University of Guyana Biochemistry II - Lecture 7 PDF
Document Details
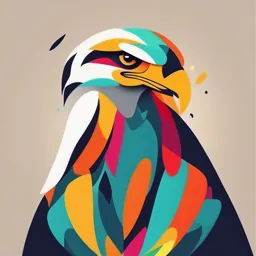
Uploaded by PositivePythagoras7633
University of Guyana
Tags
Summary
This lecture outlines amino acid oxidation and the urea cycle, covering the processes in different metabolic conditions and the fate of alpha-amino groups. It includes details about transamination, deamination, the role of glutamate, and the urea cycle. This document appears to be a lecture on biochemistry, likely part of a university course.
Full Transcript
UNIVERSITY OF GUYANA FACULTY OF NATURAL SCIENCES DEPARTMENT OF BIOLOGY BIO 3110 BIOCHEMISTRY II – Intermediary Metabolism LECTURE 7: Amino Acid Oxidation and the Urea Cycle Introduction Proteins are nitrogen-containing macromolec...
UNIVERSITY OF GUYANA FACULTY OF NATURAL SCIENCES DEPARTMENT OF BIOLOGY BIO 3110 BIOCHEMISTRY II – Intermediary Metabolism LECTURE 7: Amino Acid Oxidation and the Urea Cycle Introduction Proteins are nitrogen-containing macromolecules consisting of L-ἀ- amino acids. Nitrogen enters the body in a variety of compounds present in the food, the most important being amino acids present in the dietary protein. Amino acids are the final class of biomolecules whose oxidation makes a significant contribution towards the generation of metabolic energy. Amino acid catabolism is part of whole-body catabolism. Carnivores: derive up to 90% of their energy requirements from amino acid oxidation. Herbivores: obtain only a small fraction of their energy needs from amino acids. Microorganisms: scavenge amino acids from their environment if they are available; these can be oxidized as fuel when the metabolic conditions so demand. Photosynthetic plants: rarely oxidise amino acids to provide energy. Instead, they convert CO2 and H2O into the carbohydrate glucose that is used almost exclusively as an energy source. In animals, the amino acids can beoxidatively degraded in 3 different metabolic conditions: 1. During normal protein synthesis: some of the amino acids released during protein breakdown will undergo oxidative degradation. 2. During a protein-rich diet: the surplus may be catabolized and amino acids cannot be stored. 3. During starvation or in Diabetes mellitus: body proteins are used as fuel. Under these different circumstances, amino acids lose their amino groups, and the resulting α-keto acids may undergo oxidation to produce CO2 and H2O. Excess amino acids cannot be stored nor are they excreted. The surplus amino acids are used as metabolic fuel. The alpha-amino group of the amino acids is removed and the resulting carbon skeleton is converted into a major metabolic intermediate. Most of the amino groups of surplus amino acids are converted into urea whereas their carbon skeletons are transferred into acetyl-CoA, acetoacetyl-CoA, pyruvate or one of the intermediates of the citric acid cycle. The major site of amino acid degradation in mammals is the liver. Dietary Protein is enzymatically degraded to amino acids Nonprotein Nitrogen compounds Catabolism of amino acids leads to a net loss of nitrogen from the body (must be compensated by the diet to maintain a constant amount of body protein). However, one major factor distinguishes amino acid degradation from the catabolic processes described till now, i.e., every amino acid contains an amino group. As such, every degradative pathway passes through a key step in which the α-amino group is separated from the carbon skeleton and shunted into the specialized pathways for amino group metabolism. Catabolism of Amino Acids Ammonia Amino acid Catabolism in Mammals Fate of alpha-amino group of amino acids The presence ofthe alpha-amino group keeps amino acids safely locked away from oxidative breakdown. Removalof the alpha-amino group is an obligatory step in the catabolism of all amino acids. Once removed, this nitrogen can be incorporated into other compounds or excreted, with the carbon skeleton being metabolized. A. Transamination Transfer of an amino group from an amino acid to a keto acid, with the formation of a new amino acid and a new keto acid. Example: transfer of α-amino group to α-carbon of α-ketoglutarate. Steps: 1. The reaction is catalyzed by transaminases (aminotransferases). Old keto acid New amino acid Old amino acid New keto acid 2. Pyridoxal phosphate (PLP) is the co-factor for this class of reaction called transaminations. PLP is related to vitamin B6. - prosthetic group, carries amino acids to the active site. Where amino group is attached 3. The amino group is transferred to the alpha-ketoglutarate leaving behind the alpha-keto acid analog of the amino acid. Characteristics of transamination Pyridoxal phosphate (PLP) is the cofactor. Transamination is reversible. It involves anabolism and catabolism. There is no free NH3 liberated, only transfer of the amino group All amino acids undergo transamination reactions except lysine, threonine, proline, and hydroxyl proline. B. Deamination Deamination is the removal of an amino group from amino acids, as free NH3. This occurs both as oxidative deaminationand non- oxidative deamination. 1. Oxidative deamination Removal of NH3 from amino groups of glutamate, coupled with oxidation. Glutamate is transported from the cytosol to the mitochondria where it undergoes oxidative deamination. The reaction is catalyzed by L- glutamate dehydrogenase and alpha-ketoglutarate and ammonia (NH4+) are produced. Direct removal of an amide function group. An example would be the second half of the reaction below: The glutamate that is formed channels these amino groups into biosynthetic pathways or into terminal pathways where the nitrogenous waste are eliminated as ammonia or urea. Role of glutamate Glutamate occupies a central place in the amino acid metabolism. It acts as a collector of the amino group of the amino acids. All the amino nitrogen from amino acids that undergo transamination can be concentrated in glutamate. L-glutamate is the only amino acid that undergoes oxidative deamination at an appreciable rate in mammalian tissues. The formation of ammonia from alpha-amino groups thus occurs mainly via the alpha-amino nitrogen of L-glutamate. 2. Non-Oxidative deamination Direct deamination, without oxidation. Substrate: -OH group bearing amino acids such as serine, threonine, and homoserine (hydroxyl amino acids). - They undergo non-oxidative deamination catalyzed by PLP dependent dehydratases (highly stereospecific enzymes). Decarboxylation Amino acids can be converted into important biological amines by the process of decarboxylation. Example: some neurotransmitters are formed by this reaction (Dopamine, Norepinephrine, Epinephrine). Metabolism of ammonia At the physiological pH, ammonia exists as NH4+ ions. Formation of ammonia: 1. Amino acids by Trans-deamination 2. Biogenic amines 3. Pyrimidine catabolism 4. By action of intestinal bacteria on urea Ammonia is produced in most tissues. Since ammonia is extremely toxic, for the ultimate conversion of ammonia to urea, it is transported to the liver. It is immediately converted to non-toxic metabolites such as: - Glutamate (all tissues) → α-ketoglutarate → TCA cycle - Glutamine (Brain) → α-ketoglutarate → TCA cycle - Alanine (Muscle) → pyruvate→ TCA cycle - Aspartate → oxaloacetate → TCA cycle - Ultimately urea Transport of ammonia In humans, two mechanisms are available for the transport of ammonia from the peripheral tissues to the liver for it to be converted into urea: 1. Transport of Ammonia in the form of glutamine. 2. Transport of ammonia of the form in Alanine. Transport of Ammonia in the form of glutamine Glutamine serves a storage and transport form of NH3. It is present at the highest concentration in blood among the amino acids In many tissues (liver, kidney, and brain), ammonia is enzymatically combined with glutamate to yield glutamine by the action of glutamine synthetase. The glutamine, so formed is a neutral non-toxic major transport form of ammonia. The glutamine is transported by the blood to the liver, where it is cleaved by glutaminase to yield glutamate and free ammonia. In the liver: The ammonia so formed is converted by the liver into urea. Transport of Ammonia in the form of Alanine Alanine plays a special role in transporting amino groups to the liver in a non-toxic form by the glucose-alanine cycle. Alanine passes into the blood and is carried to the liver. Skeletal muscles produce pyruvate, lactate, and ammonia – which can be transported to the liver by alanine. Vigorously contracting skeletal muscles operate anaerobically and produce not only ammonia from protein degradation but also large amounts of pyruvate from glycolysis. These find their way to the liver for its conversion into urea for excretion and pyruvate for its incorporation into glucose and subsequent return to the muscles. The animals thus solve two problems with one cycle (glucose-alanine cycle): 1.They move the carbon atoms of pyruvate, as well as excess ammonia from muscle to the liver as alanine. 2.In the liver, alanine yields pyruvate, the starting material for gluconeogenesis and releases NH4+ for urea synthesis. Nitrogen Excretion Amino nitrogen is excreted in 3 forms: 1. Ammonia- is most aquatic vertebrates (bony fishes) and amphibian larvae (ammonotelic animals) 2. Urea- in terrestrial vertebrates including man, also sharks and adult amphibians (ureotelic animals). 3. Uric acid- in reptiles and birds (urecotelic animals). Plants recycle all amino groups. Nitrogen excretion occurs only under highly unusual circumstances. There is no general pathway for nitrogen excretion in plants. The Urea Cycle [Krebs – Hanseleit Urea Cycle] In ureotelic organisms, the ammonia in the mitochondria of liver cells (hepatocytes) is converted to urea via the urea cycle. The urea cycle is the first metabolic pathway to be elucidated. It was discovered in 1932 by Hans Adolf Krebs and Kurt Henseleit. SIGNIFICANCE: Toxic ammonia is converted into non-toxic urea. Synthesis of semi-essential amino acids and polyamines. The Urea Cycle Note that ornithine and citrulline can serve as successive precursors of arginine. Ornithine and citrulline are nonstandard amino acids that are not found in proteins. Steps in the urea cycle – 5 enzymatic steps STEP 1: Carbamoyl phosphate synthetase I: Captures free ammonia in the mitochondrial matrix First step of the urea cycle, Regulated Ammonia is recaptured via the synthesis of carbamoyl phosphate – the first nitrogen-acquiring reaction in the urea cycle. ENTERS UREA CYCLE STEP 2: Carbamoyl donates the carbamoyl group to citrulline and releases inorganic phosphate. The citrulline is released from the mitochondrion into the cytosol. STEP 3: - This is the second nitrogen-requiring reaction. - The second amino group to create urea is donated by aspartate. STEP 4: - These two reactions, which transfer the amino group of aspartate to form arginine, preserve the carbon skeleton of aspartate in the form of fumarate. STEP 5: The “Krebs Bicycle” The citric acid cycle and urea cycle both are linked together. The fumarate produced in the cytosol by the argininosuccinate lyase reaction is also an intermediate of the citric acid cycle. Fumarate enters the citric acid cycle in the mitochondrion where it is first hydrated to malate by fumarase which, in turn, is oxidized to oxaloacetate by malate dehydrogenase The “Krebs Bicycle” The oxaloacetate accepts an amino group from glutamate by transamination forming aspartate. The aspartate thus formed leaves the mitochondrion and donates its amino group to the urea cycle in the argininosuccinate synthetase reaction. The other product of this transamination is α-ketoglutarate, another intermediate of the citric acid cycle. Because the reactions of the urea and citric acid cycles are inextricably intertwined, cumulatively they have been called “Krebs bicycle”. The Krebs Bicycle Regulation of Urea Cycle Carbamoyl phosphate synthase (CPS-1) is the rate-limiting enzyme in the urea cycle. CPS-1 allosterically activated by N-acetyl glutamate (NAG) (unique enzyme cofactor, essential for liver ureagenesis and arginine biosynthesis) The rate of urea synthesis in the liver is correlated with the concentration of N-acetyl glutamate. Ala Arg His Asn Cys lle Asp Gln Leu Glu Gly Lys Ser Pro Met Try Phe Thr Trp Val END OF LECTURE 6