Liver Physiology PDF
Document Details
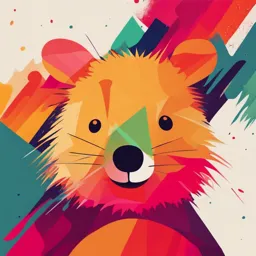
Uploaded by InstructiveTheme
BAU Medical School
Mehmet Ozansoy, Ph.D
Tags
Related
- Liver Anatomy and Physiology Lecture Notes PDF
- Liver Physiology Lecture Notes PDF
- Anatomy & Physiology II - Digestive System II - PDF
- The Functional Anatomy and Histology of the Gastrointestinal Tract Including the Liver and the Pancreas PDF MBS 232 2020
- Lecture 21.1: Liver Anatomy and Function PDF
- Liver and Pancreas Anatomy and Function PDF
Summary
This presentation discusses liver physiology, including its major functions, the overview of the liver, bile secretion and its role, and uptake, processing, and secretion of compounds by hepatocytes. It also covers cholestasis and gallstones along with different types of jaundice.
Full Transcript
LIVER PHYSIOLOGY MEHMET OZANSOY, Ph.D. Dept. of Physiology OVERVIEW After the skin, the liver and the brain are the largest organs in the human body. The liver weighs between 1200 and 1500 g, representing 2% to 5% of body weight in the adult and ~4% to 5% in the newborn. The liver is strategic...
LIVER PHYSIOLOGY MEHMET OZANSOY, Ph.D. Dept. of Physiology OVERVIEW After the skin, the liver and the brain are the largest organs in the human body. The liver weighs between 1200 and 1500 g, representing 2% to 5% of body weight in the adult and ~4% to 5% in the newborn. The liver is strategically situated in the circulatory system to receive the portal blood that drains the stomach, small intestine, large intestine, pancreas, and spleen. In this position, the liver plays a key role in handling foodstuffs assimilated by the small intestine. However, the liver’s role is far more diverse; it serves as a chemical factory, an excretory system, an exocrine gland, and an endocrine gland. OVERVIEW A major function of the liver is to metabolize, detoxify, and inactivate both endogenous compounds (e.g., steroids and other hormones) and exogenous substances (e.g., drugs and toxins). In addition, by virtue of its large vascular capacity and abundance of phagocytes (Kupffer’s cells), the liver provides an important filtering mechanism for the circulation by removing foreign particulate matter, including bacteria, endotoxins, parasites, and aging red blood cells. The liver has the capacity to convert important hormones and vitamins into a more active form. Examples include the initial hydroxylation of vitamin D and the deiodination of the thyroid hormone thyroxine (T4) to triiodothyronine (T3). Moreover, numerous enzymes in the liver process lipophilic chemicals into more polar, water-soluble metabolites, which are more readily excreted into bile OVERVIEW Bile is a complex secretory product produced by the liver. Biliary secretion has two principal functions: elimination of many endogenous and exogenous waste products from the body, such as bilirubin and cholesterol promotion of digestion and absorption of lipids from the intestine. OVERVIEW The products of digested food, including carbohydrates, peptides, vitamins, and some lipids, are extracted from portal blood by the liver. Depending on the metabolic requirements of the body, these substrates may be stored by the hepatocytes or released into the bloodstream either unbound (e.g., glucose) or associated with a carrier molecule (e.g., a triglyceride molecule complexed to a lipoprotein). The liver also synthesizes—in a highly regulated fashion— many substances that are essential to the metabolic demands of the body. These substances include albumin and other plasma proteins, glucose, cholesterol, fatty acids for triglyceride biosynthesis, and phospholipids. The liver must provide a supply of substrates as fuels for other organs, particularly in the fasted state. For example, the liver produces ketone bodies, which can be used by the central nervous system during periods of fasting, thereby sparing ~50% of the amount of glucose that would otherwise be used by this tissue. Hepatocytes: Secretory epithelial cells Hepatocytes account for ~80% of the parenchymal volume in human liver. Hepatocytes form an epithelium, one cell thick, that constitutes a functional barrier between two fluid compartments with differing ionic compositions: the tiny canalicular lumen containing bile and the much larger sinusoid containing blood Hepatocytes significantly alter the composition of these fluids by vectorial transport of solutes across the hepatocyte. This vectorial transport depends critically on the polarized distribution of specific transport mechanisms and receptors that are localized to the apical membrane that faces the canalicular lumen and the basolateral membrane that faces the pericellular space between hepatocytes and the blood-filled sinusoid The space of Disse, or perisinusoidal space, is the extracellular gap between the endothelial cells lining the sinusoids and the basolateral membranes of the hepatocytes. These basolateral membranes have microvilli that project into the space of Disse to facilitate contact with the solutes in sinusoidal blood. The microvilli greatly amplify the basolateral membrane, which accounts for ~85% of the total surface area of the hepatocyte. Slightly more than 6% of the volume of the liver parenchyma is made up of cells other than hepatocytes, including endothelial cells (2.8%), Kupffer’s cells (2.1%), and stellate cells (fat-storing or Ito’s cells, 1.4%) The endothelial cells that line the vascular channels or sinusoids form a fenestrated structure with their bodies and cytoplasmic extensions. Plasma solutes, but not blood cells, can move freely into the space of Disse through pores, or fenestrae, in the endothelial cells Kupffer’s cells are present within the sinusoidal vascular space. This population of fixed macrophages removes particulate matter from the circulation. Stellate cells are in the space of Disse and are characterized morphologically by the presence of large fat droplets in their cytoplasm. These cells play a central role in the storage of vitamin A, and evidence suggests that they can be transformed into proliferative, fibrogenic, and contractile myofibroblasts. On liver injury, these activated cells participate in fibrogenesis through remodeling of the extracellular matrix and deposition of type I collagen, which can lead to cirrhosis. The blood supply to the liver has two sources. The portal vein contributes ~ 75% of the total circulation to the liver; the hepatic artery contributes the other 25%. Blood from portal venules and hepatic arterioles combines in a complex network of hepatic sinusoids. Blood from these sinusoids converges on terminal hepatic venules (or central veins), which, in turn, join to form the hepatic veins. Branches of the portal vein, hepatic artery, and a bile duct (i.e., the triad), as well as lymphatics and nerves, travel together as a portal tract. The classic hepatic lobule includes all hepatocytes drained by a single central vein, and it is bounded by two or more portal triads. Alternatively, we can view the liver as though the triad is the core of a portal lobule. Thus, the portal lobule includes all hepatocytes drained by a single bile ductule and is bounded by two or more central veins. A third way of viewing the liver is to group the hepatocytes according to their supply of arterial blood. Thus, the portal acinus is a small, three-dimensional mass of hepatocytes that are irregular in size and shape, with one axis formed by a line between two triads (i.e., high PO2) and another axis formed by a line between two central veins (i.e., low PO2). A zonal relationship exists between cells that constitute the portal acini and their blood supply. Hepatocytes close to the vascular core formed by the terminal portal venule and terminal hepatic arteriole are perfused first and thus receive the highest concentrations of oxygen and solutes. These periportal hepatocytes are said to reside in zone I, and as a consequence of their location, they are the most resistant to the effects of circulatory compromise or nutritional deficiency. These cells are also more resistant to other forms of cellular injury and are the first to regenerate. Hepatocytes in the intermediate zone II and the most distal population of pericentral hepatocytes located near the terminal hepatic venule (central vein) in zone III are sequentially perfused with blood that is already modified by the preceding hepatocytes Thus, they are exposed to progressively lower concentrations of nutrients and oxygen. BILE SECRETION The canaliculi into which bile is secreted form a three-dimensional polygonal meshwork of tubes between hepatocytes, with many anastomotic interconnections. From the canaliculi, the bile enters the small terminal bile ductules (i.e., canals of Hering), which have a basement membrane and in cross section are surrounded by three to six ductal epithelial cells or hepatocytes. The canals of Hering then empty into a system of perilobular ducts, which, in turn, drain into interlobular bile ducts. The interlobular bile ducts form a richly anastomosing network that closely surrounds the branches of the portal vein. These bile ducts are lined by a layer of cuboidal or columnar epithelium that has microvillous architecture on its luminal surface. The cells have a prominent Golgi apparatus and numerous vesicles, which probably participate in the exchange of substances among the cytoplasm, bile, and blood plasma through exocytosis and endocytosis. The interlobular bile ducts unite to form larger and larger ducts, first the septal ducts and then the lobar ducts, two hepatic ducts, and finally a common hepatic duct The common hepatic duct emerges from the porta hepatis after the union of the right and left hepatic ducts. It merges with the cystic duct emanating from the gallbladder to form the common bile duct. In adults, the common bile duct is quite large, ~7 cm in length and ~0.5 to 1.5 cm in diameter. In most individuals, the common bile duct and the pancreatic duct merge before forming a common antrum known as the ampulla of Vater. At the point of transit through the duodenal wall, this common channel is surrounded by a thickening of both the longitudinal and the circular layers of smooth muscle, the so-called sphincter of Oddi. This sphincter constricts the lumen of the bile duct and thus regulates the flow of bile UPTAKE, PROCESSING, AND SECRETION OF COMPOUNDS BY HEPATOCYTES The liver metabolizes an enormous variety of compounds that are brought to it by the portal and systemic circulations. These compounds include endogenous molecules (e.g., bile salts and bilirubin, which are key ingredients of bile) and exogenous molecules (e.g., drugs and toxins). The hepatocyte handles these molecules in four major steps: (1) the hepatocyte imports the compound from the blood across its basolateral (i.e., sinusoidal) membrane, (2) the hepatocyte transports the material within the cell, (3) the hepatocyte may chemically modify or degrade the compound intracellularly, and (4) the hepatocyte excretes the molecule or its product or products into the bile across the apical (i.e., canalicular) membrane The primary bile acids are cholic acid and chenodeoxycholic acid, both of which are synthesized by hepatocytes. Other “secondary” bile acids form in the intestinal tract as bacteria dehydroxylate the primary bile acids. Because the small intestine absorbs some bile acids and salts, they appear in the blood plasma, mainly bound to albumin, and are presented to the hepatocytes for re-uptake. This recycling of bile acids, an example of enterohepatic circulation Jaundice-Icterus Jaundice denotes a yellowish discoloration of body tissues, most notable in the skin and sclera of the eyes. The condition is caused by an accumulation of bilirubin in extracellular fluid, either in free form or after conjugation. Bilirubin is a yellow-green pigment that is the principal degradation product of heme, the iron-binding portion of hemoglobin. The metabolism of hemoglobin of senescent red cells accounts for 65% to 80% of total bilirubin production. Because of extraction and conjugation by the liver, the normal plasma concentration of bilirubin, which is mostly of the unconjugated variety, is ~0.5 mg/dL or lower. The skin or eyes may begin to appear jaundiced when the bilirubin level rises to 1.5 to 3 mg/dL. Jaundice-Icterus Jaundice occurs under several circumstances. Increased destruction of red blood cells or hemolysis may occur with rapid release of free, unconjugated bilirubin into the circulation. Unconjugated hyperbilirubinemia occurs commonly in neonates not only because of increased production of heme but also because of the immaturity of the pathways for glucuronidation in the liver. Obstruction of the bile ducts or damage to the liver may also result in jaundice. In this setting, conjugated bilirubin produced by the liver cannot be excreted in bile and consequently refluxes back into the systemic circulation. Jaundice-Icterus Under normal conditions, approximately half of the bilirubin reaching the intestinal lumen is metabolized by bacteria into the colorless urobilinogen. The intestinal mucosa reabsorbs ~20% of this soluble compound into the portal circulation. The liver then extracts most of the urobilinogen and re-excretes it into the gastrointestinal tract. The kidneys excrete a small fraction (~20% of daily urobilinogen production) into the urine. Jaundice-Icterus Oxidation of urobilinogen yields urobilin, which gives urine its yellow color. In the feces, metabolism of urobilinogen yields stercobilin, which contributes to the color of feces. In obstructive jaundice, no bilirubin reaches the intestine for conversion into urobilinogen, and therefore no urobilinogen appears in the blood for excretion by the kidney. Because of the lack of stercobilin and other bile pigments in obstructive jaundice, the stool becomes clay colored. Cholestasis The term cholestasis refers to the suppression of bile secretion. Cholestasis causes three major groups of negative effects: First, regurgitation of bile components (bile acids, bilirubin) into the systemic circulation gives rise to the symptoms of jaundice and pruritus (itching). Second, cholestasis damages hepatocytes, as evidenced by the release of liver enzymes (e.g., alkaline phosphatase) into the plasma. Third, because the bile acids do not arrive in the duodenum, lipid digestion and absorption may be impaired. Gallstones Most gallstones (~80%) consist mainly of cholesterol. Thus, cholelithiasis may be regarded as a disturbance of bile secretion and cholesterol elimination. When cholesterol and phospholipids are secreted together into the bile, they form unilamellar bilayered vesicles. These vesicles become incorporated into mixed micelles that form because of the amphiphilic properties of bile acids. Micellation allows cholesterol to remain in solution in its passage through the biliary tree. However, if the concentration of bile acids is insufficient to maintain all the cholesterol in the form of mixed micelles, the excess cholesterol is left behind as vesicles in the aqueous phase. These cholesterol-enriched vesicles are relatively unstable and are prone to aggregate and form large multilamellar vesicles, from which cholesterol crystals nucleate. Growth of crystals may result in the formation of gallstones BIOTRANSFORMATION IN LIVER The liver is responsible for the metabolism and detoxification of many endogenous and exogenous compounds. Some compounds (e.g., proteins and other ligands) taken up by hepatocytes are completely digested within lysosomes. Hepatocytes handle other compounds by biotransformation reactions that usually occur in two phases. Phase I reactions represent oxidation or reduction reactions in large part catalyzed by the P-450 cytochromes The metabolic products of phase I may be directly excreted, but more commonly, because of only a modest increment in solubility, further metabolism by phase II reactions is required BIOTRANSFORMATION IN LIVER In phase II, the hepatocyte conjugates the metabolites generated in phase I to produce more hydrophilic compounds, such as glucuronides, sulfates, and mercapturic acids. These phase II products are readily secreted into the blood or bile. Conjugation reactions are generally considered to be the critical step in detoxification. BIOTRANSFORMATION IN LIVER The liver and intestine express a nuclear receptor called the steroid and xenobiotic receptor (SXR). SXR serves as a master regulator of xenobiotic metabolism. Enzymes upregulated by SXR include the phase I drug-metabolizing enzymes of the P-450 family, such as CYP3A, which metabolizes more than 50% of all drugs in humans. SXR also activates the phase II enzyme glutathione-S-transferase, which is critical for catalyzing conjugation of many substrates to glutathione. SXR also upregulates the multidrug resistance transporter MDR1 LIVER & GLUCOSE METABOLISM The liver is one of the key organs that maintain blood glucose concentrations within a narrow range, in a dynamic process involving endogenous glucose production and glucose utilization. The fasting blood [glucose] is normally 4 to 5 mM. Between meals, when levels of insulin are relatively low and levels of glucagon are high, the liver serves as a source of plasma glucose, both by synthesizing glucose and by generating it from the breakdown of glycogen. The de novo synthesis of glucose, or gluconeogenesis, is one of the liver’s most important functions It is essential for maintaining a normal plasma concentration of glucose, which is the primary energy source for most tissues Glucose is synthesized in the lumen of the ER, principally from amino acids and lactate. Dietary fructose and galactose are also largely converted to glucose. Glucose exits the ER by facilitated diffusion (mediated by GLUT7) and then passes into the blood through another facilitated diffusion mechanism (GLUT2) The second way in which the liver delivers glucose to blood plasma is by glycogenolysis. Stored glycogen may account for as much as 7% to 10% of the total weight of the liver. Glycogenolysis in the liver yields glucose as its major product After a meal, when levels of insulin are relatively high, the liver does just the opposite: it acts as a sink for glucose by taking it up from the portal blood and either breaking it down to pyruvate or using it to synthesize glycogen The liver also consumes glucose by using it for glycogen synthesis. Carbohydrate that is not stored as glycogen or oxidized is metabolized to fat The liver synthesizes a variety of important plasma proteins One of the major functions of the liver is to produce a wide array of proteins for export to the blood plasma. These products include major plasma proteins that are important for maintaining the colloid osmotic pressure of plasma. Other products include factors involved in hemostasis (blood clotting) and fibrinolysis (breakdown of blood clots), carriage proteins that bind and transport hormones and other substances in the blood, prohormones, and lipoproteins The liver obtains dietary triglycerides and cholesterol Enterocytes in the small intestine process fatty acids consumed as dietary triglycerides and secrete them into the lymph primarily in the form of extremely large proteolipid aggregates called chylomicrons. These chylomicrons—made up of triglycerides (80% to 90%), phospholipids, cholesterol, and several apo-proteins—pass from the lymph to the blood through the thoracic duct. Lipoprotein lipase (LPL) on the walls of the capillary endothelium in adipose tissue and muscle then partially digests the triglycerides in these chylomicrons. The results of this digestion are glycerol, fatty acids, and smaller or “remnant” chylomicrons, which are triglyceride depleted and thus enriched in cholesterol The glycerol and fatty acids generated by LPL enter adipocytes and muscle cells. The cholesterol-rich remnant chylomicrons, conversely, remain in the blood and make their way to the liver, where they enter hepatocytes by a process that is saturable, specific, and of high affinity. Although low-density lipoprotein (LDL) receptors can recognize chylomicron remnants, another member of the LDL receptor gene family, the LDL-related receptor, is of greater importance. The chylomicron remnant binds to this receptor on the basolateral membrane, enters the hepatocyte through receptor-mediated endocytosis and is degraded in lysosomes. Thus, chylomicrons transport dietary triglycerides to adipose tissue and muscle, whereas their remnants transport dietary triglycerides and cholesterol to hepatocytes. To extract energy from the neutral fats derived from the remnant chylomicrons, hepatocytes must first split the triglycerides into glycerol and fatty acids. The fatty acids thus derived from remnant chylomicrons, as well as those that enter the hepatocyte directly, are metabolized by -oxidation into two-carbon acetyl intermediates that then form acetyl CoA. Acetyl CoA can enter the citric acid cycle, where it is oxidized to produce large amounts of energy. The acetyl CoA that is not used by the liver is converted by the condensation of two molecules of acetyl CoA to yield acetoacetic acid. The liver is the only organ that produces acetoacetate for metabolism by muscle, brain, and kidney, but it does not use this substrate for its own energy needs. In fasting or in poorly controlled diabetes, in which the supply of acetyl CoA is in excess, the acetyl CoA is diverted to produce acetoacetate, which, in turn, can yield β-hydroxybutyrate and acetone. Together, acetoacetate, β-hydroxybutyrate, and acetone are referred to as ketone bodies. Another fate of fatty acids in the liver is that they can be re- esterified to glycerol, with the formation of triglycerides that can either be stored or exported as very-low-density lipoproteins (VLDLs) and released into the circulation for use by peripheral tissues LIVER & CHOLESTEROL The liver is the major organ for controlling cholesterol metabolism. The liver obtains cholesterol from three major sources. First, the intestine packages dietary cholesterol as chylomicrons, which travel through the lymph to blood vessels in adipocytes and muscle, where LPL hydrolyzes triglycerides to fatty acids and glycerol. The resultant cholesterol-enriched remnant chylomicrons are then delivered as cholesterol to the liver. Second, the liver synthesizes cholesterol de novo. Third, the liver takes up cholesterol in the guise of LDLs. However, the liver exports cholesterol in two major ways: (1) the liver uses cholesterol to synthesize bile acids and also includes cholesterol and cholesteryl esters in the bile (2) the liver also exports cholesterol to the blood in the form of VLDLs. The other major player in cholesterol metabolism is HDL, which is composed of cholesterol, phospholipids, triglycerides, and apoproteins. The two major apoproteins of HDLs, A-I and A-II, are made by the intestines as part of chylomicrons, as well as by the liver LIVER & LIPID SOLUBLE VITAMINS Vitamin A (retinol and its derivatives)—like dietary vitamin D, as well as vitamins E and K—is absorbed from the intestine and is then transported in newly synthesized chylomicrons or VLDLs. In the hepatocyte, retinyl esters may be hydrolyzed to release free retinol, which can then be transported into the sinusoids bound to retinol-binding protein (RBP) and prealbumin. Alternatively, retinyl esters may be stored in the hepatocyte or transported as RBP- bound retinol to stellate (Ito) cells, the storage site of more than 80% of hepatic vitamin A under normal conditions. Liver disease resulting in cholestasis may lead to a secondary vitamin A deficiency by interfering with absorption in the intestine (lack of the bile needed for digestion/absorption of vitamin A) or by impairing delivery to target tissues because of reduced hepatic synthesis of RBP Skin cells—under the influence of ultraviolet light—synthesize vitamin D3. Dietary vitamin D can come from either animal sources (D3) or plant sources (D2). In either case, the first step in activation of vitamin D is the 25-hydroxylation of vitamin D, catalyzed by a hepatic cytochrome P-450 enzyme. This hydroxylation is followed by 1-hydroxylation in the kidney to yield a product (1,25-dihydroxyvitamin D) with full biological activity. Vitamin E is absorbed from the intestine primarily in the form of - and ©- tocopherol. It is incorporated into chylomicrons and VLDLs with other products of dietary lipid digestion. Vitamin K is a fat-soluble vitamin produced by intestinal bacteria. This vitamin is essential for certain coagulation factors II, VII, IX, and X as well as anticoagulants protein C and protein S. Intestinal absorption and handling of vitamin K—which is present in two forms, K1 and K2—are similar to those of the other fat-soluble vitamins: A, D, and E. Common causes of vitamin K deficiency, which can lead to a serious bleeding disorder, include extrahepatic or intrahepatic cholestasis, fat malabsorption, biliary fistulas, and dietary deficiency, particularly in association with antibiotic therapy The liver stores copper and iron The trace element copper is essential for the function of cuproenzymes such as cytochrome c oxidase and superoxide dismutase. Approximately half the copper in the diet (recommended dietary allowance, 1.5 to 3 mg/day) is absorbed in the jejunum and reaches the liver in the portal blood, mostly bound to albumin. A small fraction is also bound to amino acids, especially histidine. More than 80% of the copper absorbed by the jejunum each day is excreted in bile Wilson Disease Wilson disease is inherited as an autosomal recessive illness caused by a mutation in ATP7B, the pump responsible for copper accumulation in the trans-Golgi network. The impaired biliary excretion of copper causes a buildup of copper in cells, which produces toxic effects in the liver, brain, kidney, cornea, and other tissues. The disease is rare, but it must be considered in the differential diagnosis of anyone younger than 30 years with evidence of significant liver disease. Patients most often have neuropsychiatric complications, including ataxia, tremors, increased salivation, and behavioral changes. Slitlamp examination of the cornea reveals the diagnostic Kayser-Fleischer rings at the limbus of the cornea. Dietary iron is absorbed by the duodenal mucosa and is then transported through the blood bound to transferrin, a protein synthesized in the liver. The liver also takes up, secretes, and stores iron. Entry of iron into hepatocytes is mediated through specific cell surface transferrin receptors. Within the cell, a small pool of soluble iron is maintained for intracellular enzymatic reactions, primarily for those involved in electron transport. However, iron is also toxic to the cell. Hence, most intracellular iron is complexed to ferritin. THANK YOU…