Developmental Biology Lesson 27 - Chapter 22 - The Endoderm
Document Details
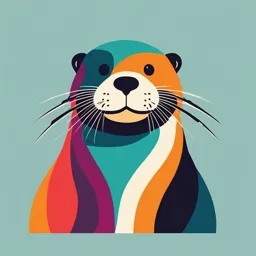
Uploaded by LustrousBongos5217
Augustana University
Tags
Summary
This document provides an overview of lesson 27, specifically focusing on Chapter 22. It details the Endoderm, including its two primary sources, primitive and definitive Endoderm and discusses the Sox17 transcription factor, differential signals, and anterior-posterior axis specification. The document presents this information with various images and figures to illustrate the concepts presented.
Full Transcript
BIOL 346 Developmental Biology Lesson 27 Chapter 22: The Endoderm: Tubes and Organs for Digestion and Respiration Emergence of the Endoderm Two Sources of Endoderm: Primitive Endoderm: First cells to delaminate from the epiblas...
BIOL 346 Developmental Biology Lesson 27 Chapter 22: The Endoderm: Tubes and Organs for Digestion and Respiration Emergence of the Endoderm Two Sources of Endoderm: Primitive Endoderm: First cells to delaminate from the epiblast, forming the yolk sac and aiding in gastrulation. Definitive Endoderm: Enters through the primitive streak during gastrulation, intercalating into the primitive endoderm layer. Sox17 Transcription factor: Marks endoderm cells as they exit the primitive streak. Essential for definitive endoderm formation; mutants lacking Sox17 fail to develop this layer. Differential signals: High Nodal induces Sox17 (endoderm fate); BMPs and FGFs specify mesoderm by opposing Nodal. Anterior-Posterior Axis Specification: Low Wnt/FGF/BMP (Anterior): Forms lung and thyroid precursors (anterior foregut). Moderate Wnt/FGF/BMP (Central): Forms liver and pancreas precursors (posterior foregut). High Wnt/FGF/BMP (Posterior): Forms intestinal progenitors (midgut-hindgut). Fig 22.1: Signals mediating endoderm progenitor fates Fig. 22.2: Endodermal folding (yellow) during early human development Formation of Gut Tube: Gut cells start as a flat sheet beneath or around the embryo (chick/human vs. mouse). Tube formation starts at two sites—anterior intestinal portal (AIP) and caudal intestinal portal (CIP)—which fuse to form the midgut. Endoderm-Ectoderm Junctions: Unique junctions at oral (anterior) plate and anorectal (posterior) junction where endoderm meets ectoderm. By ~22 days, the oral plate breaks, forming the oral opening, lined by ectoderm. Pituitary Gland Dual Origin: Ectoderm from oral plate forms Rathke’s pouch (anterior pituitary). Diencephalon neural tissue forms the infundibulum (posterior pituitary). Sox17 as Boundary Marker: Sox17 highlights ectoderm-endoderm boundary in the mouth. Teeth and major salivary glands originate from ectoderm, while posterior taste buds and some posterior glands are endodermal. Fig. 22.6: Regional specification of the gut endoderm and splanchnic mesoderm through reciprocal interactions Wnt signaling Role: Wnt signals from posterior mesoderm (guided by RA and FGF gradients) activate transcription factors (Cdx1, Cdx2) and Indian hedgehog; high (Cdx) levels specify the large intestine, lower levels specify the small intestine. Cdx2 Function: (Cdx2) suppresses genes like (Hhex), blocking stomach, liver, and pancreas formation in the posterior, while Wnt inhibition in the anterior allows these organs to form. Barx and Want Inhibition: In the stomach, (Barx1) activates Wnt antagonists (sFRP1, sFRP2) to block Wnt signaling, allowing stomach development. Endoderm-Mesoderm Interaction: (Shh) from endoderm induces (Hox) genes in mesoderm, essential for precise regional gut specification. Fig. 22.7: The muscular layers of the gut tube form in response to signals originating in the endoderm Sequential Muscle Layer Formation: Inner circumferential muscle layer forms first, followed by the outer longitudinal layer. Signal Regulation of Muscle Pattern: Shh induces BMP, restricting initial muscle formation to the inner layer. BMP antagonists from the inner layer and enteric neurons enable outer layer muscle formation. Mechanical Forces and Muscle Alignment Continuous tension aligns inner layer cells circumferentially. Cyclic contractions align outer layer cells longitudinally. Villi and ISCs: Mesoderm compression shapes villi; (Shh) and (BMP) signaling limits intestinal stem cell (ISC) formation to villi bases. Fig. 22.8: Pancreatic development in humans _Pancreas Development: Starts with 2 pancreatic buds (dorsal and ventral) Ventral pancreas migrates and they fuse to form one pancreas In humans, most individuals just have one remaining duct into duodenum Fig. 22.9: Positive and negative signaling in formation of the mouse hepatic (liver) endoderm Inhibitory Signals: Ectoderm and notochord inhibit liver-specific gene expression in the endoderm. Positive Signals for Liver Development: Cardiogenic mesoderm releases FGFs, and adjacent mesenchyme secretes BMPs to activate liver-specific genes. Pancreas Inhibition: FGFs and BMPs promote liver development while simultaneously suppressing pancreatic gene expression in the same tissue. Fig. 22.10: Induction of Pdx1 gene expression in the gut epithelium Reciprocal induction during pancreatic development _Blood vessels induce Pdx1 expression and pancreatic buds off of endodermal tube (gut tube) Blood vessels are necessary and sufficient for Pdx1 expression and pancreatic development from endodermal tube. Islets of Langerhans include cells that secrete insulin (Beta cells) Islets secrete VEGF (promotes angiogenesis) to attract blood vessels to surround islet. Fig. 22.11: Pancreatic and liver cell lineages Pancreatic vs Liver Lineage: All pancreatic cells express Pdx1, distinguishing them from liver- forming cells. Endocrine and Exocrine Progenitors: Ngn3-expressing cells become endocrine progenitors, giving rise to hormone-producing cells. Ptf1a-expressing cells become exocrine progenitors, forming ducts and acinar cells that secrete digestive enzymes. Endocrine Lineage Differentiation: Endocrine progenitors divide into two lineages: one forms β and δ cells; the other forms α and PP cells. Fig. 22.12: Production of functional insulin-secreting human β cells from adult cells Goal of Cell Replacement Therapy: Developmental biology aims to replace damaged cells with new functional ones, such as insulin- secreting pancreatic β cells. __Induced Pluripotent Stem Cells (iPSCs): Adult skin cells can be reprogrammed into iPSCs, resembling embryonic stem cells, by activating specific transcription factors. Differentiation into β Cells: By mimicking embryonic conditions with specific paracrine factors, iPSCs can sequentially differentiate into pancreatic β cells. Functional Application: These lab-generated β cells successfully regulated glucose and cured diabetes in mouse models. Most human clinical trials are currently in Phase 1 or 2, focusing primarily on safety and initial efficacy data in a small number of patients. Fig. 22.14: Partitioning of the foregut into the esophagus and the respiratory diverticulum during the third and fourth weeks of human gestation Respiratory Tube Branches off of foregut (anterior digestive tube) Forms trachea and lungs Mesenchymal Signals: Wnt from mesenchyme determines whether esophagus or respiratory tube are specified Determine whether trachea or lung will form (to continue branching or not) Fig. 22.15: Morphogenesis of branches in the mouse bronchial epithelium Branching continues to form lung Branching pattern is not random: Highly stereotypical, can map lineage of each alveolus 3 patterns of branching: Domain branching: Like teeth along jaw Planar bifurcation: Split at the end along same plane Orthogonal birfucation: Split at end with 90-degree rotation Fig. 22.16: Smooth muscle may force branch bifurcation of the mouse lung Forces in Lung Branching Different Developmental Approaches: Mammals and birds use distinct methods to form lung branches. Role of Smooth Muscle in Mammals: In mammals, smooth muscle differentiation at the branch tip mesenchyme initiates cleft formation, connecting with smooth muscle around the proximal branch. __Branch Bifurication__________________: Smooth muscle contraction at the branch tip promotes bifurcation; disruption of this differentiation prevents terminal branching. Visual Evidence: Smooth muscle differentiation (αSMA) is observed at branch clefts, aligning with epithelial markers (E-cadherin) during bifurcation. Fig. 22.17: The mouse and avian airways form branches via different mechanisms Both mice and avian lungs have branched airways Mice rely on smooth muscle contractions to create bifurcations of lung branch buds Birds rely on apical constrictions to induce new branch points Both require FGF signaling from mesenchyme to induce branching