempts to contract to a greater degree than the now-rigid exterior will allow. Thus, the inside tends impose radial stress on the outside which allows the cooled product to sustain compressive forces on the surface and tensile stress at interior regions Fabrication and Heat-Treating of Glass-Ceramics - After forming of the glass, it undergoes crystallisation accompanied by heat treatments - After melting and forming operations, nucleation and growth of the crystalline phase particles are carried out isothermally at two different temperatures. Fabrication and processing of clay products: - Hydroplasticity - condition when water is added inducing plasticity (this is important for forming) - Clay melts over a range of temperatures (depending on composition) thus dense and strong ceramic pieces may be produced during firing without complete melting such that the desired shape is maintained - Clays are aluminosilicates composed of alumina (Al2O3) and silica (SiO2) that contain chemically bound water - Have layered crystal structures and often contain impurities such as organic material - When water is added, the water molecules fit between these layered sheets and form a thin film around the clay particles allowing movement over one another - Contain some nonplastic ingredients as filler materials Hydroplastic Forming: - Hydroplasticity causes low yield strengths - Water-clay ratio needs to maintain a yield strength sufficient to maintain shape during handling and drying - Extrusion - stiff plastic ceramic mass is forced through a die orifice having the desired cross-sectional geometry - Brick, pipe, ceramic blocks, and tiles are all commonly fabricated using hydroplastic forming in which air is removed in a vacuum chamber to enhance the density Slip Casting - Slip - a suspension of clay and/or other nonplastic materials in water - When poured into a porous mould, water from the slip is absorbed into the mould, leaving behind a solid layer on the mould wall, the thickness of which depends on the time. - Can be continued until the entire mould cavity becomes solid - Drain casting - casting in which tipping out the excess occurs - As the cast piece dries and shrinks, it pulls away (or releases) from the mould wall allowing for removal - Clay must have a high specific gravity and yet be very fluid and pourable 47 - The cast piece must be free of bubbles, have low drying shrinkage and relatively high strength. - Properties of the mould influence the quality of the casting (normally plaster of Paris is used - economical, easy to fabricate and reusable) - Sanitary lavatory ware, art objects and ceramic tubes. Drying and firing: - Pieces formed by hydroplastic and slip casting retain porosity nd liquid and have insufficient strength for use - Green - a body that has been formed and dried, but not fired - Water is removed during drying and density and strength are enhanced during high-temperature heat treatment/firing - Drying and firing can cause defects such as cracking Drying: - As clay dries and water is removed, interparticle separation decreases and shrinkage occurs - Crate of water removal needs to be controlled as water is pulled (diffused) to the surface and evaporates, the surface cannot dry out before the inside is dry - Evaporation rate is controlled by temperature, humidity and airflow. Microwave energy can also be used to dry products - Shrinkage is enhanced as particle size is decreased Firing: - Green body is fired at temperatures between 900 and 1400℃ - depends on composition and properties of finished piece - Density is increased and mechanical strength is enhanced - Vitrification - gradual formation of liquid glass that forms and fills some of the pore volume. Degree of vitrification depends on firing temperature and time + controls the final product’s room temperature properties - Fused phase flows around remaining unmelted particles and fills in pores due to surface tension forces. Shrinkage also occurs - Fused phase becomes a glassy matrix upon cooling, resulting in a strong body - Complete vitrification is avoided as the body becomes too soft and will collapse Powder pressing: - Similar to powder metallurgy in which powdered ceramic mass which contains a binder is compacted into desired shapes under compression - 1. Uniaxial pressing - powder compacted in a metal die by pressure applied in a single direction. The formed piece takes on the configuration of the die and platens through which the pressure is applied. Can only make simple shapes, but is inexpensive and fast - 2. Isostatic (or hydrostatic) pressing - powdered material is contained in a rubber envelope and pressure is applied isostatically by a fluid. Creates more complicated shapes, but is time consuming and expensive - 1. and 2. require firing - Sintering - changes occur by the coalescence of the powder particles into a denser mass Sintering allows for particles to coalesce into a denser, stronger mass as pores become smaller and more spherical - 3. Hot pressing - the powder pressing and heat treatment are performed simultaneously. Used for materials that do not form a liquid phase except at very high and impractical temperatures and when high densities without appreciable grain growth are desired. Expensive, time consuming, costly moulds with short lifespan Tape casting: - thin sheets of a flexible tape are produced by means of a casting process in which sheets are prepared from slips - Contains binders and plasticizers coupled with de-airing to increase strength - Poured onto a flat surface, spread thin by doctor blade until uniform thickness Synthesis and processing of polymers: - Properties of a polymer may be modified and enhanced by the inclusion of additive materials - Finished piece having a desired shape must be fashioned during a forming operation 48 Polymerization: - Large macromolecules of the commercially useful polymers must be synthesised from substances having smaller molecules - A process by which monomers are linked together to generate long chains composed of repeat units Addition polymerisation: - A process by which monomer units are attached one at a time in chainlike fashion to form a linear macromolecule - Composition of the resultant product molecule is an exact multiple of that of the original reactant monomer - Stages: - Initiation - active centre capable of propagation is formed by a reaction between an initiator (or catalyst) species and the monomer unit - Propagation - the linear growth of the polymer chain by the sequential addition of monomer units - Termination - active ends of two propagating chains may link together to form one molecule or two growing molecules react to form two “dead chains” - Molecular weight governed by the relative rates of stages and is controlled to ensure the production of a polymer having the desired degree of polymerization Condensation polymerization: - The formation of polymers by stepwise intermolecular chemical reactions that may involve more than one monomer species - Low-molecular-weight by-product is eliminated (condensed) - Reaction time is longer than for addition polymerisation Polymer additives: Fillers Improve tensile and compressive strength, abrasion resistance, toughness, dimensional and thermal stability Wood flour, silica flour, sand, glass etc. Plasticizers Improves flexibility, ductility and toughness and reduces hardness and stiffness Used for polymers that are brittle at room temperature for thin sheets or films, tubing, raincoats, and curtains Stabilisers Counteract deteriorative processes ie. sunlight exposure and oxidation Colourants Impart a specific colour to a polymer. Do not dissolve in polymer Flame retardants Enhances flammability resistance of combustible polymers Forming techniques: - Depend on thermoplastic/thermosetting, temperature at which polymer softens, atmospheric stability of formed material and the geometry and size of product - Normally occurs at elevated temperatures and often by the application of pressure - Thermoplastics - formed above their glass transition temperatures (if amorphous) and above melting temperature (if semicrystalline) - Thermoplastics can be recycled, thermosetting products are difficult to recycle - Thermosetting products - preparation of linear polymer as a liquid with a low molecular weight which is then converted into the final hard and stiff product (curing) as crosslinked/network structures form - Elastomers can be vulcanised and reinforced by carbon black, follow the same fabrication processes as plastics 49 Compression and transfer moulding: - Compression - Appropriate amounts of thoroughly mixed polymer and necessary additives are placed between male and female mould members. Both mould pieces are heated, but only one is movable. Mould is closed, heated and pressure is applied, causing the plastic to become viscous and flow to conform to the mould shape - Suitable for thermosetting and thermoplastic - Transfer - solid ingredients are first melted in a heated transfer chamber (thermosetting polymers and complex geometry pieces) Injection moulding: - Thermoplastics (but can be used for thermosetting, just takes longer) - Pelletized polymer is fed from a hopper, through a heating chamber where pellets melt. A ram compels melted polymer into an enclosed mould. Pressure maintained until mould has solidified - Very fast Extrusion: - Viscous, molten polymer is forced through a die orifice of desired cross-sectional shape - Solidification aided by blowers/water spray/bath Blow Moulding: - A parison, length of polymer tubing, is extruded. While still semi molten, it is placed in a two-piece mould. Air/steam is blown under pressure is blown, forcing the tube walls to conform to the contours of the mould - Temperature and viscosity must be regulated carefully Casting: - Molten plastic material is poured into a mould and allowed to solidify. - Both thermoplastic and thermosetting plastics - For thermoplastics, solidification occurs upon cooling from the molten state - For thermosets, hardening is a consequence of polymerization or curing process Fabrication of fibres and films: Fibres: - Spinning - process by which fibres are formed from bulk polymer material - Spun from the molten, viscous state in a process called melt spinning and is pumped through a spinneret with multiple small holes. Thus, a single, rapidly cooled fibre is formed - Crystallinity of a spun fibre depends on its rate of cooling during spinning - Strength enhanced by drawing Films: - Extruded through a thin die slit, followed by rolling/drawing operation which reduces thickness and improves strength - Can also be blown as continuous polymer tube expands around hot air bubble, while being drawn simultaneously 50 CHAPTER 10 - Failure - Need to be prevented as failure puts human life at risk, causes economic loss and interferes with the availability of products and services - Simple fracture - the separation of a body into two or more pieces in response to an imposed static stress at temperatures that are low relative to the melting temperature of the material - Ductile metals - typically exhibit substantial plastic deformation with high energy absorption before fracture - Brittle metals - little or no plastic deformation with low energy absorption before fracture - Ductility - quantified in terms of percent elongation and percent reduction in area and is a function of temperature of the material, the strain rate and the stress state - Fracture process: crack formation and propagation in response to an imposed stress - Ductile fracture - characterised by extensive plastic deformation in the vicinity of an advancing crack, in which the process proceeds relatively slowly as the crack length is extended. Stable cracks - resists any further extension unless there is an increase in the applied stress. There is typically evidence of gross deformation at the fracture surfaces - Brittle fracture - cracks may spread extremely rapidly, with very little accompanying plastic deformation. Unstable cracks in which crack propagation, once started, continues spontaneously without an increase in magnitude of the applied stress - Ductile fracture is preferred to brittle fracture as ductile fracture gives a warning of failure and more strain energy is required to induce ductile fracture Ductile fracture: Process: 1. Small cavities (microvoids) form int the interior of the cross section 2. Microvoids enlarge and come together coalesce to form an elliptical crack (long axis perpendicular to stress direction) 3. Fracture ensues by the rapid propagation of a crack around the outer perimeter of the neck by shear deformation at an angle of about 45 degrees with the tensile axis (shear stress at max) - called cup and cone fracture Internal surface has irregular and fibrous appearance and has dimples Brittle fracture: - Takes place without any appreciable deformation by rapid crack propagation - Direction of crack motion - very flat and nearly perpendicular to the direction of the applied tensile stress - Chevron lines (v-shaped markings) form near the centre of the fracture cross section that point to the crack initiation site - Contain lines/ridges that radiate from the origin of the crack in a fanlike pattern - Transgranular crack - crack propagation corresponds to the successive and repeated breaking of atomic bonds along specific crystallographic planes (cleavage). Fracture cracks pass through grains - Intergranular crack - crack propagation is along grain boundaries usually subsequent to the occurrence of processes that weaken or embrittle grain boundary regions 51 Principles of fracture mechanics: - Fracture mechanics - quantification of the relationships among material properties, stress level, the presence of crack-producing flaws, and crack propagation mechanisms Stress Concentration: - Microscopic flaws or cracks exist under normal conditions at the surface and within the interior of a body of material are stress raisers (mainly at the crack tip) - The magnitude of this localised stress decreases with distance away from the crack tip - Stress amplification is not restricted to these microscopic defects; it may occur at macroscopic internal discontinuities, sharp corners, scratches, and notches - The effect of a stress raiser is more significant in brittle than in ductile materials Fracture toughness: - A measure of a material’s resistance to brittle fracture when a crack is present - Plane strain - load operates on a crack in a manner which there is no strain component perpendicular to the front and back faces - Fracture toughness testing: Impact testing: - Charpy and Izod tests measure impact energy of prepared samples which have notches in them - Specimen: 10 x 10 x 8mm with a notch - Impact energy - a measure of the energy absorbed during fracture of a standard specimen when subjected to rapid loading - Ductile-to-brittle transition - measured by impact tests to determine whether/over what - Temperature range a material undergoes ductile to brittle transition with decreasing temperature 52 Fatigue: - A form of failure that occurs in structures subjected to dynamic and fluctuating stresses - Possible for failure to occur at a stress level considerably lower than the tensile or yield strength for a static load - Occurs after a lengthy period of repeated stress or strain cycling - Occurs suddenly without warning, usually brittle-like in nature and happen to brittle materials Cyclic stresses: - Applied stress may be axial (tension–compression), flexural (bending) or torsional (twisting) in nature - Fluctuating stress-time modes: 1. Reversed, 2. Repeated, 3. Random S-N curve: - test apparatus should be designed to duplicate as nearly as possible the service stress conditions - rotating-bending beam: alternating tension and compression stresses of equal magnitude are imposed on the specimen as it is simultaneously bent and rotated - Stress cycle is reversed - R = -1 - Records the number of cycles until failure at a range of stresses - The recorded data is plotted on a graph as stress versus the number of cycles to failure (logarithm) - The higher the magnitude of the stress, the smaller the number of cycles the material is capable of sustaining before failure - Fatigue limit - largest value of fluctuating stress that will not cause failure for essentially an infinite number of cycles, below which fatigue failure will not occur. Indicated by a horizontal plateau - Fatigue strength - the stress level at which failure will occur for some specified number of cycles - Fatigue life - the number of cycles to cause failure at a specified stress level Crack initiation and propagation: - Fatigue failure steps: 1. Crack initiation, 2. Crack propagation, 3. Final failure - Cracks associated with fatigue failure almost always initiate on the surface of a component at some point of stress concentration - Beachmarks - Each band represents a period of time over which crack growth has occurred. Macroscopic (can see with naked eye) and one benchmark consists of thousands of striations. Show on components that experienced interruptions during crack propagation. - Striations - Each represents the advance distance of a crack front during a single stress cycle. Striation width depends on, and increases with, increasing stress range. These marks are microscopic. - Both beachmarks and striations do not appear on regions over which rapid failure occurred - Presence confirms fatigue, absence does not confirm not fatigue 53 - During the propagation of fatigue cracks and on a microscopic scale, there is localised plastic deformation at crack tips, even though the maximum applied stress to which the object is exposed in each stress cycle lies below the yield strength of the metal - Applied stress is amplified at crack tips to the degree that local stress levels exceed the yield strength Factors that affect fatigue life: Mean stress - increasing the mean stress level leads to a decrease in fatigue life Surface effects - maximum stress within a component or structure occurs at its surface Reducing the probability of normal fatigue failure: - Design factors - Any notch or geometrical discontinuity can act as a stress raiser and fatigue crack initiation site, the sharper the discontinuity (the smaller the radius of curvature), the more severe the stress concentration Can be avoided by modifying designs with sudden contour changes leading to sharp corners - Surface treatments - improving the surface finish by polishing enhances fatigue life significantly. Imposing residual compressive stresses within a thin outer surface layer also increases fatigue performance by: Shot peening - projecting small, hard particles onto surface at high velocities Case hardening - A carbonaceous or nitrogenous rich outer layer is introduced by atomic diffusion from the gaseous phase Environmental effects: Thermal fatigue - induced at elevated temperatures by fluctuating thermal stresses (mechanical stresses from an external source do not need to be present) - Thermal stresses do not arise if mechanical restraint is absent - Reduced by: allowing unhindered dimensional changes with temperature variations (less mechanical restraint) or to choose materials with appropriate physical properties. Corrosion fatigue - failure that occurs by the simultaneous action of a cyclic stress and chemical attack - Small pits may form as a result of chemical reactions between the environment and the material, which may serve as points of stress concentration and therefore as crack nucleation sites. Enhanced in corrosive environment - Reduced by: applying protective surface coatings, selecting a more corrosion-resistant material, and reducing the corrosiveness of the environment or reducing the probability of normal fatigue failure as mentioned above Creep: - the time-dependent and permanent deformation of materials when subjected to a constant load or stress which limits lifetime of parts - Metals - usually occurs are temperatures 0.4*melting temperature - Amorphous polymers (plastics and rubbers) are prone to creep - Typical creep test - subjecting a specimen to a constant load or stress while maintaining the temperature constant; deformation or strain is measured and plotted as a function of elapsed time 1. Primary/transient creep - occurs first, typified by a continuously decreasing creep rate (the slope of the curve decreases with time). This suggests that the material is experiencing an increase in creep resistance or strain hardening 2. Secondary/steady-state creep - the rate is constant (plot becomes linear). Stage of longest duration. Balance between the competing processes of strain hardening and recovery (recovery - the process by which a material becomes softer and retains its ability to experience deformation) 3. Tertiary creep - acceleration of the rate and ultimate failure (rupture) which results from microstructural and/or metallurgical changes leading to a decrease in the effective cross-sectional area and an increase in strain rate 54 Alloys for high temperature use: - Factors that affect creep: melting temperature, elastic modulus and grain size - The higher the melting temperature of a material, the greater the elastic modulus; the larger the grain size, the better a material’s resistance to creep - Grain-size - smaller grains permit more grain boundary sliding, which results in higher creep rates (contrasted to the influence of grain size on