Wave and Tidal Energy PDF
Document Details
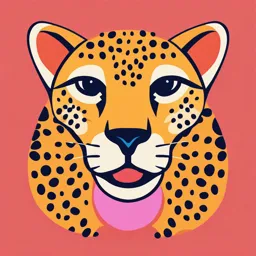
Uploaded by LuxuriousLagoon
Università degli Studi di Padova
D. Del Col
Tags
Summary
This document provides an overview of wave and tidal energy technologies. It explores different types of wave energy converters and discusses the exploitation of wave and tidal resources. The document also covers the theoretical background behind the different technologies of wave energy converter.
Full Transcript
UNIVERSITẦ DEGLI STUDI DI PADOVA LM Energy engineering Renewable Energy Technologies Wave and tidal energy D. Del Col Sources: Arthur Pecher, Jens Peter Kofoed,...
UNIVERSITẦ DEGLI STUDI DI PADOVA LM Energy engineering Renewable Energy Technologies Wave and tidal energy D. Del Col Sources: Arthur Pecher, Jens Peter Kofoed, Ocean Wave Energy, Springer Deborah Greaves, Gregorio Iglesias, Wave and Tidal Energy, John Wiley & Sons The Wave Resource There exist many types of waves in the ocean, with different driving mechanisms and properties. However, the term wave energy does not refer to all of them, but to a particular type of waves: wind waves, those generated by the wind blowing over the sea. Winds are ultimately the result of solar energy acting on the atmosphere, and therefore wave energy may be regarded as a concentrated form of solar energy – and the oceans, as a gigantic energy conversion system transforming one type of mechanical energy (wind energy) into another, more concentrated type (wave energy). Wave Energy Resource Considering that the oceans cover 71% of the Earth’s surface and that wind waves can propagate over long distances with little energy loss, a substantial amount of wave power reaches almost continuously any stretch of coastline open to the ocean. This quasi‐ubiquity of wave power inshore constitutes one of its principal advantages, and explains the enormous, virtually unlimited global resource – which has been estimated at 32,000 TWh per year (see for comparison figure on the right displaying TWh vs. year). Wave energy has great potential for development, and its present status is reminiscent of wind energy in the 1980s. The deployment of arrays of WECs (wave energy converters) to harness this vast, untapped resource is hindered by a number of factors, not least the lack of maturity of the technology and the concomitant high costs. Wave Energy Converters Wave Energy Converters Wave Energy Converters Wave Energy Converters: Oscillating Water Column Oscillating Water Columns are conversion devices with a semi-submerged chamber, keeping a trapped air pocket above a column of water. Waves force the air out of the chamber and back into it. This continuous movement generates a reversing stream of high-velocity air, which drives an air turbine-generator group to produce electricity. They generally have fixed structure (but not always). A big advantage is the simplicity (no moving parts other than the turbine). A disadvantage is the low performance level. Wave Energy Converters: Oscillating Water Column fixed structure floating structure Wave Energy Converters: Oscillating Water Column 16 air chambers that are 4.5m wide, 3.1m depth, and 10m high 18.5 kW (Wells turbine) for each air chamber The Wells turbine is a low-pressure air turbine that rotates continuously in one direction independent of the direction of the air flow. Its blades feature a symmetrical airfoil with its plane of symmetry in the plane of rotation and perpendicular to the air stream. It was developed for use in Oscillating Water Column wave power plants, in which a rising and falling water surface moving in an air compression chamber produces an oscillating air current. The use of this bidirectional turbine avoids the need to rectify the air stream by delicate and expensive check valve systems. Its efficiency is lower than that of a turbine with constant air stream direction and asymmetric airfoil. One reason for the lower efficiency is that symmetric airfoils have a higher drag coefficient than asymmetric ones, even under optimal conditions. The Wells turbine was developed by Prof. Alan Arthur Wells of Queen's University Belfast in the late 1970s. Wave Energy Converters: Oscillating Water Column Oscillating water columns (floating), Ocean Energy Buoy Ireland Wave Energy Converters Wave Energy Converters Wave activated bodies (WABs) or Oscillating body converters (OBCs) The category of wave activated bodies (WABs) encompasses a very large field of WEC concepts. Here a number of examples are given to give an impression of the plurality, but it cannot be considered complete as the number of concepts in this category can be counted in hundreds. Oscillating Body Converters are either floating (usually) or submerged (sometimes fixed to the bottom). They exploit the more powerful wave regimes They are more complex than OWCs , particularly with regards to their PTO systems (the power take off system by which mechanical energy is converted into electrical energy; e.g., hydraulic generators with linear hydraulic actuators, linear electric generators, etc.) The advantages of oscillating body converters include their size and versatility since most of them are floating devices. Wave Energy Converters the heave plate is kept in the position by means of mooring buoys Ocean Power Technologies (OPT) (Fig. 12) is one among a number of technologies utilizing a point absorber. The OPT PowerBuoy is using a reference plate as point of reference for the PTO (power take off ). OPT has used different solutions for PTO, including oil hydraulics. OPT is working on a range of deployment projects, and have conducted sea trials using both a 40 kW and 150 kW version of their technology Wave Energy Converters SeaBased uses a fixed reference point at the seabed, where also the PTO, a linear generator, is placed (Fig. 14) Wave Energy Converters Wave Energy Converters Wave Energy Converters Wave Energy Converters Wave Energy Converters: Overtopping Devices Overtopping converters (or terminators) consist of a floating or bottom fixed water reservoir structure. As waves arrive, they spill over the top of a ramp structure and are restrained in the reservoir of the device. The potential energy , due to the height of collected water above the sea surface, is transformed into electricity using conventional low head hydro turbines (similar to those used in mini hydro plants). The main advantage of this system is the simple concept: it stores water and when there is enough, it lets it pass through a turbine. The main disadvantages are the low head (in the order of 1-2 m) and the big size of a full-scale over topping device. Wave Energy Converters: Overtopping Devices The Wave Dragon is a slack moored WEC utilizing the overtopping principle (Fig. 24). The structure consists of a floating platform with an integrated reservoir and a ramp. The waves overtop the ramp and enter the reservoir, where the water is temporarily stored before it is led back to the sea via hydro turbines generating power to the grid, and thereby utilizing the obtained head in the reservoir. Furthermore, the platform is equipped with two reflectors focusing the incoming waves towards the ramp, which thereby enhance the power production capability. Wave Energy Converters: Overtopping Devices Other overtopping based approaches do also exist, including the SSG, which is a fixed structure acting as a combination of a WEC and a breakwater (Figs. 25). In order to still being able to harvest the wave power with good efficiency, while not having the option of adjusting the ramp height through the floating level, SSG consists of multiple reservoirs with different heights. Exploitation of the Wave Resource For wave energy to truly take off, development on four fronts is necessary: 1. robust and efficient WECs must be developed 2. the resource must be thoroughly characterized so that the areas of interest can be selected and the energy output of prospective wave farms determined 3. the economics of these farms (groups of WECs) must be assessed, which requires a thorough understanding of the lifetime costs of the WECs themselves and the other components of a wave farm, including their installation, maintenance and decommissioning 4. the impacts of wave energy on marine and, in particular, coastal environments must be assessed at different time scales, from the short to the long term. Waves Generally speaking, the nearshore wave resource is less uniform than its offshore counterpart, the reason being that waves do not interact with the seabed in deep water. h Ocean waves propagate over deep water until, eventually, they approach a coast. When the water depth decreases to half the wavelength, i.e. when h the relative water depth, h/L = ½ h (with h the water depth and L the wavelength), the wave begins to interact with the seabed and, by definition, leaves deep water to enter transitional water. h h h How much energy is in the waves? Linear Wave Theory In linear wave theory, also known as Airy wave theory, the (sinusoidal) displacement of the free surface (the sea surface) is given by where η is the vertical excursion of the free surface, H is the wave height (vertical distance from the wave crest to the wave trough), L the wavelength (distance between two similar points of the wave), and T the wave period (the time taken for the wave to repeat). Amplitude L Wavenumber Angular frequency Linear Wave Theory: Dispersion relationship An important characteristic of ocean waves is that they are generally dispersive, which means that the energy in the wave does not travel at the same velocity as the wave profile. The effect of dispersion can be seen when a stone is dropped into water or the wave paddles in a wave tank stop generating waves. In this case waves appear to be left behind the main wave and are travelling at a slower velocity than the wave crests due to the wave energy. The velocity of a wave crest is typically called the wave celerity, c, and the velocity of the energy propagation is typically called the group velocity, cg Being h water depth, g gravity acceleration or for a given wave period at a certain point (water depth h), there exists only one wavelength that satisfies the equation. In other words, at a certain point the wave period determines the wavelength, or viceversa. For a given wave period, the greater the water depth, the longer the wave. As waves approach the coast, the wave period may be assumed to remain constant; therefore, as the water depth reduces, so does the wavelength. Eventually, the water depth becomes too small for the wave to be viable, at which point it breaks. Linear Wave Theory: Dispersion relationship Wave celerity is the speed at which the wave form (i.e., the wave crest, trough, etc., but not the wave energy) travels, being we obtain For a given water depth, the greater the wave period, the greater the wave celerity, i.e. the faster the wave. This property, dispersion, is of great importance in wave theory. Linear Wave Theory: wave energy Wave energy density is the amount of energy per unit surface area averaged over the wave period, where ρ is seawater density (as a first approximation, ρ ~ 1025 kg m–3). In the SI wave energy density is expressed in (J m–2). The term density alludes to the fact that the energy is measured per unit surface area in the reference plane (i.e., the quiescent sea surface). It can be proven that the wave energy density is composed of kinetic and potential energy, with equal shares: 50% of kinetic and 50% of potential energy. Importantly, the wave energy density varies with the square of the wave height, so a 2 m wave has four times as much energy density as a 1 m wave. Linear Wave Theory: wave energy Group celerity is the speed at which wave energy propagates, At the surface n=1 In deep water n=1/2 Wave power, also known as wave energy flux, is the amount of energy that passes per unit time through a vertical section of unit width perpendicular to the direction of propagation (i.e., parallel to the wave fronts); it is given by In the SI wave power has units of (W m–1 or kW m–1). The wave power over a certain width, b, of wave front may be obtained from This quantity is useful in establishing the amount of wave power that is available to a WEC, and is the basis of the concept of capture width. So far we have covered linear wave theory. This theory applies only to certain wave conditions. The Tidal Resource The tide is the periodic oscillation of the sea level caused by the gravitational attraction of the Moon and Sun acting on the water particles in the hydrosphere. The force driving the tide is controlled by the complex motion of the Earth–Moon–Sun system. In spite of the much larger mass of the Sun, solar attraction plays a lesser role, approximately 46% of lunar attraction, due to the greater distance from the Earth to the Sun than to the Moon. Tides are different across the globe, with many coastal regions experiencing only very weak tides (e.g. enclosed seas such as the Mediterranean) while others are subjected to large tidal oscillations. In sum, the tide is a complex phenomenon driven by astronomical forces but controlled locally by the bathymetry and coastline geometry of the estuary, gulf, or sea concerned, and of the ocean basin to which it is connected. The Tidal Resource There are essentially two methods for harnessing the energy of the tide. The first consists of building a tidal barrage or lagoon in an estuary or other semi‐enclosed body of water with a significant tidal range. With the barrage sluices closed, a difference in elevation is established between the two sides of the barrage or lagoon as the tidal level rises or falls on the sea (outer) side. Eventually, the sluice gates are open, and the flow through them drives turbine‐generator groups. The second method consists of deploying turbines in the free flow of water caused by the tide (the tidal stream) to harness its kinetic energy. The potential energy of the water mass impounded by a tidal barrage or lagoon has been used since antiquity. Modern‐day applications to generate electricity include the tidal power stations at La Rance (France) and Sihwa Lake (South Korea), which have operated since 1966 and 2011, respectively, with rated power of 240 MW and 254 MW. For all their interest, tidal barrages have a number of disadvantages which may hinder prospective developments: the number of potential sites is very limited, their environmental impact is potentially considerable and a large capital investment is required before the first kilowatt‐hour is produced. These disadvantages are mitigated in the case of tidal lagoons, and they are currently under consideration for certain areas (S Wales, UK). In any case, many more sites are available for tidal stream farms, their environmental impact is lower, and the financial aspects are less challenging. The Tidal Resource -Tidal Range Tidal range can be defined as the difference in vertical height of the water level between its highest and lowest points, and tidal range generation extracts energy by harnessing the potential energy which is available through the difference in water height, defined as where ρ is the density (kg m–3), A is the area of the basin (m2), g is acceleration due to gravity (m s–2) and h is the head (m). There are two types of civil tidal range systems: barrages and lagoons. These differ in that a barrage creates an impoundment across an estuary, while a lagoon is a standalone impoundment and so can be sited completely offshore. The Tidal Resource - Tidal Range Tidal range technologies harvest the potential energy created by the difference in head between ebb tide and flood tide. Tidal range energy is predictable: the energy production is not influenced by weather conditions, but rather by the cyclical constellations, the gravity of the moon, sun and earth, providing a predictable cycle. The Tidal Resource - Tidal Range One-way power generation at ebb tide The reservoir is filled at flood tide through sluice gates or valves that are closed once the tide has One-way power generation at flood tide reached its highest level. At flood tide the sluice gates are kept closed At the ebb tide, the water in the reservoir is to isolate the reservoir while at its lowest level. released through the turbines and power is When the tide is high, the water from the sea- generated. side flows into the reservoir via the turbines, With this single cycle, power is generated for thus generating power. only few hours per day. The disadvantage of this cycle is that it has less capacity and generates less electricity It may be ecologically disadvantageous as the water level in the impoundment is kept at a low level for a long time. The Tidal Resource - Tidal Range Two-way power generation Both incoming and outgoing tides generate power through the turbines. Reversible turbines are required and can also operate as pumps in order to optimize the power production COMPOUNDED AREA OR BAYS AND ESTUARIES OCEAN The Tidal Resource - Tidal Stream In contrast to barrages and tidal lagoons, tidal stream turbines (TSTs) use the kinetic energy of the tide directly, and unlike the impoundment schemes, TSTs allow the water to pass through and around them and do not require the storage of water. Therefore local tide velocity, turbulence, bathymetry, water column velocity profile and depth, seabed mounting, local shipping requirements and concerns associated with marine fishes and mammals are just some of the key issues facing the successful development of tidal stream devices. Much of the fundamental technology associated with TSTs, however, is derived from the wind industry which in some respects circumvents much of the early developments phases, such as blade profile testing and the basics of the ensuing hydrodynamics. The medium in which TSTs operate, however, produces higher structural loading, when compared with air driven turbines, with the addition of biological fouling from marine life, possible impact from marine creatures and flotsam, increased material corrosion from salts and the possibility of blade cavitation at shallower water depths. The Tidal Stream Resource The tidal stream resource may be defined as the kinetic energy in tidal currents, i.e. horizontal motions of water caused (primarily) by the tide. The tidal stream power through a cross‐section Ω normal to the flow is the flux of kinetic energy through that section, where v is the magnitude of the flow velocity (the speed of the current), AΩ is the surface area of section Ω (normal to the flow), ρ is the density of water. The speed is assumed uniform over Ω. This is the power available in the tidal stream flow; however, not all of this tidal stream power can be transformed into mechanical power by the turbine, due to Betz’s law and the hydrodynamic and mechanical losses. Technology developments are comparable to the development of wind turbines. The most used are horizontal axis axial turbine but there are solutions with vertical axis turbines The Tidal Stream Resource The tidal stream power density may be defined as the tidal stream power passing through a unit surface area normal to the flow, where p has SI units of watts per square metre. The tidal stream power available to a turbine may be obtained by multiplying the tidal stream power density by the rotor (swept) area. If the variation of the flow speed, v, with time is known (typically through numerical modelling), then the power density may be integrated with respect to time to obtain the tidal stream energy density: where the interval of integration considered, (t1,t2), may be a typical one year. The tidal stream energy density may be expressed in joules per square metre or kilowatt‐hours per square metre. Tidal Stream Turbines the Betz limit for a tidal stream turbine in a free flow is 59.3%. Tidal Stream Turbines Tidal Stream Turbine tip speed ratio Cardiff Tidal Turbine Tidal Stream Turbine Tip Speed Ratio