DNA Replication, Transcription, and Translation Lecture Notes PDF
Document Details
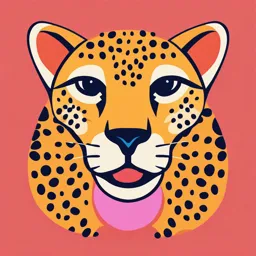
Uploaded by ClaraJeniffer1
University of Silesia in Katowice
2023
Tags
Related
- DNA Replication, Transcription, and Translation PDF
- DNA Replication, Transcription, and Translation PDF
- Cell Division, DNA Replication, Transcription, Translation PDF
- DNA Structure, Replication, Transcription, and Translation PDF
- PL1003 Lecture 3.3 - DNA Replication, Transcription, & Translation PDF
- DNA Expression 12th Grade PDF
Summary
These lecture notes cover the fundamental processes of DNA replication, transcription, and translation. The document details the stages, enzymes, and proteins involved in each of these processes and includes diagrams to illustrate the various interactions. The focus is on molecular biology principles.
Full Transcript
DNA replication, transcription and translation Molecular biology, 2023/2024 Book Reference Essential Cell Biology by Bruce Alberts, 6th Edition. Focus on what is discussed in the lectures ⚫ Chapter 6: DNA Replication and Repair ⚫ Chapter 7: From DNA...
DNA replication, transcription and translation Molecular biology, 2023/2024 Book Reference Essential Cell Biology by Bruce Alberts, 6th Edition. Focus on what is discussed in the lectures ⚫ Chapter 6: DNA Replication and Repair ⚫ Chapter 7: From DNA to Protein DNA replication Replication is a semiconservative process because each strand of the double helix is a template for the synthesis of a new, complementary strand DNA synthesis occurs only in the 5’ → 3’ direction Newly added nucleotides are joined by a phosphodiester bond This process: ❑ is enzymatic because it requires numerous protein complexes to be completed ❑ requires energy input, which comes from the hydrolysis of high-energy phosphate bonds Semiconservative nature of DNA replication Replication proteins Helicase: Protein MCM 2-7 (MiniChromosome maintenance protein complex 2-7) Topoisomerases Replication Protein A (RPA) Proliferating Cell Nuclear Anitgen (PCNA) Replication Factor C (RFC) DNA Polymerases (, , , , ) RNase H (endonucleases) FEN1 (exonuclease) Ligase DNA Helicase The MCM 2-7 complex consists of six identical subunits that form a ring encompassing a single strand of DNA This enzyme complex breaks hydrogen bonds between complementary nitrogenous bases and is a DNA helicase The MCM 2-7 proteins hydrolyze ATP as they move along the strand splitting apart the complementary DNA strands Topoisomerases Unwinding of DNA strands by helicase generates torsional stress (a twisting tension) in DNA If the tension is very high, it prevents further unwinding of the DNA strand Topoisomerases change the topology of DNA reducing the tension within the affected molecule There are two types of topoisomerases: ❑ topoisomerase I ❑ topoisomerase II Torsional stress of DNA RPA After the complementary DNA strands are separated by helicase, single-stranded DNA fragments are created They tend to form short, double-stranded fragments that are an obstacle to DNA polymerase's action The RPA protein solves this problem because it binds to single- stranded DNA segments which: ❑ prevents the formation of hydrogen bonds ❑ allows DNA polymerase to synthesize a new DNA chain RPA Helicase Hairpin structure makes replication difficult RPA proteins PCNA PCNA consists of three subunits forming a ring-shaped homotrimer PCNA is placed on the DNA strand with the participation of RFC PCNA surrounds the DNA strand (i.e., acts as a scaffold) for the recruitment of proteins involved in DNA replication and repair, chromatin remodeling and epigenetic regulation PCNA moves along the DNA strand during replication PCNA The PCNA : ❑ protects DNA polymerase from falling off the template strand ❑ releases DNA polymerase after each Okazaki fragment is synthesized RFC RFC (ring-applying protein) RFC consists of five different subunits RFC opens the PCNA protein trimer in the presence of ATP, introduces DNA into the center of the ring, and then closes the ring In the case of the leading strand, this is a one-time process In the case of the lagging strand, due to the synthesis of Okazaki fragments, the PCNA protein is placed on the DNA strand multiple times Construction of the PCNA and RFC complex DNA polymerase Characteristics of DNA polymerases: ❑ require the presence of a DNA template ❑ are unable to start the synthesis of a complementary strand on the DNA template - a primer is required ❑ have only polymerase activity for DNA synthesis in the 5’ → 3’ direction ❑ have corrective activity: 3’ → 5’ exonuclease activity Polymerase α/primase Polymerase α/primase (DNA-dependent RNA polymerase) It has primase and DNA polymerase activity Primase synthesizes an RNA primer (iRNA, primer), which is a short strand of 10 RNA nucleotides complementary to that of the sequence of a single DNA strand Polymerase α adds a 20 - 30 nucleotide section of DNA (iDNA, initiator DNA) to the primer Then polymerases δ and ε take over the synthesis of daughter strands of DNA Primase activity Polymerases i Polymerases δ (d) and ε (e) are composed of four subunits of different molecular weights, respectively: ❑ δ - p125, p66, p50, p12 ❑ ε - p256, p80, p23, p22 Polymerase δ prefers leading strand replication Polymerase ε prefers lagging strand replication Both polymerases exhibit both 5’→ 3’ polymerase and 3’→ 5’ exonuclease activity Polymerase catalytic domains DNA polymerases During DNA replication, DNA polymerases proofread their own work, which is why they operate in two modes: ❑ polymerization (P) ❑ editing (E) If DNA polymerase introduces an incorrect nucleotide into a new strand, its shape changes and it enters into proofreading mode It then becomes a 3’ → 5' exonuclease and cuts out the incorrectly introduced nucleotide After removing the incorrect nucleotide, the polymerase enters polymerization mode and continues DNA replication DNA polymerases Ribonucleases RNAses are enzymes from the hydrolase class and divide RNA molecules into shorter chains or single nucleotides by hydrolyzing phosphodiester bonds Endoribonucleases divide RNA molecules in the middle of the RNA chain Exoribonucleases detach nucleotides from the 3' or 5' end of RNA Replication proteins RNAse H is an endoribonuclease that removes the iRNA primer, except for the last ribonucleotide in the DNA chain FEN1 (Flap endonuclease 1) is an exoribonuclease that removes the last ribonucleotide of the iRNA primer Ligase joins: ❑ deoxyribonucleotides to a DNA strand by forming phosphodiester bond using ATP ❑ Okazaki fragments on the lagging strand Limitations of the replication process The need to unwind the DNA double helix The inability of DNA polymerase to independently initiate the synthesis of a new DNA strand The addition of nucleotides to single DNA strand can only occur in the 5’→ 3’ direction Limitations of the replication process Synthesis of a new DNA strand begins with primer: ❑ the primer is synthesized by the primase unit of polymerase α, which synthesizes 10 nucleotides of the iRNA strand ❑ polymerase α extends the primer to synthesize the iDNA strand ❑ after adding 20 or 30 deoxynucleotides of DNA, polymerase or begins its action DNA replication Stages of DNA replication: ❑ initiation (start) ❑ elongation (extension) ❑ termination (ending) Initiation - recognition of places within the DNA strand from which replication begins Elongation - the action of replication forks and copying of parent DNA strands Termination - completion and assembly of new DNA strands Initiation of DNA replication In mammals, the unwinding of the DNA helix occurs at origins of replication and involves a specific sequence of 10,000 base pairs Replication occurs simultaneously at many sites in the DNA, which is why there are many origins in chromosomes Their clustering are observed in sparse clusters, called "replication factories” or "replication foci" A fragment of DNA replicated from one origin is called a replicon. Initiation of DNA replication Initiation of DNA replication Formation of the pre-replication complex (pre-RC): ❑ beginning is the attachment of the ORC (Origin Recognition Complex) at the DNA origin of replication site ❑ The ORC is composed of six protein domains ❑ After binding to DNA, ORC combines with additional proteins, Cdt 1 and Cdc 6 to recruit helicase completing the formation of the pre-RC Initiation of DNA replication Initiation of DNA replication DNA replication initiation stages: ❑ DNA helix separation via helicase ❑ DNA supercoil relaxation (topoisomerase) ❑ replication "bubble” formation ❑ "bubble” transformation into replication forks ❑ obtaining two single-stranded DNA (ssDNA) templates ❑ ssDNA stabilization by RPA proteins Initiation of DNA replication http://www.chemiabioorganiczna.polsl.pl Replication forks Due to the antiparallel arrangement of DNA strands and the synthesis of new strands taking place in the 5’ → 3’ direction, replication forks are asymmetric For this reason: ❑ one new DNA strand is synthesized continuously - the leading strand ❑ The second new DNA strand is synthesized in sections called Okazaki fragments - the lagging strand Replication forks Replication forks Overview of replication DNA replication elongation DNA copying stages on the leading strand: ❑ synthesis of iRNA strand - primer (primase in α-polymerase) ❑ synthesis of iDNA strand in the 5’ → 3’ direction (α polymerase) ❑ recognition of the primer - template DNA hybrid by the RFC, which then deposits the PCNA at this site ❑ exchange of α-polymerase for δ-polymerase, ❑ synthesis of DNA strand in the 5’→ 3’ direction by δ-polymerase DNA replication elongation DNA copying steps on the leading strand (continued): ❑ hydrolysis of one of the phosphate bonds of deoxynucleotide triphosphate - the energy needed to attach it to the DNA strand ❑ formation of a phosphodiester bond between the 3' end of the last deoxynucleotide in DNA and the 5' end of the new deoxynucleotide ❑ release of pyrophosphate (PPi) ❑ hydrolysis of pyrophosphate (PPi) makes the polymerization reaction essentially irreversible DNA replication elongation DNA replication elongation DNA copying stages on the lagging strand: ❑ DNA synthesis is discontinuous ❑ synthesis of subsequent iRNA strands - primers (primase in α-polymerase) ❑ synthesis of subsequent iDNA strands in the 5' → 3' direction (α-polymerase) ❑ recognition of the primer - template DNA hybrid by the RFC, which then deposits the PCNA at this location ❑ exchange of α-polymerase for ε-polymerase DNA replication elongation DNA copying stages on the lagging strand (continued): ❑ DNA strand synthesis in the 5’ → 3’ direction by ε-polymerase - creation of the Okazaki fragment ❑ PCNA transfer ❑ after reaching the previous primer, α-polymerase with primase is detached from the template ❑ DNA strand synthesis in the 5’ → 3’ direction by ε-polymerase ❑ removal of the iRNA and iDNA fragments ❑ exchange of ε-polymerase for α-polymerase ❑ synthesis of new iRNA and then iDNA Overview DNA replication on the lagging strand DNA replication on the lagging strand DNA replication elongation Creating a continuous new DNA strand from Okazaki fragments requires: ❑ removing the iRNA and iDNA primer (RNase H/FEN1) ❑ replacing it with a DNA fragment (ε polymerase) ❑ joining adjacent DNA fragments together with ligase Ribonuclease activity Ligase action ligase Replication machine Replication termination Replication is terminated when a pair of replication forks meet In eukaryotes, replication forks meet multiple times The details of how forks join are not well understood Replication termination Termination steps: ❑ encounter of a terminal sequence ❑ disintegration of the replication complex ❑ telomere synthesis Replication termination Lagging strand synthesis requires multiple primer synthesis, which is eventually removed In the case of a linear DNA molecule, the lagging strand is always shorter than the template (at least by the length of the primer) This situation occurs at the end of the chromosome (i.e., in the telomeric part) Thanks to telomeres, the problem of shortening the DNA strand after each division is minimized Replication termination Eukaryotic gene Transcription Transcription is the process of rewriting genetic information contained in DNA molecule onto RNA molecule It is based on the complementarity rule that applies during the pairing of nitrogenous bases (A - U, C - G) The unit of transcription is the section of DNA located between the promoter and the terminator The DNA strand is read in the 3’ → 5' direction The RNA molecule (mRNA, tRNA and rRNA) is synthesized in the 5' → 3' direction The process is catalyzed by RNA polymerases I, II and III Transcription RNA polymerase I occurs in nucleolus and is involved in transcription of genes encoding ribosomal RNA (rRNA) RNA polymerase II occurs in nucleoplasm and is involved in transcription of genes encoding messenger RNA (mRNA) RNA polymerase III occurs in nucleoplasm and is involved in transcription of genes encoding transport RNA (tRNA) All types of RNA are synthesized in the form of precursors (i.e., pre-rRNA, pre-mRNA, and pre-tRNA) All types of RNA undergo various modifications to become functional Transcription Transcription Transcription stages: ❑ initiation ❑ elongation ❑ termination Initiation of mRNA transcription A complex of two proteins recognizes the promoter and creates platform to which the following bind: Transcription factors (one of which is helicase) RNA polymerase II The transcription factors and RNA polymerase II form pre-initiation complex that binds to the gene promoter, allowing RNA polymerase II to initiate transcription of the pre- mRNA Elongation of mRNA transcription Once elongation begins, most transcription factors detach from the DNA The first step in freeing RNA polymerase II from the transcription factors is the addition of phosphate groups to its "tail” More ribonucleosides are added A pre-mRNA molecule is synthesized on a strand of DNA called the template strand Termination of mRNA transcription RNA polymerase II continues transcription until it receives signal to end transcription Transcription terminator is a fragment of DNA sequence that marks the end of gene Termination steps: ❑ phosphodiester bond formation ceases ❑ pre-mRNA dissociates from the DNA-RNA hybrid ❑ the unravelled DNA fragment joins Transcription steps Post-transcriptional processing Post-transcriptional processing, or maturation of pre-mRNA, includes: ❑ adding a cap to the 5’ end ❑ cutting out introns and joining exons (splicing) ❑ adding series of adenine nucleotides to the 3’ end (polyadenylation). Mature mRNA Translation Translation is the process of synthesizing a polypeptide chain from an mRNA Translation takes place in the cytoplasm and/or on the membranes of rough endoplasmic reticulum This process is catalyzed by the ribosome The core of the translation process includes: ⚫ synthesis of a polypeptide chain according to the information stored in a DNA fragment (i.e., a gene) ⚫ formation of peptide bonds between consecutive amino acids TRANSLATION PROTEIN BIOSYNTHESIS Ribosome structure Each ribosome is made up of two matched subunits: small and large Both subunits of a ribosome are made up of proteins and rRNA The subunits of a ribosome are only connected during translation The small subunit ribosome has a mRNA binding site Both subunits participate in the formation of tRNA binding sites: ❑ A - aminoacyl-tRNA site ❑ P - peptidyl-tRNA site ❑ E - exit site Ribosome structure Translation Stages of translation: ❑ initiation ❑ elongation ❑ termination Each of these three stages requires the presence of specific protein factors Translation initiation Stages of translation initiation: ❑ formation of pre-initiation complex, consisting of: ❑ small ribosomal subunit ❑ initiation factors ❑ formation of initiator tRNA Initiator tRNA The synthesis of every protein begins with formylmethionine, which is the first amino acid of every peptide chain There are two types of tRNA that can bind methionine, one of which: ❑ participates in initiation - fmet-tRNAf ❑ participates in elongation - met-tRNAm Only fmet-tRNAf can bind to the P site in the absence of the large ribosomal subunit Attaching tRNA to amino acid Aminoacyl-tRNA synthetase attaches its specific amino acid to each type of tRNA Translation initiation There are no signal sequences before the AUG start codon in the mRNA that would help find the translation start site Therefore, there is a complex that is formed consisting of: ❑ small ribosomal subunit ❑ initiator fmet-tRNAf ❑ mRNA This complex searches for (scanning) the first AUG start codon in the mRNA Translation initiation Translation initiation When the AUG codon is recognized on the mRNA: ❑ translation initiation factors detach from small ribosomal subunit ❑ large ribosomal subunit binds and ribosome is formed ❑ the A site is ready to accept another aminoacyl-tRNA Translation initiation Translation elongation Translation elongation occurs in a cycle consisting of several stages Stage 1: ❑ tRNA carrying the next amino acid binds to the free A site of the ribosome ❑ base complementation of tRNA anticodon with mRNA codon occurs Translation elongation Translation elongation Stage 2: ❑ the first peptide bond is formed ❑ peptidyl transferase (rRNA, ribozyme) is responsible for this reaction ❑ the reaction is carried out at catalytic site of large subunit ❑ the peptide is temporarily bound to the tRNA located in site A Translation elongation Translation elongation Step 3: ❑ occurs after peptide bond formation ❑ tRNA without amino acid moves to site E ❑ peptidyl-tRNA moves from site A to site P Step 4: ❑ small subunit ribosome moves three nucleotides along the mRNA molecule ❑ tRNA without an amino acid is removed from site E ❑ site A is freed for another amino acid-linked transport RNA, (i.e., aa-tRNA) Translation elongation Step 3 Translation elongation Step 4 Translation elongation Termination of translation Termination of translation is signaled by the presence of one of the STOP codons in the mRNA: UAA, UAG and UGA, respectively STOP codons: ❑ are not recognized by tRNA ❑ do not encode an amino acid ❑ inform the ribosome about the end of translation Termination of translation Proteins called release factors bind to STOP codon, which occupies the A site in ribosome This causes change in activity of peptidyl transferase, which adds water molecules to peptidyl-tRNA instead of an amino acid Termination of translation Termination of translation Detachment of the carboxyl (COOH) terminus of the polypeptide chain from the last tRNA molecule results in: ⚫ release of the full-length protein ⚫ release of the mRNA molecule from the ribosome ⚫ disintegration of the ribosome into two subunits Termination of translation Post-translational modifications Many proteins require post-translational modifications to achieve full functionality They consist of: ❑ proteolytic processing ❑ formation of secondary structure ❑ protein folding Post-translational modifications So-called chaperone proteins are involved in the correct folding of proteins The chaperone proteins bind to the newly formed polypeptide chain After the polypeptide chain folds correctly, the chaperone proteins dissociate The incorrectly folded protein is degraded Some chaperone proteins create isolation chambers that facilitate protein folding Post-translational modifications Post-translational modifications Post-translational modifications Biochemical processes used to modify proteins: ❑ phosphorylation - adding phosphate group ❑ dephosphorylation - removing phosphate group ❑ glycosylation - adding sugar residues ❑ hydroxylation - adding hydroxyl group ❑ acetylation - adding acetyl group ❑ methylation - adding methyl group ❑ formylation - adding formyl group Post-translational modifications