Behavioral Neuroscience Lecture 9 - Vision PDF
Document Details
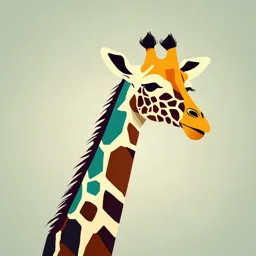
Uploaded by PanoramicComprehension352
McGill University
Jonathan Britt
Tags
Related
Summary
This document is a lecture on vision, discussing sensation, perception, sensory neurons, and photoreceptors. The lecture is part of a behavioral neuroscience course (PSYC 211) and provides an overview of the topics related to visual processing.
Full Transcript
Introduction to Behavioral Neuroscience PSYC 211 Lecture 9 of 24 – Vision (part 1 or 2) Chapter 6-1, 6-2 (p173-188) Professor Jonathan Britt Questions? Concerns? Please write to [email protected] SENSATION VERSUS PERCEPTION Sensation refe...
Introduction to Behavioral Neuroscience PSYC 211 Lecture 9 of 24 – Vision (part 1 or 2) Chapter 6-1, 6-2 (p173-188) Professor Jonathan Britt Questions? Concerns? Please write to [email protected] SENSATION VERSUS PERCEPTION Sensation refers to how cells of the nervous system detect stimuli in the environment (such as light, sound, heat, etc.), and how they transduce (convert) these signals into a change in membrane potential and neurotransmitter release. Perception refers to the conscious experience and interpretation of sensory information. SENSORY NEURONS Sensory neurons are specialized cells that detect a specific category of physical events, such as – the presence of specific molecules smell, taste, nausea, pain – the presence (or absence) of physical pressure touch, stretch, vibration, acceleration, gravity, balance, hearing, thirst, pain – the temperature heat, cold, pain – the pH of a liquid (whether it is acidic or alkaline) sour taste, suffocation, pain – electromagnetic radiation (light) vision Some non-human animals have other senses, such as the ability to detect electrical and magnetic fields, humidity, and water pressure. SENSORY TRANSDUCTION Sensory neurons have specialized receptors that transduce sensory stimuli into a change in membrane potential. Sensory neurons come in all shapes and sizes. Many sensory neurons do not have axons or action potentials, but they all release neurotransmitter. Sensory neurons that do not have action potentials release neurotransmitter in a graded fashion, dependent on their membrane potential. The more depolarized they are, the more neurotransmitter they release. PHOTORECEPTORS Photoreceptor The sensory neurons responsible for vision. cells These cells transduce the electromagnetic energy of visible light into a change in membrane potential, which affects how much neurotransmitter they release. These cells do not have action potentials. Opsins Light-sensitive proteins. The opsins in photoreceptor cells are metabotropic receptors. They are only sensitive to light because they bind a molecule of retinal, which changes shape in response to light. The change in the shape of retinal is what activates this metabotropic receptor. Retinal Small molecule (synthesized from vitamin A) that attaches to the opsin proteins in the photoreceptor cells in our eyes. The retinal molecule is technically what absorbs the electromagnetic energy of visible light that allows us to see. The two configurations of the retinal molecule light enzyme + ATP retinal molecule opsin protein (embedded in the membrane of photoreceptor cells) When retinal absorbs a wavelength of visible light, it activates the opsin protein (a metabotropic receptor). This launches an intracellular g protein signaling cascade that changes the membrane potential of the photoreceptor cell, affecting how much neurotransmitter it releases. NEURAL TRANSDUCTION OF LIGHT 4 types of photoreceptor cells contribute to our conscious perception of vision. Each one expresses a different type of metabotropic opsin protein: 1) red cone cells express the red cone opsin 2) green cone cells express the green cone opsin 3) blue cone cells express the blue cone opsin 4) rod cells express the rhodopsin opsin Each of these opsin proteins are sensitive to different wavelengths of light according to how they hold the retinal molecule. Rod cells (containing the rhodopsin protein) were the last to evolve; they are 100 times more sensitive to light than the cone cells. (Humans express a few other type of opsin proteins, but they are not used for conscious vision perception.) WHAT THE EYES DETECT Visible light refers to electromagnetic energy that has a wavelength between 380 and 760 nm. We detect this light using four kinds of photoreceptor cells (1 rod cell & 3 cone cells). blue green red cone cone cone Cone Photoreceptors: Trichromatic Coding Blue cone opsins are most sensitive to short wavelengths of light Green cone opsins are most sensitive to medium wavelengths of light Red cone opsins are most sensitive to long wavelengths of light The figure shows the absorption spectra of each of our cone opsins. Notice that 610 nm wavelength light (orange) activates green cones 13% of their maximum level and the red cones 75% of their maximum level. Color perception is a function of the relative rates of activity across the three types of cone cells (i.e. colors are discriminated by the ratio of activity across these cells). Cone Photoreceptors: Trichromatic Coding The details get complicated because the three cone cells are not equally sensitivity to light. For example, when people look at red, green, and blue lights of the same intensity, they often say that the green light is brighter (because our green cone opsins are more sensitive to light in general). But the key consideration for our brain for identifying color is how much each type of cone cell is activated relative to its maximum level. Each color of the rainbow corresponds to a particular pattern of activation across the three types of cones cells. Trichromatic Coding When red and green light bulbs are so close together that our eyes can’t differentiate them, the color looks yellow to us. There are 3 kinds of light bulbs here: red, green and blue. Green light (530nm) activates the green cone opsin more than the red cone opsin. Red light (680nm) activates the red cone opsin more than the green cone opsin. The combination of red and green light causes the red and green cone opsins to be activated at similar amounts, which is what happens with yellow light (580nm). Additive versus Subtractive Color The primary colors of light The primary colors of paint Sunlight is white light, since it contains an equal mixture of all the colors. Paint doesn’t produce light; it absorbs some wavelengths of light and reflects others. Yellow, magenta, and cyan are the three primary colors of office printers because each of these dyes only absorbs (subtracts) one color from white light (from sunlight), while reflecting the other colors. For example, yellow is a primary paint color because it only absorbs one color (blue) from white light. Yellow paint reflects green and red light, which in combination we perceive as yellow. Red is not a primary paint color because red paint simultaneously absorbs both blue and green light. Printers create red by mixing yellow and magenta paint, which absorb blue and green light, respectively. Note that it is advantageous to paint with chemicals that only absorb one color, because otherwise you quickly end with black when mixing different paints together (when all light is absorbed, none is reflected). You do not need to know about paint or the concept of subtractive color for this class. PERCEPTUAL DIMENSIONS OF COLOR & LIGHT Our perception of light and color has three dimensions to it: 1) Brightness – intensity (luminance, amount) 2) Saturation – purity (in terms of wavelength mixture) 3) Hue – dominant wavelength (color) 0 to 360 100% 0% degrees If brightness is zero, the image is completely black. Hue and saturation have no impact without brightness. If there is (bright) light, the next question is whether the light is saturated with a particular wavelength (color). If saturation is 0%, then you are in the http://jamiewayne.com/wp-content/uploads/2011/05/hsb.jpg middle of the color cone where there is an equal contribution from all visible wavelengths. An image with 0% saturation is grayscale (black and white), because all wavelengths are present in equal amounts. If saturation is >0%, the hue indicates the color that the light is saturated with. 0 COLOR VISION DEFICIENCY Protanopia Absence of the red cone opsin (1% of males). People with this condition have trouble distinguishing colors in the green-yellow-red spectrum. Visual acuity is normal because red cone cells switch to using the green cone opsin. Simple mutations in of the red cone opsin (1% of males) produce less pronounced deficits in color vision. Deuteranopia Absence of the green cone opsin (1% of males). Visual acuity is normal because green cone cells switch to using the red cone opsin. People with this inherited condition have trouble distinguishing colors in the Mutations in the red cone opsin green-yellow-red section of the spectrum. hinder color vision if they make Simple mutations in of the green cone opsinit(6% of males) act more produce like the less green opsin pronounced deficits in color vision. (in terms of what light it can detect). Tritanopia Absence of the blue cone opsin (1% of the population). Blue cone cells do not compensate for this in any way, but since the blue cone opsin is not that sensitive to light anyway, visual acuity is not noticeably affected. COLOR VISION DEFICIENCY Protanopia Absence of the red cone opsin (1% of males). People with this condition have trouble distinguishing colors in the green-yellow-red spectrum. Visual acuity is normal because red cone cells switch to using the green cone opsin. Simple mutations in of the red cone opsin (1% of males) produce less pronounced deficits in color vision. Deuteranopia Absence of the green cone opsin (1% of males). People with this condition have trouble distinguishing colors in the green-yellow-red spectrum. Visual acuity is normal because green cone cells switch to using the red cone opsin. Simple mutations in of the green cone opsin (6% of males) produce less pronounced deficits in color vision. Tritanopia Absence of the blue cone opsin (1% of the population). Blue cone cells do not compensate for this in any way, but since the blue cone opsin is not that sensitive to light anyway, visual acuity is not noticeably affected. COLOR VISION DEFICIENCY Normal Vision Deuteranopia (no green cone) Protanopia Tritanopia (no red (no blue cone) cone) True color blindness is called achromatopsia. It is typically caused by mutations in the g protein signaling cascade that is used by all the cone opsins. WHAT THE EYES DETECT Visible light refers to electromagnetic energy that has a wavelength between 380 and 760 nm. We detect this light using four kinds of photoreceptor cells (1 rod cell & 3 cone cells). rhodopsin blue green red cone cone cone Rod cells are 100x more sensitive to light than any of the cone cells. They are in a different category. Cone cells are useful because there are three of them, and they are sensitive to different wavelengths, which is necessary for color vision. ANATOMY OF THE EYE The conjunctiva is a mucous membranes that line the eyelid The cornea is the outer, front layer of the eye. It focuses incoming light a fixed amount. The sclera is opaque and does not permit entry of light ANATOMY OF THE EYE The iris is a ring of muscle. The contraction and relaxation of this muscle determines the size of the pupil, which determines how much light enters the eye. The lens consists of several transparent layers. We change the shape of this lens to focus near versus far, a process known as accommodation. ANATOMY OF THE EYE The interior lining (furthest back part) of the eye is the retina. Photoreceptor cells are located in the furthest back layer of the retina. Light passed through the lens and crosses the vitreous humor, a clear, gelatinous fluid. The central region of the retina is called the fovea. It primarily contains cone cells. The periphery of the retina only contains rod cells. The optic disk is where blood vessels enter and leave the eye. It is also where the optic nerve exits the eye, carrying visual information to the brain. There are no photoreceptors in this spot, so it is a blind spot. THE BLIND SPOT MOVEMENTS OF THE EYE Eyes are suspended in bony sockets in the front of the skull called orbits. Our eyes never sit still for very long. Our eyes scan a scene by making saccadic eye movements – rapid, jerky shifts in gaze from one point to another. However, when we maintain focus on an object that is moving (relative to us) our eyes exhibit pursuit movements. This is the only time are eyes appear to calm down and move smoothly, slowly. Six extraocular muscles are attached to the sclera: the tough, outer white of the eye. These muscles rotate the eye and hold it in place. ORGANIZATION OF THE RETINA Visual information propagates from photoreceptor cells → bipolar cells → retinal ganglion cells → brain. So, when light enters our eyes, it must pass through each of the cell layers in the retina before it can reach the opsin proteins in photoreceptor cells. There does not seem to be a good reason for this awkward arrangement. Opsin proteins Light are here, in the furthest back of the retina. neural activity to the brain RETINA FOVEA VERSUS PERIPHERY In the fovea, there is an equal number of photoreceptor cells, bipolar cells, and retinal ganglion cells. This means there is no compression of information. The fovea is the only part of our retina where our visual acuity is good enough to read text (20/20 vision). And the photoreceptor cells in our fovea are mostly cone cells, which support color vision, so the fovea supports high resolution, color vision. Outside the fovea (in the periphery of our retina), there is a massive compression (averaging) of information, since there are 100x more photoreceptor cells than retinal ganglion cells. Our visual acuity in peripheral vision is about 20/200, which is quite blurry. It is also gray scale. We can make out general shapes but not any details. Yet, the periphery of our retina contains a high density of rod cells, which are very sensitive to light, allowing us to easily detect dim light and movements of light. ❖ Our fovea supports high resolution color vision, but only when there is a sufficient amount of light. At night, the moon must be at least half full for us to see in color (if there are no other sources of light.) Light ❖ Our peripheral vision is very sensitive to dim light, but it only provides low resolution grayscale images. What we see in peripheral vision 20 feet away is as detailed as what we see in our fovea 200 feet away. PERIPHERAL VISION People have poor visual acuity and are not great at identifying colors in their peripheral vision. We can still make out general shapes, but images look blurry, unfocused. The high visual acuity needed for reading is only possible in the fovea, where we also see in color. ROD AND CONE PHOTORECEPTORS NEURONS IN THE RETINA Back of Light eye Photoreceptor cells are located in the furthest back part of the retina. They express the opsin proteins that transduce light. Photoreceptor cells synapse on bipolar cells. Bipolar cells relay information from photoreceptor cells to retinal ganglion cells. Retinal ganglion cells are the only cells that send information out of the eye. Their axons form the optic nerve, which exits the retina through the optic disc (the blind spot of the retina). Horizontal cells and amacrine cells interconnect cells within each layer, which gives rise to complex interactions between neighboring cells (within a layer). VISUAL INFORMATION PATHWAYS Retinal ganglion cells have action potentials, unlike most other cells in the retina. Their axons go to 3 places: Thalamus (specifically the lateral geniculate nucleus), which in turn projects to primary visual cortex (area V1) in the occipital lobe where visual information enters consciousness. This retina→thalamus→V1 pathway creates an internal (mental) representation of your entire visual space: the objects in it, their position, and their attentional value. Midbrain (specifically the superior colliculi): Visual information is used here to control fast visually-guided reflexive movements. The midbrain doesn’t know what you are looking at, but it can draw attention to unexpected visual events. Hypothalamus: Visual information is used here to control circadian rhythms such as sleep-wake cycles. The hypothalamus doesn’t know what you are looking at, but it knows how much light is present in your environment. VISUAL CORTEX WIRING DIAGRAM The wiring diagram of visual information processing as it was understood in the 1970s. This diagram is an oversimplification because there are actually tons more pathways and most of them are bidirectional (bottom-up and top-down). One interesting theory of sensory processing is the predictive coding theory. The idea is that each node in the network tries to predict what its ascending inputs will look like in the next moment, based on previous experience. The idea is that top-down (descending) activity represents sensory predictions that neutralize (cancel out) any correctly predicted bottom-up ascending signals. Thus, what propagates up through the network may only be prediction error signals, which inform the brain of how the current moment differs from what was expected. The prediction error signals that ascend through the network would cause learning to improve future predictions.