Lecture 6 - Physiology PDF
Document Details
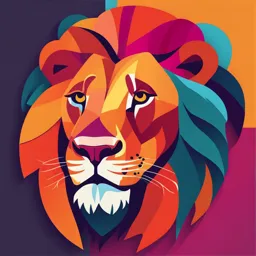
Uploaded by WonMaple
İstanbul Medipol University
Mehmet OZANSOY
Tags
Related
- Action Potential Reading Material Physiology PDF
- General Physiology - Neurons and Action Potential PDF
- L8 Graded Potential & Action Potential (PHYSIOLOGY) PDF
- Action Potential in Physiology - Detailed Notes PDF
- Nervous System: Neurons and Action Potentials PDF
- University of Galway Physiology Lecture 3 on Action Potentials 2024-2025 PDF
Summary
This document is a lecture on bioelectrical potentials and action potentials. It details the processes involved in action potentials, including the role of sodium and potassium channels. The lecture is from Istanbul Medipol University.
Full Transcript
BIOELECTRICAL POTENTIALS-II Action Potential Assist. Prof. Dr. Mehmet OZANSOY, Ph.D. İstanbul Medipol University, International School of Medicine, Dept. of Physiology Action Potential...
BIOELECTRICAL POTENTIALS-II Action Potential Assist. Prof. Dr. Mehmet OZANSOY, Ph.D. İstanbul Medipol University, International School of Medicine, Dept. of Physiology Action Potential An AP is a rapid change in the membrane potential during which the membrane rapidly depolarizes and repolarizes. 1 3 2 4 Voltage-Gated Sodium Channel Activation and Inactivation of the Channel ) Resting membrane potential Voltage-Gated Sodium Channel Activation and Inactivation of the Channel The inactivation gate do not reopen until the membrane potential returns to or near the original resting membrane potential level ! Voltage-Gated Potassium Channel and Its Activation Resting membrane potential Voltage-Gated Sodium and Potassium Channels The channel gates are closed at the resting membrane potential of -70 mV and open in response to depolarization of the membrane to a threshold value. Na channels respond much faster to changes in membrane voltage. Depolarization of the membrane causes Na+ channels to rapidly open, then undergo inactivation followed by the opening of K+ channels. When an area of a membrane is suddenly depolarized, local Na channels open before the K channels do, and if the membrane is then repolarized to negative voltages, the K+ channels are also slower to close. (Hyperpolarization) Na channels have an extra feature in their structure known as an inactivation gate. The inactivated channel cannot be opened by depolarization. The inactivation ends when the membrane potential returns to or near the resting membrane potential level. INITIATION OF THE ACTION POTENTIAL Steps of Action Potential Na+-K+ ATPase ! All-or-None Law Stimulus is weaker than required to reach threshold, and no action potentials are produced. When the membrane potential reaches threshold, action potentials are generated. Increasing the stimulus strength above threshold level does not cause larger action potentials. The stimulus strength is coded by the frequency (rather than the amplitude) of action potentials. Absolute & Relative Refractory Periods Absolute Refractory Period If a second stimulus is applied during most of the time that an action potential is being produced, the second stimulus will have no effect on the axon membrane. The membrane is thus said to be in an absolute refractory period; it cannot respond to any subsequent stimulus (no matter how strong). Relative Refractory Period During the time that the Na+ channels are in the process of recovering from their inactivated state and the K+ channels are still open (the membrane is still in the process of repolarizing), the membrane is in a relative refractory period. Only a very strong depolarization stimulus will be able to produce a second action potential. Action Potential Propagation Saltatory Conduction in Myelinated Fibers The myelin sheath is an excellent electrical insulator that decreases ion flow through the membrane. Conduction in an Myelinated Axon Conduction in an Myelinated Axon Propagation via saltatory conduction is faster than propagation in nonmyelinated fibers of the same axon diameter. This is because less charge leaks out through the myelin covered sections of the membrane, more charge arrives at the node adjacent to the active node, and an action potential is generated there sooner than if the myelin were not present. Action potentials occur only at the nodes of Ranvier, where the myelin coating is interrupted and the concentration of voltage-gated Na channels is high. Myelinated axons are generally thicker than unmyelinated axons, and so have less resistance to the spread of charges Saltatory conduction is of value for two reasons: 1. First, by causing the depolarization process to jump long intervals along the axis of the nerve fiber, this mechanism increases the velocity of nerve transmission in myelinated fibers as much as 5-to 50-fold. 2. Second, saltatory conduction conserves energy for the axon because only the nodes depolarize, allowing perhaps 100 times less loss of ions than would otherwise be necessary, and therefore requiring much less energy expenditure for re-establishing the sodium and potassium concentration differences across the membrane after a series of nerve impulses. Inhibition of Excitability — Stabilizers and Local Anesthetics Procaine, tetracaine, lidocaine. Local anesthetics reversibly bind to voltage gated sodium channels in the axon membrane, preventing them from opening to produce a depolarization. PLATEAU IN SOME ACTION POTENTIALS * Voltage-gated calcium-sodium channels Heart muscle fibers RHYTHMICITY OF SOME EXCITABLE TISSUES — REPETITIVE DISCHARGE Rhythmical beat of the heart, rhythmical peristalsis of the intestines, rhythmical control of breathing.