Lecture 18: From Big Bang to Black Holes PDF
Document Details
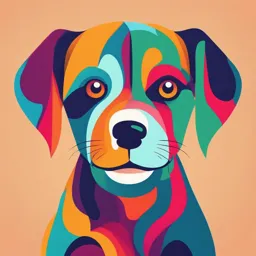
Uploaded by TimeHonoredTransformation
Dr. Dario Carbone
Tags
Summary
This lecture covers solar activity, its causes, effects on humans, and variability. It also introduces basic properties of stars, including luminosity, temperature and mass measurements, and the Hertzsprung-Russell diagram.
Full Transcript
From the Big Bang to Black Holes Dr. Dario Carbone The Sun–Earth Connection Our goals for learning: – What causes solar activity? – How does solar activity affect humans? – How does solar activity vary with time? What causes solar a...
From the Big Bang to Black Holes Dr. Dario Carbone The Sun–Earth Connection Our goals for learning: – What causes solar activity? – How does solar activity affect humans? – How does solar activity vary with time? What causes solar activity? © 2014 Pearson Education, Inc. Solar activity is like "weather". Sunspots Solar flares Solar prominences All these phenomena are related to magnetic fields. Sunspots They are cooler than other parts of the Sun's surface (4000 K) They are regions with strong magnetic fields © 2014 Pearson Education, Inc. Zeeman effect We can measure magnetic fields in sunspots by observing the splitting of spectral lines. © 2014 Pearson Education, Inc. Strong magnetic fields Loops of bright gas often connect sunspot pairs. © 2014 Pearson Education, Inc. Strong magnetic fields Magnetic activity causes solar flares that send bursts of X rays and charged particles into space. © 2014 Pearson Education, Inc. How does solar activity affect humans? © 2014 Pearson Education, Inc. Coronal mass ejections Coronal mass ejections send bursts of energetic charged particles out through the solar system. © 2014 Pearson Education, Inc. Coronal mass ejections Charged particles streaming from the Sun can disrupt electrical power grids and can disable communications satellites. © 2014 Pearson Education, Inc. How does solar activity vary with time? 11 years solar cycle © 2014 Pearson Education, Inc. Solar Cycle The number of sunspots rises and falls in an 11-year cycle. © 2014 Pearson Education, Inc. What have we learned? What causes solar activity? – Stretching and twisting of magnetic field lines near the Sun's surface cause solar activity. How does solar activity affect humans? – Bursts of charged particles from the Sun can disrupt radio communication and electrical power generation and damage satellites. How does solar activity vary with time? – Activity rises and falls with an 11-year period. https://shorturl.at/IjjT3 Surveying the Stars © 2014 Pearson Education, Inc. Properties of Stars Our goals for learning: – How do we measure stellar luminosities? – How do we measure stellar temperatures? – How do we measure stellar masses? How do we measure stellar luminosities? The brightness of a star depends on both distance and luminosity © 2014 Pearson Education, Inc. How do we measure stellar luminosities? Luminosity: Amount of power a star radiates (energy per second = watts) Apparent brightness: Amount of starlight that reaches Earth (energy per second per square meter) © 2014 Pearson Education, Inc. Thought Question Alpha Centauri and the Sun have about the same luminosity. Which one appears brighter? A. Alpha Centauri B. The Sun Thought Question Alpha Centauri and the Sun have about the same luminosity. Which one appears brighter? A. Alpha Centauri B. The Sun How do we measure stellar luminosities? The amount of luminosity passing through each sphere is the same. 2 Area of sphere = 4πR Divide luminosity by area to get brightness. © 2014 Pearson Education, Inc. How do we measure stellar luminosities? The relationship between apparent brightness and luminosity depends on distance: Luminosity Brightness = 4π (distance)2 We can determine a star's luminosity if we can measure its distance and apparent brightness: Luminosity = 4π (distance)2 × (brightness) © 2014 Pearson Education, Inc. Thought Question How would the apparent brightness of Alpha Centauri change if it were three times farther away? A. It would be only 1/3 as bright B. It would be only 1/6 as bright. C. It would be only 1/9 as bright. D. It would be three times brighter. Thought Question How would the apparent brightness of Alpha Centauri change if it were three times farther away? A. It would be only 1/3 as bright B. It would be only 1/6 as bright. C. It would be only 1/9 as bright. D. It would be three times brighter. So how far away are these stars? © 2014 Pearson Education, Inc. Parallax Parallax is the apparent shift in position of a nearby object against a background of more distant objects. © 2014 Pearson Education, Inc. © 2014 Pearson Education, Inc. Parallax and Distance p = parallax angle d (in parsecs) = 1 p (in arcseconds) d (in light-years) = 3.26 × 1 p (in arcseconds) Most luminous stars: 106 LSun Least luminous stars: 10–4LSun LSun is luminosity of Sun © 2014 Pearson Education, Inc. The Magnitude Scale m = apparent magnitude, M = absolute magnitude Apparent brightness of star 1 = (100 ) 1/5 m1 −m2 Apparent brightness of star 2 Luminosity of star 1 = (100 ) 1/5 M1 −M2 Luminosity of star 2 How do we measure stellar temperatures? © 2014 Pearson Education, Inc. How do we measure stellar temperatures? Every object emits thermal radiation with a spectrum that depends on its temperature. © 2014 Pearson Education, Inc. Properties of Thermal Radiation 1. Hotter objects emit more light per unit area at all frequencies. 2. Hotter objects emit photons with a higher average energy. Hottest stars: 50,000 K Coolest stars: 3000 K Sun's surface is 5800 K © 2014 Pearson Education, Inc. How do we measure stellar temperatures? Absorption lines in star's spectrum tell us its temperature © 2014 Pearson Education, Inc. How do we measure stellar temperatures? Lines in a star's spectrum correspond to a spectral type that reveals its temperature (Hottest) O B A F G K M (Coolest) © 2014 Pearson Education, Inc. Thought Question Which kind of star is hottest? A. M star B. F star C. A star D. K star Thought Question Which kind of star is hottest? A. M star B. F star C. A star D. K star How do we measure stellar masses? © 2014 Pearson Education, Inc. How do we measure stellar masses? The orbit of a binary star system depends on strength of gravity. © 2014 Pearson Education, Inc. How do we measure stellar masses? We measure mass using gravity. Direct mass measurements are possible only for stars in binary star systems. 4π2 p 2 = a 3 G (M1 + M2) p = period a = average separation © 2014 Pearson Education, Inc. Need two out of three observables to measure mass: 1. Orbital period (p) 2. Orbital separation (a or r = radius) 3. Orbital velocity (v) For circular orbits, v = 2πr/p. v r M © 2014 Pearson Education, Inc. Most massive stars: 100MSun Least massive stars: 0.08MSun MSun is the mass of the Sun © 2014 Pearson Education, Inc. What have we learned? How do we measure stellar luminosities? – If we measure a star's apparent brightness and distance, we can compute its luminosity with the inverse square law for light. – Parallax tells us distances to the nearest stars. How do we measure stellar temperatures? – A star's color and spectral type both reflect its temperature. © 2014 Pearson Education, Inc. What have we learned? How do we measure stellar masses? – Newton's version of Kepler's third law tells us the total mass of a binary system, if we can measure the orbital period (p) and average orbital separation of the system (a). © 2014 Pearson Education, Inc. Patterns Among Stars Our goals for learning: – What is a Hertzsprung-Russell diagram? – What is the significance of the main sequence? – What are giants, supergiants, and white dwarfs? – Why do the properties of some stars vary? What is a Hertzsprung-Russell diagram? © 2014 Pearson Education, Inc. What is a Hertzsprung-Russell diagram? Luminosity A H-R diagram plots the luminosity and temperature of stars. © 2014 Pearson Education, Inc. Temperature What is a Hertzsprung-Russell diagram? Most stars fall somewhere on the main sequence of the H-R diagram. © 2014 Pearson Education, Inc. What is a Hertzsprung-Russell diagram? Stars with lower T and higher L than those on the main sequence must have larger radii. These stars are called giants and supergiants. © 2014 Pearson Education, Inc. What is a Hertzsprung-Russell diagram? Stars with higher T and lower L than those on the main sequence must have smaller radii. These stars are called white dwarfs. © 2014 Pearson Education, Inc. Stellar Luminosity Classes A star's full classification includes spectral type (line identities) and luminosity class (line shapes, related to the size of the star): I - supergiant II - bright giant III - giant IV - subgiant V - main sequence Examples: Sun - G2 V Sirius - A1 V Proxima Centauri - M5.5 V Betelgeuse - M2 I © 2014 Pearson Education, Inc. What is a Hertzsprung-Russell diagram? H-R diagram depicts: Luminosity - Temperature - Color - Spectral type - Luminosity - Radius Temperature © 2014 Pearson Education, Inc. Which star is the hottest? Luminosity © 2014 Pearson Education, Inc. Temperature Which star is the most luminous? Luminosity © 2014 Pearson Education, Inc. Temperature Which star is a main-sequence star? Luminosity © 2014 Pearson Education, Inc. Temperature Which star has the largest radius? Luminosity © 2014 Pearson Education, Inc. Temperature Stellar Properties Review Luminosity: from brightness and distance 10–4LSun–106LSun Temperature: from color and spectral type 3000 K–50,000 K Mass: from period (p) and average separation (a) of binary star orbit 0.08MSun – 100MSun Mass and Lifetime Sun's life expectancy: 10 billion years Mass and Lifetime Until core hydrogen (10% of total) Sun's life expectancy: 10 billion years is used up Mass and Lifetime Until core hydrogen (10% of total) Sun's life expectancy: 10 billion years is used up Life expectancy of 10MSun star: 10 times as much fuel, uses it 104 times as fast 10 million years ~ 10 billion years × 10/104 Mass and Lifetime Until core hydrogen (10% of total) Sun's life expectancy: 10 billion years is used up Life expectancy of 10MSun star: 10 times as much fuel, uses it 104 times as fast 10 million years ~ 10 billion years × 10/104 Life expectancy of 0.1MSun star: 0.1 times as much fuel, uses it 0.01 times as fast 100 billion years ~ 10 billion years × 0.1/0.01 Main-Sequence Star Summary High-Mass Star: High luminosity Short-lived Larger radius Blue Low-Mass Star: Low luminosity Long-lived Small radius Red © 2014 Pearson Education, Inc. What are giants, supergiants, and white dwarfs? © 2014 Pearson Education, Inc. Sizes of Giants and Supergiants © 2014 Pearson Education, Inc. Off the Main Sequence Stellar properties depend on both mass and age: Those that have finished fusing H to He in their cores are no longer on the main sequence. All stars become larger and redder after exhausting their core hydrogen: giants and supergiants. Most stars end up small and white after fusion has ceased: white dwarfs. © 2014 Pearson Education, Inc. Which star is most like our Sun? Luminosit Temperature © 2014 Pearson Education, Inc. Which of these stars will have changed the least 10 billion years from now? Luminosit Temperature © 2014 Pearson Education, Inc. Which of these stars can be no more than 10 million years old? Luminosit Temperature © 2014 Pearson Education, Inc. Variable Stars Any star that varies significantly in brightness with time is called a variable star. Some stars vary in brightness because they cannot achieve proper balance between power welling up from the core and power radiated from the surface. Such a star alternately expands and contracts, varying in brightness as it tries to find a balance. Pulsating Variable Stars The light curve of this pulsating variable star shows that its brightness alternately rises and falls over a 50-day period. © 2014 Pearson Education, Inc. Cepheid Variable Stars Most pulsating variable stars inhabit an instability strip on the H-R diagram. The most luminous ones are known as Cepheid variables. © 2014 Pearson Education, Inc. What have we learned? What is a Hertzsprung-Russell diagram? – An H-R diagram plots stellar luminosity of stars versus surface temperature (or color or spectral type). What is the significance of the main sequence? – Normal stars that fuse H to He in their cores fall on the main sequence of an H-R diagram. – A star's mass determines its position along the main sequence (high-mass: luminous and blue; low-mass: faint and red). What have we learned? What are giants, supergiants, and white dwarfs? – All stars become larger and redder after core hydrogen burning is exhausted: giants and supergiants. – Most stars end up as tiny white dwarfs after fusion has ceased. Why do the properties of some stars vary? – Some stars fail to achieve balance between power generated in the core and power radiated from the surface. Star Clusters Our goals for learning: – What are the two types of star clusters? – How do we measure the age of a star cluster? What are the two types of star clusters? © 2014 Pearson Education, Inc. Open cluster: A few thousand loosely packed stars © 2014 Pearson Education, Inc. Globular cluster: Up to a million or more stars in a dense ball bound together by gravity © 2014 Pearson Education, Inc. How do we measure the age of a star cluster? © 2014 Pearson Education, Inc. How do we measure the age of a star cluster? The Pleiades cluster now has no stars with life expectancy less than around 100 million years. © 2014 Pearson Education, Inc. How do we measure the age of a star cluster? The main-sequence turnoff point of a cluster tells us its age. © 2014 Pearson Education, Inc. How do we measure the age of a star cluster? Detailed modeling of the oldest globular clusters reveals that they are about 13 billion years old. © 2014 Pearson Education, Inc. What have we learned? What are the two types of star clusters? – Open clusters are loosely packed and contain up to a few thousand stars. – Globular clusters are densely packed and contain hundreds of thousands of stars. How do we measure the age of a star cluster? – A star cluster's age roughly equals the life expectancy of its most massive stars still on the main sequence. From the Big Bang to Black Holes Dr. Dario Carbone The Formation of Other Solar Systems Our goals for learning: – Can we explain the surprising orbits of many extrasolar planets? – Do we need to modify our theory of solar system formation? Can we explain the surprising orbits of many extrasolar planets? © 2014 Pearson Education, Inc. Revisiting the Nebular Theory The nebular theory predicts that massive Jupiter- like planets should not form inside the frost line (at NO Chemical energy ~ 10,000 years Luminosity © 2014 Pearson Education, Inc. Is it contracting? © 2014 Pearson Education, Inc. Is it contracting? Gravitational potential energy ~ 25 million years Luminosity © 2014 Pearson Education, Inc. Is it contracting? —> NO Gravitational potential energy ~ 25 million years Luminosity © 2014 Pearson Education, Inc. 2 It can be powered by nuclear energy (E = mc ) Nuclear potential energy (core) ~ 10 billion years Luminosity © 2014 Pearson Education, Inc. Weight of upper layers compresses lower layers. © 2014 Pearson Education, Inc. Gravitational Equilibrium Energy supplied by fusion maintains the pressure that balances the inward crush of gravity. © 2014 Pearson Education, Inc. Energy Balance The rate at which energy radiates from the surface of the Sun must be the same as the rate at which it is released by fusion in the core. © 2014 Pearson Education, Inc. Gravitational Contraction Provided the energy that heated the core as the Sun was forming. Gravitational contraction stopped when nuclear fusion began. © 2014 Pearson Education, Inc. Our Sun Radius: 6.9 × 108 m (109 times Earth) Mass: 2 × 1030 kg (300,000 Earths) Luminosity: 3.8 × 1026 W © 2014 Pearson Education, Inc. What have we learned? Why does the Sun shine? – Chemical and gravitational energy sources could not explain how the Sun could sustain its luminosity for more than about 25 million years. – The Sun shines because gravitational equilibrium keeps its core hot and dense enough to release energy through nuclear fusion. Nuclear Fusion in the Sun Our goals for learning: – How does nuclear fusion occur in the Sun? – How does the energy from fusion get out of the Sun? – How do we know what is happening inside the Sun? How does nuclear fusion occur in the Sun? © 2014 Pearson Education, Inc. Types of nuclear reactions Big nucleus splits into smaller Small nuclei stick together to pieces. make a bigger one. Example: nuclear power plants Example: the Sun, stars © 2014 Pearson Education, Inc. Nuclear fusion needs extreme conditions High temperatures and densities enable nuclear fusion to happen in the core. © 2014 Pearson Education, Inc. Nuclear fusion in the Sun The Sun releases energy by fusing four hydrogen nuclei into one helium nucleus. The proton–proton chain is how hydrogen fuses into helium in Sun. © 2014 Pearson Education, Inc. Thought Question What would happen inside the Sun if a slight rise in core temperature led to a rapid rise in fusion energy? A. The core would expand and heat up slightly. B. The core would expand and cool. C. The Sun would blow up like a hydrogen bomb. Thought Question What would happen inside the Sun if a slight rise in core temperature led to a rapid rise in fusion energy? A. The core would expand and heat up slightly. B. The core would expand and cool. C. The Sun would blow up like a hydrogen bomb. The solar thermostat keeps burning rate steady. © 2014 Pearson Education, Inc. Solar Thermostat Decline in core temperature Rise in core temperature causes fusion rate to drop, causes fusion rate to rise, so so core contracts and heats core expands and cools up. down. © 2014 Pearson Education, Inc. How does the energy from fusion get out of the Sun? Energy gradually leaks out of radiation zone in form of randomly bouncing photons. © 2014 Pearson Education, Inc. Convection Convection (rising hot gas) takes energy to surface. © 2014 Pearson Education, Inc. Convection Bright blobs on photosphere show where hot gas is reaching the surface © 2014 Pearson Education, Inc. How we know what is happening inside the Sun? We learn about the inside of the Sun by … making mathematical models observing solar vibrations observing solar neutrinos Solar neutrinos Neutrinos created during fusion fly directly through the Sun. Observations of these solar neutrinos can tell us what's happening in core. © 2014 Pearson Education, Inc. What have we learned? How does nuclear fusion occur in the Sun? – The core's extreme temperature and density are just right for nuclear fusion of hydrogen to helium through the proton–proton chain. – Gravitational equilibrium acts as a thermostat to regulate the core temperature because fusion rate is very sensitive to temperature. What have we learned? How does the energy from fusion get out of the Sun? – Randomly bouncing photons carry energy through the radiation zone. – Rising of hot plasma carries energy through the convection zone to photosphere. How do we know what is happening inside the Sun? – Mathematical models agree with observations of solar vibrations and solar neutrinos. The Sun–Earth Connection Our goals for learning: – What causes solar activity? – How does solar activity affect humans? – How does solar activity vary with time? What causes solar activity? © 2014 Pearson Education, Inc. Solar activity is like "weather". Sunspots Solar flares Solar prominences All these phenomena are related to magnetic fields. Sunspots They are cooler than other parts of the Sun's surface (4000 K) They are regions with strong magnetic fields © 2014 Pearson Education, Inc. Zeeman effect We can measure magnetic fields in sunspots by observing the splitting of spectral lines. © 2014 Pearson Education, Inc. Strong magnetic fields Loops of bright gas often connect sunspot pairs. © 2014 Pearson Education, Inc. Strong magnetic fields Magnetic activity causes solar flares that send bursts of X rays and charged particles into space. © 2014 Pearson Education, Inc. How does solar activity affect humans? © 2014 Pearson Education, Inc. Coronal mass ejections Coronal mass ejections send bursts of energetic charged particles out through the solar system. © 2014 Pearson Education, Inc. Coronal mass ejections Charged particles streaming from the Sun can disrupt electrical power grids and can disable communications satellites. © 2014 Pearson Education, Inc. How does solar activity vary with time? 11 years solar cycle © 2014 Pearson Education, Inc. Solar Cycle The number of sunspots rises and falls in an 11-year cycle. © 2014 Pearson Education, Inc. What have we learned? What causes solar activity? – Stretching and twisting of magnetic field lines near the Sun's surface cause solar activity. How does solar activity affect humans? – Bursts of charged particles from the Sun can disrupt radio communication and electrical power generation and damage satellites. How does solar activity vary with time? – Activity rises and falls with an 11-year period. From the Big Bang to Black Holes Dr. Dario Carbone Detecting Planets Around Other Stars Our goals for learning: – Why is it so challenging to learn about extrasolar planets? – How can a star's motion reveal the presence of planets? – How can changes in a star's brightness reveal the presence of planets? Why is it so challenging to learn about extrasolar planets? © 2014 Pearson Education, Inc. Brightness and Distance A Sun-like star is about a billion times brighter than the light reflected from its planets. Planets are close to their stars, relative to the distance from us to the star. – This is like being in San Francisco and trying to see a pinhead 15 meters from a grapefruit in Washington, D.C. How Did We Learn That Other Stars Are Suns? Ancient observers didn't think stars were like the Sun because Sun is so much brighter. Christian Huygens (1629–1695) used holes drilled in a brass plate to estimate the angular sizes of stars. His results showed that, if stars were like Sun, they must be at great distances, consistent with the lack of observed parallax. How can a star's motion reveal the presence of planets? © 2014 Pearson Education, Inc. Planet Detection Direct: pictures or spectra of the planets themselves Indirect: measurements of stellar properties revealing the effects of orbiting planets Gravitational Tugs The Sun and Jupiter orbit around their common center of mass. The Sun therefore wobbles around that center of mass with same period as Jupiter. © 2014 Pearson Education, Inc. Gravitational Tugs The Sun's motion around the solar system's center of mass depends on tugs from all the planets. Astronomers around other stars that measured this motion could determine the masses and orbits of all the planets. © 2014 Pearson Education, Inc. Astrometric Technique We can detect planets by measuring the change in a star's position on sky. However, these tiny motions are very difficult to measure (~ 0.001 arcsecond). © 2014 Pearson Education, Inc. Doppler Technique Measuring a star's Doppler shift can tell us its motion toward and away from us. Current techniques can measure motions as small as 1 m/s (walking speed!). © 2014 Pearson Education, Inc. First Extrasolar Planet Doppler shifts of the star 51 Pegasi indirectly revealed a planet with 4-day orbital period. This short period means that the planet has a small orbital distance. This was the first extrasolar planet to be discovered around a Sun- like star (1995). © 2014 Pearson Education, Inc. First Extrasolar Planet The planet around 51 Pegasi has a mass similar to Jupiter's, despite its small orbital distance. © 2014 Pearson Education, Inc. How can changes in a star's brightness reveal the presence of planets ? © 2014 Pearson Education, Inc. Transits and Eclipses A transit is when a planet crosses in front of a star. The resulting eclipse reduces the star's apparent brightness and tells us planet's radius. No orbital tilt: accurate measurement of planet mass © 2014 Pearson Education, Inc. Kepler NASA's Kepler mission was launched in 2008 to begin looking for transiting planets. It is designed to measure the 0.008% decline in brightness when an Earth-mass planet eclipses a Sun-like star. © 2014 Pearson Education, Inc. Other Planet-Hunting Strategies Gravitational Lensing: Mass bends light in a special way when a star with planets passes in front of another star. Features in Dust Disks: Gaps, waves, or ripples in disks of dusty gas around stars can indicate presence of planets. What have we learned? Why is it so challenging to learn about extrasolar planets? – Direct starlight is billions of times brighter than the starlight reflected from planets. How can a star's motion reveal the presence of planets? – A star's periodic motion (detected through Doppler shifts or by measuring its motion across the sky) tells us about its planets. – Transiting planets periodically reduce a star's brightness. What have we learned? How can changes in a star's brightness reveal the presence of planets? – Transiting planets periodically reduce a star's brightness. – The Kepler mission has found thousands of candidates using this method. https://shorturl.at/IjjT3 The Nature of Planets Around Other Stars Our goals for learning: – What properties of extrasolar planets can we measure? – How do extrasolar planets compare with planets in our solar system? What properties of extrasolar planets can we measure? © 2014 Pearson Education, Inc. Measurable Properties Orbital period, distance, and shape Planet mass, size, and density Atmospheric properties What can Doppler shifts tell us? Doppler shift data tell us about a planet's mass and the shape of its orbit. © 2014 Pearson Education, Inc. Planet Mass and Orbit Tilt We cannot measure an exact mass for a planet without knowing the tilt of its orbit, because Doppler shift tells us only the velocity toward or away from us. Doppler data give us lower limits on masses. © 2014 Pearson Education, Inc. Thought Question Suppose you found a star with the same mass as the Sun moving back and forth with a period of 16 months. What could you conclude? A. It has a planet orbiting at less than 1 AU. B. It has a planet orbiting at greater than 1 AU. C. It has a planet orbiting at exactly 1 AU. D. It has a planet, but we do not have enough information to know its orbital distance. Thought Question Suppose you found a star with the same mass as the Sun moving back and forth with a period of 16 months. What could you conclude? A. It has a planet orbiting at less than 1 AU. B. It has a planet orbiting at greater than 1 AU. C. It has a planet orbiting at exactly 1 AU. D. It has a planet, but we do not have enough information to know its orbital distance. The Kepler 11 system The periods and sizes of Kepler 11's 6 known planets can be determined using transit data. © 2014 Pearson Education, Inc. Calculating density Using mass, determined using the Doppler technique, and size, determined using the transit technique, density can be calculated. © 2014 Pearson Education, Inc. Spectrum During Transit Change in spectrum during a transit tells us about the composition of planet's atmosphere. © 2014 Pearson Education, Inc. Surface Temperature Map Measuring the change in infrared brightness during an eclipse enables us to map a planet's surface temperature. © 2014 Pearson Education, Inc. How do extrasolar planets compare with planets in our solar system? © 2014 Pearson Education, Inc. Orbits of Extrasolar Planets Most of the detected planets have orbits smaller than Jupiter's. Planets at greater distances are harder to detect with the Doppler technique. © 2014 Pearson Education, Inc. Orbits of Extrasolar Planets Orbits of some extrasolar planets are much more elongated (have a greater eccentricity) than those in our solar system. © 2014 Pearson Education, Inc. Orbits of Extrasolar Planets Most of the detected planets have greater mass than Jupiter. Kepler data not included here. Planets with smaller masses are harder to detect with Doppler technique. © 2014 Pearson Education, Inc. Surprising Characteristics Some extrasolar planets have highly elliptical orbits. Planets show huge diversity in size and density. Some massive planets, called hot Jupiters, orbit very close to their stars. What have we learned? What properties of extrasolar planets can we measure? – Orbital properties, such as period, distance, and shape. – Planetary properties, such as mass and size. – Atmospheric properties, such as temperature and composition. What have we learned? How do extrasolar planets compare with planets in our solar system? – Planets with a wide variety of masses and sizes. – Many orbiting close to their stars and with large masses. The Formation of Other Solar Systems Our goals for learning: – Can we explain the surprising orbits of many extrasolar planets? – Do we need to modify our theory of solar system formation? Can we explain the surprising orbits of many extrasolar planets? © 2014 Pearson Education, Inc. Revisiting the Nebular Theory The nebular theory predicts that massive Jupiter- like planets should not form inside the frost line (at 8MSun have short lives, eventually becoming hot enough to make iron, and end in supernova explosions. Low-mass stars with < 2MSun have long lives, never become hot enough to fuse carbon nuclei, and end as white dwarfs. Intermediate-mass stars can make elements heavier than carbon but end as white dwarfs. How are the lives of stars with close companions different? © 2014 Pearson Education, Inc. Thought Question The binary star Algol consists of a 3.7MSun main- sequence star and a 0.8MSun subgiant star. What's strange about this pairing? How did it come about? Thought Question Answers The stars in Algol are close enough that matter can flow from the subgiant onto the main- sequence star. © 2014 Pearson Education, Inc. The star that is now a subgiant was originally more massive. As it reached the end of its life and started to grow, it began to transfer mass to its companion (mass exchange). Now the companion star is more massive. © 2014 Pearson Education, Inc. What have we learned? How does a star's mass determine its life story? – Mass determines how high a star's core temperature can rise and therefore determines how quickly a star uses its fuel and what kinds of elements it can make. How are the lives of stars with close companions different? – Stars with close companions can exchange mass, altering the usual life stories of stars. White Dwarfs Our goals for learning: – What is a white dwarf? – What can happen to a white dwarf in a close binary system? What is a white dwarf? © 2014 Pearson Education, Inc. White Dwarfs White dwarfs are the remaining cores of dead stars. Electron degeneracy pressure supports them against the crush of gravity. © 2014 Pearson Education, Inc. White Dwarfs A white Dwarf is formed from the remains of the nuclear fusion that stopped M Evolving stars: ain (s - -burning hydrogen in a she in tars seq th b u eir ur enc outside the core co nin e b -then burning helium and re g h ra ) yd nc ro h heavier elements ge n White dwarfs cool off and grow dimmer with time as shown in the H-R Diagram. © 2014 Pearson Education, Inc. Size of a White Dwarf G ×(mass) g= (radius)2 White dwarfs with same mass as Sun are about same size as Earth. Higher-mass white dwarfs are smaller. © 2014 Pearson Education, Inc. The White Dwarf Limit Electron quantum (degeneracy) pressure (due to Pauli exclusion principle) balances the inward pull of gravity. Quantum mechanics says that electrons must move faster as they are squeezed into a very small space. As a white dwarf's mass approaches 1.4MSun, its electrons must move at nearly the speed of light. Because nothing can move faster than light, a white dwarf cannot be more massive than 1.4MSun, the white dwarf limit (or Chandrasekhar limit). What can happen to a white dwarf in a close binary system? © 2014 Pearson Education, Inc. What can happen to a white dwarf in a close binary system? A star that started with less mass gains mass from its companion. Eventually, the mass- losing star will become a white dwarf. What happens next? © 2014 Pearson Education, Inc. What can happen to a white dwarf in a close binary system? Mass falling toward a white dwarf from its close binary companion has some angular momentum. The matter therefore orbits the white dwarf in an accretion disk. Friction between orbiting rings of matter in the disk transfers angular momentum outward and causes the disk to heat up and glow. © 2014 Pearson Education, Inc. Thought Question What would the gas in an accretion disk do if there were no friction? A. It would orbit indefinitely. B. It would eventually fall in. C. It would blow away. Thought Question What would the gas in an accretion disk do if there were no friction? A. It would orbit indefinitely. B. It would eventually fall in. C. It would blow away. Nova The temperature of accreted matter eventually becomes hot enough for hydrogen fusion. Fusion begins suddenly and explosively, causing a nova. The nova star system temporarily appears much brighter. The explosion drives accreted matter out into space. © 2014 Pearson Education, Inc. Thought Question What happens to a white dwarf when it accretes enough matter to reach the 1.4MSun limit? A. It explodes. B. It collapses into a neutron star. C. It gradually begins fusing carbon in its core. Thought Question What happens to a white dwarf when it accretes enough matter to reach the 1.4MSun limit? A. It explodes. B. It collapses into a neutron star. C. It gradually begins fusing carbon in its core. Two Types of Supernova Massive star supernova: – Iron core of a massive star reaches white dwarf limit and collapses into a neutron star, causing total explosion. White dwarf supernova: – Carbon fusion suddenly begins as a white dwarf in close binary system reaches white dwarf limit, causing total explosion. Two Types of Supernova One way to tell supernova types apart is with a light curve showing how luminosity changes with time. © 2014 Pearson Education, Inc. Nova or Supernova? Supernovae due to massive star are MUCH MUCH more luminous (about 100 thousand times)!!! Nova: H to He fusion of a layer of accreted matter, white dwarf left intact Supernova from a white dwarf: complete explosion of white dwarf, nothing left behind © 2014 Pearson Education, Inc. Supernova Type: Massive Star or White Dwarf? Light curves differ. Spectra differ (exploding white dwarfs don't have hydrogen absorption lines). What have we learned? What is a white dwarf? – A white dwarf is the inert core of a dead star. – Electron degeneracy pressure balances the inward pull of gravity. What can happen to a white dwarf in a close binary system? – Matter from its close binary companion can fall onto the white dwarf through an accretion disk. – Accretion of matter can lead to novae and white dwarf supernovae. Neutron Stars Our goals for learning: – What is a neutron star? – How were neutron stars discovered? – What can happen to a neutron star in a close binary system? What is a Neutron Star? A neutron star is the ball of neutrons left behind by a massive-star supernova. Degeneracy pressure of neutrons supports a neutron star against gravity. What is a Neutron Star? If the mass of the core is greater than 1.4MSun electron degeneracy pressure goes away because electrons combine with protons, making neutrons and neutrinos. Neutrons collapse to the center, forming a neutron star. © 2014 Pearson Education, Inc. How were neutron stars discovered? © 2014 Pearson Education, Inc. How were neutron stars discovered? Using a radio telescope in 1967, Jocelyn Bell noticed very regular pulses of radio emission coming from a single part of the sky. The pulses were coming from a spinning neutron star - a pulsar. © 2014 Pearson Education, Inc. Pulsars A pulsar is a neutron star that beams radiation along a magnetic axis that is not aligned with the rotation axis. The radiation beams sweep through space like lighthouse beams as the neutron star rotates. Pulsars spin fast because a stellar core's Conservation of spin speeds up as it angular momentum collapses into neutron © 2014 Pearson Education, Inc. star. Why Pulsars Must Be Neutron Stars Circumference of NS = 2π (radius) ~ 60 km Spin rate of fast pulsars ~ 1000 cycles per second Period of rotation ~ 1 ms Surface rotation velocity = 2π (radius) / Period of rotation ~ 60,000 km/s ~ 20% speed of light ~ escape velocity from NS Anything else would be torn (broken) to pieces! Thought Question Could there be neutron stars that appear as pulsars to other civilizations but not to us? A. Yes B. No Thought Question Could there be neutron stars that appear as pulsars to other civilizations but not to us? A. Yes B. No What can happen to a neutron star in a close binary system? © 2014 Pearson Education, Inc. What can happen to a neutron star in a close binary system? Matter falling toward a neutron star forms an accretion disk, just as in a white dwarf binary. Accreting matter adds angular momentum to a neutron star, increasing its spin. Thought Question According to the conservation of angular momentum, what would happen if a star orbiting in a direction opposite the neutron's star rotation fell onto a neutron star? A. The neutron star's rotation would speed up. B. The neutron star's rotation would slow down. C. Nothing. The directions would cancel each other out. Thought Question According to the conservation of angular momentum, what would happen if a star orbiting in a direction opposite the neutron's star rotation fell onto a neutron star? A. The neutron star's rotation would speed up. B. The neutron star's rotation would slow down. C. Nothing. The directions would cancel each other out. © 2014 Pearson Education, Inc. X-Ray Bursts Matter accreting onto a neutron star can eventually become hot enough for helium fusion. The sudden onset of fusion produces a burst of X rays. © 2014 Pearson Education, Inc. What have we learned? What is a neutron star? – It is a ball of neutrons left over from a massive star supernova and supported by neutron degeneracy pressure. How were neutron stars discovered? – Beams of radiation from a rotating neutron star sweep through space like lighthouse beams, making them appear to pulse. – Observations of these pulses were the first evidence for neutron stars. What have we learned? What can happen to a neutron star in a close binary system? – The accretion disk around a neutron star can become hot enough to produce X rays, making the system an X-ray binary. – Sudden fusion events periodically occur on a the surface of an accreting neutron star, producing X-ray bursts. Black Holes: Gravity's Ultimate Victory Our goals for learning: – What is a black hole? – What would it be like to visit a black hole? – Do black holes really exist? What is a black hole? A black hole is an object whose gravity is so powerful that not even light can escape it. © 2014 Pearson Education, Inc. Thought Question What happens to the escape velocity from an object if you shrink it? G ×(mass) A. It increases. g= B. It decreases. (radius)2 C. It stays the same. Hint: © 2014 Pearson Education, Inc. Thought Question What happens to the escape velocity from an object if you shrink it? A. It increases. B. It decreases. C. It stays the same. Hint: © 2014 Pearson Education, Inc. Escape Velocity Initial kinetic Final gravitational energy = potential energy when vesc is equal to c That is the definition of the event horizon. Light would not be able to escape Earth's surface if you could shrink it to < 1 cm "Surface" of a Black Hole The "surface" of a black hole is the radius at which the escape velocity equals the speed of light. This spherical surface is known as the event horizon. The radius of the event horizon is known as the Schwarzschild radius. © 2014 Pearson Education, Inc. Black Holes A black hole's mass strongly warps space and time in the vicinity of its event horizon. © 2014 Pearson Education, Inc. No Escape Nothing can escape from within the event horizon because nothing can go faster than light. No escape means there is no more contact with something that falls in. It increases the hole mass, changes the spin or charge, but otherwise loses its identity. Neutron Star mass Limit Quantum mechanics says that neutrons in the same place cannot be in the same state. Neutron degeneracy pressure can no longer support a neutron star against gravity if its mass exceeds about 3 Msun Some massive star supernovae can make a black hole if enough mass falls onto core. Singularity Beyond the neutron star limit, no known force can resist the crush of gravity. As far as we know, gravity crushes all the matter into a single point known as a singularity. © 2014 Pearson Education, Inc. Thought Question How does the radius of the event horizon change when you add mass to a black hole? A. It increases. B. It decreases. C. It stays the same. Thought Question How does the radius of the event horizon change when you add mass to a black hole? A. It increases. B. It decreases. C. It stays the same. What would it be like to visit a black hole? If the Sun became a black hole, its gravity would be different only near the event horizon. Black holes don't suck! © 2014 Pearson Education, Inc. Effects of a Black Hole: 1. Gravitational Redshift Light waves take extra time and lose energy to climb out of a deep hole in spacetime, leading to a Gravitational Redshift. Effects of a Black Hole: 2. Gravitational Time Dilation Time passes more slowly near the event horizon. Gravitational Time Dilation © 2014 Pearson Education, Inc. Thought Question Is it easy or hard to fall into a black hole? A. easy B. hard Hint: A black hole with the same mass as the Sun wouldn't be much bigger than a college campus. Thought Question Is it easy or hard to fall into a black hole? A. easy B. hard Hint: A black hole with the same mass as the Sun wouldn't be much bigger than a college campus. Effects of a Black Hole: 3. Tidal Elongation and Disruption Tidal forces near the event horizon of a 3 MSun black hole would be lethal to humans. Tidal forces would be gentler near a supermassive black hole because its radius is much bigger. © 2014 Pearson Education, Inc. Do black holes really exist? © 2014 Pearson Education, Inc. Black Hole Verification We need to measure mass by: – Using orbital properties of a companion – Measuring the velocity and distance of orbiting gas It's a black hole if it's not a star and its mass exceeds the neutron star limit (~3MSun) Black Hole Verification Some X-ray binaries contain compact objects of mass exceeding 3 MSun, which are likely to be black holes. One famous X-ray binary with a likely black hole is in the constellation Cygnus. © 2014 Pearson Education, Inc. What have we learned? What is a black hole? – A black hole is a massive object whose radius is so small that the escape velocity exceeds the speed of light. What would it be like to visit a black hole? – You can orbit a black hole like any other object of the same mass—black holes don't suck! – Near the event horizon, time slows down and tidal forces are very strong. What have we learned? Do black holes really exist? – Some X-ray binaries contain compact objects too massive to be neutron stars—they are almost certainly black holes. From the Big Bang to Black Holes Dr. Dario Carbone Stages of Star Birth Our goals for learning: – What slows the contraction of a star-forming cloud? – What is the role of rotation in star birth? – How does nuclear fusion begin in a newborn star? What slows the contraction of a star-forming cloud? © 2014 Pearson Education, Inc. Trapping of Thermal Energy As contraction packs the molecules and dust particles of a cloud fragment closer together, it becomes harder for infrared and radio photons to escape. Thermal energy then begins to build up inside, increasing the internal pressure. Contraction slows down, and the center of the cloud fragment becomes a protostar. How does nuclear fusion begin in a newborn star? © 2014 Pearson Education, Inc. From Protostar to Main Sequence A protostar looks starlike after the surrounding gas is blown away, but its thermal energy comes from gravitational contraction, not fusion. Contraction must continue until the core becomes hot enough for nuclear fusion. Contraction stops when the energy released by core fusion balances energy radiated from the surface—the star is now a main-sequence star. © 2014 Pearson Education, Inc. Birth Stages on a Life Track A life track illustrates a star's surface temperature and luminosity at different moments in time. © 2014 Pearson Education, Inc. Assembly of a Protostar Luminosity and temperature grow as matter collects into a protostar © 2014 Pearson Education, Inc. Convective Contraction Surface temperature remains near 3000 K while convection is main energy transport mechanism. © 2014 Pearson Education, Inc. Radiative Contraction Luminosity remains nearly constant during late stages of contraction, while radiation transports energy through star. © 2014 Pearson Education, Inc. Self-Sustaining Fusion Core temperature continues to rise until star begins fusion and arrives on the main sequence. © 2014 Pearson Education, Inc. Life Tracks for Different Masses Models show that Sun required about 30 million years to go from protostar to main sequence. Higher-mass stars form faster. Lower-mass stars form more slowly. © 2014 Pearson Education, Inc. What have we learned? What slows the contraction of a star-forming cloud? – The contraction of a cloud fragment slows when thermal pressure builds up because infrared and radio photons can no longer escape. What is the role of rotation in star birth? – Conservation of angular momentum leads to the formation of disks around protostars. What have we learned? How does nuclear fusion begin in a newborn star? – Nuclear fusion begins when contraction causes the star's core to grow hot enough for fusion. Masses of Newborn Stars Our goals for learning: – What is the smallest mass a newborn star can have? – What is the greatest mass a newborn star can have? – What are the typical masses of newborn stars? What is the smallest mass a newborn star can have? © 2014 Pearson Education, Inc. Fusion and Contraction Fusion will not begin in a contracting cloud if some sort of force stops contraction before the core temperature rises above 10 K.7 Thermal pressure cannot stop contraction because the star is constantly losing thermal energy from its surface through radiation. Is there another form of pressure that can stop contraction? Degeneracy Pressure The laws of quantum mechanics prohibit two electrons from occupying the same state in same place. © 2014 Pearson Education, Inc. Thermal Pressure: Depends on heat content. Is the main form of pressure in most stars. Degeneracy Pressure: Particles can't be in same state in same place. Doesn't depend on heat content. © 2014 Pearson Education, Inc. Brown Dwarfs Degeneracy pressure halts the contraction of objects with < 0.08MSun before core temperature becomes hot enough for fusion. Starlike objects not massive enough to start fusion are brown dwarfs. © 2014 Pearson Education, Inc. What is the greatest mass a newborn star can have? © 2014 Pearson Education, Inc. Radiation Pressure Photons exert a slight amount of pressure when they strike matter. Very massive stars are so luminous that the collective pressure of photons drives their matter into space. © 2014 Pearson Education, Inc. Upper Limit on a Star's Mass Models of stars suggest that radiation pressure limits how massive a star can be without blowing itself apart. Maximum thought to be around 150MSun, but new observations indicate some may be even larger! © 2014 Pearson Education, Inc. Stars more massive than 150MSun would blow apart. Luminosity Stars less massive than 0.08MSun can't sustain fusion. Temperature © 2014 Pearson Education, Inc. What are the typical masses of newborn stars? © 2014 Pearson Education, Inc. Demographics of Stars Observations of star clusters show that star formation makes many more low-mass stars than high-mass stars. © 2014 Pearson Education, Inc. What have we learned? What is the smallest mass a newborn star can have? – Degeneracy pressure stops the contraction of objects 8MSun Intermediate- Mass Stars Low-Mass Stars < 2MSun Brown Dwarfs © 2014 Pearson Education, Inc. What have we learned? How does a star's mass affect nuclear fusion? – A star's mass determines its core pressure and temperature and therefore determines its fusion rate. – Higher mass stars have hotter cores, faster fusion rates, greater luminosities, and shorter lifetimes. Life as a Low-Mass Star Our goals for learning: – What are the life stages of a low-mass star? – How does a low-mass star die? What are the life stages of a low-mass star? © 2014 Pearson Education, Inc. Thought Question What happens when a star can no longer fuse hydrogen to helium in its core? A. The core cools off. B. The core shrinks and heats up. C. The core expands and heats up. D. Helium fusion immediately begins. Thought Question What happens when a star can no longer fuse hydrogen to helium in its core? A. The core cools off. B. The core shrinks and heats up. C. The core expands and heats up. D. Helium fusion immediately begins. Life Track after Main Sequence Observations of star clusters show that a star becomes larger, redder, and more luminous after its time on the main sequence is over. © 2014 Pearson Education, Inc. Red Giants: Broken Thermostat As the core contracts, H begins fusing to He in a shell around the core. Luminosity increases because the core thermostat is broken. The increasing fusion rate in the shell does not stop the core from contracting. © 2014 Pearson Education, Inc. Helium Fusion Helium fusion does not begin right away because it requires higher temperatures than hydrogen fusion due to larger charge leading to greater repulsion. Fusion of two helium nuclei doesn't work, so helium fusion must combine three helium nuclei to make carbon. © 2014 Pearson Education, Inc. Thought Question What happens in a low-mass star when the core temperature rises enough for helium fusion to begin? A. Helium fusion slowly starts. B. Hydrogen fusion stops. C. Helium fusion rises very sharply. Hint: Degeneracy pressure is the main form of pressure in the inert helium core. © 2014 Pearson Education, Inc. Thought Question What happens in a low-mass star when the core temperature rises enough for helium fusion to begin? A. Helium fusion slowly starts. B. Hydrogen fusion stops. C. Helium fusion rises very sharply. Hint: Degeneracy pressure is the main form of pressure in the inert helium core. © 2014 Pearson Education, Inc. Helium Flash The thermostat of a low-mass red giant is broken because degeneracy pressure supports the core. Core temperature rises rapidly when helium fusion begins. Helium fusion rate skyrockets until thermal pressure takes over and expands the core again. Helium Flash Helium-burning stars neither shrink nor grow because core thermostat is temporarily fixed. Life Track after Helium Flash Red giants shrink and become less luminous after helium fusion begins in the core. © 2014 Pearson Education, Inc. How does a low-mass star die? © 2014 Pearson Education, Inc. Thought Question What happens when the star's core runs out of helium? A. The star explodes. B. Carbon fusion begins. C. The core cools off. D. Helium fuses in a shell around the core. Thought Question What happens when the star's core runs out of helium? A. The star explodes. B. Carbon fusion begins. C. The core cools off. D. Helium fuses in a shell around the core. Double Shell Burning After core helium fusion stops, helium fuses into carbon in a shell around the carbon core, and hydrogen fuses to helium in a shell around the helium layer. This double shell–burning stage never reaches equilibrium—fusion rate periodically spikes upward in a series of thermal pulses. With each spike, convection dredges carbon up from core and transports it to surface. Planetary Nebulae Double shell burning ends with a pulse that ejects the H and He into space as a planetary nebula. The core left behind becomes a white dwarf. © 2014 Pearson Education, Inc. End of Fusion Fusion progresses no further in a low-mass star because the core temperature never grows hot enough for fusion of heavier elements (some helium fuses to carbon to make oxygen). Degeneracy pressure supports the white dwarf against gravity. Life Track of a Sun-like Star © 2014 Pearson Education, Inc. Earth's Fate The Sun's luminosity will rise to 1000 times its current level. Too hot for life on Earth. © 2014 Pearson Education, Inc. Earth's Fate The Sun's radius will grow to near current radius of Earth's orbit. © 2014 Pearson Education, Inc. Low-Mass Star Summary 1. Main sequence: H fuses to He in core. 2. Red giant: H fuses to He in shell around He core. 3. Helium core burning: He fuses to C in core while H fuses to He in shell. 4. Double shell burning: H and He both fuse in shells. 5. Planetary nebula leaves white dwarf behind. © 2014 Pearson Education, Inc. What have we learned? What are the life stages of a low-mass star? – Hydrogen fusion in core (main sequence) – Hydrogen fusion in shell around contracting core (red giant) – Helium fusion in core (horizontal branch) – Double shell burning (red giant) How does a low-mass star die? – Ejection of hydrogen and helium in a planetary nebula leaves behind an inert white dwarf. Life as a High-Mass Star Our goals for learning: – What are the life stages of a high-mass star? – How do high-mass stars make the elements necessary for life? – How does a high-mass star die? What are the life stages of a high-mass star? © 2014 Pearson Education, Inc. Life Stages of High-Mass Stars Late life stages of high-mass stars are similar to those of low-mass stars: – Hydrogen core fusion (main sequence) – Hydrogen shell burning (supergiant) – Helium core fusion (supergiant) How do high-mass stars make the elements necessary for life? © 2014 Pearson Education, Inc. Big Bang made 75% H, 25% He; stars make everything else. © 2014 Pearson Education, Inc. Helium fusion can make carbon in low-mass stars. © 2014 Pearson Education, Inc. CNO cycle can change carbon into nitrogen and oxygen. © 2014 Pearson Education, Inc. Helium capture builds carbon into oxygen, neon, magnesium, and other elements. © 2014 Pearson Education, Inc. Advanced Nuclear Burning Core temperatures in stars with >8MSun allow fusion of elements as heavy as iron. © 2014 Pearson Education, Inc. Advanced reactions in stars make elements like Si, S, Ca, Fe. © 2014 Pearson Education, Inc. Multiple Shell Burning Advanced nuclear burning proceeds in a series of nested shells. © 2014 Pearson Education, Inc. Star Killer Iron is a dead end for fusion because nuclear reactions involving iron do not release energy because iron has lowest mass per nuclear particle. © 2014 Pearson Education, Inc. How does a high-mass star die? © 2014 Pearson Education, Inc. Supernova Explosion Iron builds up in core until degeneracy pressure can no longer resist gravity. The core then suddenly collapses, creating a supernova explosion. © 2014 Pearson Education, Inc. Supernova Explosion Core degeneracy pressure goes away because electrons combine with protons, making neutrons and neutrinos. Neutrons collapse to the center, forming a neutron star. Such star is sustained by neutron degeneracy pressure. © 2014 Pearson Education, Inc. Supernova Explosion If neutron degeneracy pressure is not sufficient as the core is too massive, it will turn into a black hole. © 2014 Pearson Education, Inc. Energy and neutrons released in supernova explosion enable elements heavier than iron to form, including gold and uranium. © 2014 Pearson Education, Inc. Supernova Remnant Energy released by the collapse of the core drives the star's outer layers into space. The Crab Nebula is the remnant of the supernova seen in A.D. 1054. © 2014 Pearson Education, Inc. Supernova 1987A The closest supernova in the last four centuries was seen in 1987. © 2014 Pearson Education, Inc. High-Mass Star Summary 1. Main sequence: H fuses to He in core. 2. Red supergiant: H fuses to He in shell around He core. 3. Helium core burning: 4. He fuses to C in core while H fuses to He in shell. 5. Multiple shell burning: 6. Many elements fuse in shells. 7. Supernova leaves neutron star behind. © 2014 Pearson Education, Inc. What have we learned? What are the life stages of a high-mass star? – They are similar to the life stages of a low-mass star. How do high-mass stars make the elements necessary for life? – Higher masses produce higher core temperatures that enable fusion of heavier elements. How does a high-mass star die? – Its iron core collapses, leading to a supernova. The Roles of Mass and Mass Exchange Our goals for learning: – How does a star's mass determine its life story? – How are the lives of stars with close companions different? Role of Mass A star's mass determines its entire life story because it determines its core temperature. High-mass stars with > 8MSun have short lives, eventually becoming hot enough to make iron, and end in supernova explosions. Low-mass stars with < 2MSun have long lives, never become hot enough to fuse carbon nuclei, and end as white dwarfs. Intermediate-mass stars can make elements heavier than carbon but end as white dwarfs. How are the lives of stars with close companions different? © 2014 Pearson Education, Inc. Thought Question The binary star Algol consists of a 3.7MSun main- sequence star and a 0.8MSun subgiant star. What's strange about this pairing? How did it come about? Thought Question Answers The stars in Algol are close enough that matter can flow from the subgiant onto the main- sequence star. © 2014 Pearson Education, Inc. The star that is now a subgiant was originally more massive. As it reached the end of its life and started to grow, it began to transfer mass to its companion (mass exchange). Now the companion star is more massive. © 2014 Pearson Education, Inc. What have we learned? How does a star's mass determine its life story? – Mass determines how high a star's core temperature can rise and therefore determines how quickly a star uses its fuel and what kinds of elements it can make. How are the lives of stars with close companions different? – Stars with close companions can exchange mass, altering the usual life stories of stars. © 2014 Pearson Education, Inc. From the Big Bang to Black Holes Dr. Dario Carbone https://shorturl.at/IjjT3 What are giants, supergiants, and white dwarfs? © 2014 Pearson Education, Inc. Sizes of Giants and Supergiants © 2014 Pearson Education, Inc. Off the Main Sequence Stellar properties depend on both mass and age: Those that have finished fusing H to He in their cores are no longer on the main sequence. All stars become larger and redder after exhausting their core hydrogen: giants and supergiants. Most stars end up small and white after fusion has ceased: white dwarfs. © 2014 Pearson Education, Inc. Which star is most like our Sun? Luminosit Temperature © 2014 Pearson Education, Inc. Which of these stars will have changed the least 10 billion years from now? Luminosit Temperature © 2014 Pearson Education, Inc. Which of these stars can be no more than 10 million years old? Luminosit Temperature © 2014 Pearson Education, Inc. Variable Stars Any star that varies significantly in brightness with time is called a variable star. Some stars vary in brightness because they cannot achieve proper balance between power welling up from the core and power radiated from the surface. Such a star alternately expands and contracts, varying in brightness as it tries to find a balance. Pulsating Variable Stars The light curve of this pulsating variable star shows that its brightness alternately rises and falls over a 50-day period. © 2014 Pearson Education, Inc. Cepheid Variable Stars Most pulsating variable stars inhabit an instability strip on the H-R diagram. The most luminous ones are known as Cepheid variables. © 2014 Pearson Education, Inc. What have we learned? What is a Hertzsprung-Russell diagram? – An H-R diagram plots stellar luminosity of stars versus surface temperature (or color or spectral type). What is the significance of the main sequence? – Normal stars that fuse H to He in their cores fall on the main sequence of an H-R diagram. – A star's mass determines its position along the main sequence (high-mass: luminous and blue; low-mass: faint and red). What have we learned? What are giants, supergiants, and white dwarfs? – All stars become larger and redder after core hydrogen burning is exhausted: giants and supergiants. – Most stars end up as tiny white dwarfs after fusion has ceased. Why do the properties of some stars vary? – Some stars fail to achieve balance between power generated in the core and power radiated from the surface. Star Clusters Our goals for learning: – What are the two types of star clusters? – How do we measure the age of a star cluster? What are the two types of star clusters? © 2014 Pearson Education, Inc. Open cluster: A few thousand loosely packed stars © 2014 Pearson Education, Inc. Globular cluster: Up to a million or more stars in a dense ball bound together by gravity © 2014 Pearson Education, Inc. How do we measure the age of a star cluster? © 2014 Pearson Education, Inc. How do we measure the age of a star cluster? The Pleiades cluster now has no stars with life expectancy less than around 100 million years. © 2014 Pearson Education, Inc. How do we measure the age of a star cluster? The main-sequence turnoff point of a cluster tells us its age. © 2014 Pearson Education, Inc. How do we measure the age of a star cluster? Detailed modeling of the oldest globular clusters reveals that they are about 13 billion years old. © 2014 Pearson Education, Inc. What have we learned? What are the two types of star clusters? – Open clusters are loosely packed and contain up to a few thousand stars. – Globular clusters are densely packed and contain hundreds of thousands of stars. How do we measure the age of a star cluster? – A star cluster's age roughly equals the life expectancy of its most massive stars still on the main sequence. Star Birth © 2014 Pearson Education, Inc. Stellar Nurseries Our goals for learning: – Where do stars form? – Why do stars form? Where do stars form? © 2014 Pearson Education, Inc. Star-Forming Clouds Stars form in dark clouds of dusty gas in interstellar space. The gas between the stars is called the interstellar medium. © 2014 Pearson Education, Inc. Composition of Clouds We can determine the composition of interstellar gas from its absorption lines in the spectra of stars. 70% H, 28% He, 2% heavier elements in our region of Milky Way © 2014 Pearson Education, Inc. Molecular Clouds Most of the matter in star-forming clouds is in the form of molecules (H2, CO, etc.). These molecular clouds have a temperature of 10–30 K and a density of about 300 molecules per cubic centimeter. © 2014 Pearson Education, Inc. Molecular Clouds Most of what we know about molecular clouds comes from observing the emission lines of carbon monoxide (CO). © 2014 Pearson Education, Inc. Interstellar Dust Tiny solid particles of interstellar dust block our view of stars on the other side of a cloud. Particles are < 1 micrometer in size and made of elements like C, O, Si, and Fe. © 2014 Pearson Education, Inc. Interstellar Reddening Stars viewed through the edges of the cloud look redder because dust blocks (shorter-wavelength) blue light more effectively than (longer-wavelength) red light. © 2014 Pearson Education, Inc. Interstellar Reddening Long-wavelength infrared light passes through a cloud more easily than visible light. Observations of infrared light reveal stars on the