Bio 224.3 - Animal Body Systems Lecture 7: Electrochemical Potentials in Neurons
Document Details
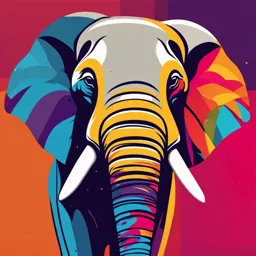
Uploaded by ChivalrousMossAgate1187
University of Saskatchewan
Dr Joan Forder
Tags
Summary
These lecture notes cover the electrochemical potentials in neurons. The lecture, part of a larger course on animal body systems, includes supplementary reading from a textbook.
Full Transcript
Biol 224.3 – Animal Body Systems Lecture 7: Electrochemical Potentials in Neurons Dr Joan Forder Supplementary Reading: Textbook (5th Edition, Chapter 42, page 1123-1137) 1 Biol...
Biol 224.3 – Animal Body Systems Lecture 7: Electrochemical Potentials in Neurons Dr Joan Forder Supplementary Reading: Textbook (5th Edition, Chapter 42, page 1123-1137) 1 Biol 224.3 – Animal Body Systems Lecture 7: Electrochemical Potentials in Neurons Dr Joan Forder Supplementary Reading: Textbook (5th Edition, Chapter 42, page 1123-1137) 1 Review General Concepts Organ systems must be coordinated: - within an animal - with the environment Two major systems involved: - nervous system - endocrine system Both act together - allows for complex homeostatic control 2 Nervous Systems In all animals except sponges A very rapid coordination/regulation system Has three major roles: 1. Collects information from internal or external environment using modified neurons (sensory receptors) 2. Process & integrate information more rapid know what to do (already ↑ evaluates based on past experience and/or genetics 3. Transmit information coordinates/regulates effector organ/cells 3 Terminology Neuron: individual cell Nerve: a bundle of axons (a few to millions) - NOT entire neuron Axon: also called a nerve fiber Synapse: connection between axon terminal & effector cell space - > synaptic cleft Effector: can be a neuron, muscle cell, any other cell eX. - > GLANDS 4 Bioelectricity - it all happens at the membrane! Electrical Terminology Source: https://sciencebusiness.technewslit.com/?p=32388 Potential: difference in electrical charge between regions from - measured in volts (V) or millivolts (mV) difference to outside inside Current: flow of electrical charge between regions - opposites attract, like repels specific to cell. Membrane potential: unequal charge distribution across a cell membrane 5 Cells are Polarized All living cells are electrically have a membrane polarized potential (MP) inside of membrane is negative relative to exterior side size of MP ranges from -10 to -90 mV ↑ milli-volts. 6 Excitable Cells - higher difference Neurons (& muscle cells) are specially adapted. They have: Large membrane potentials higher >-10 Special mechanisms to regulate membrane potentials and currents Three types of membrane potentials: 1. Resting membrane potentials (RMP) 2. Electrotonic potentials (EP) N Or electrical to regulate need cell 3. Action potentials (AP) ma action for Membrane potentials & currents depend on inorganic ions. 7 Resting Membrane Potential of a Cell All cells have a resting membrane potential Measured when the cell is inactive OUTSIDE The membrane potential of a cell results - 70 mV from the unequal distribution of positive and negative charges on either side of the membrane. This establishes a potential difference, the resting potential, across the membrane. INSIDE Axon of Neuron principle ions involved are Na+ and K+ to measure - & cl Charge different CC Ion Concentrations in Cells a nions inside bla they re too bis to leave => - CHARGE INSIDE Extracellular fluid always has.. Intracellular fluid always has.. high [Na+] high [K+] low [K+] low [Na+] How ? embedded protein that can be controlled * (sodium-potassium pump) Sodium outside cells; potassium inside cells canions) 9 Ion Gradients in all Cells ion gradients maintained by active transport - > energy required Na+ / K+ ATPase moves 3 [Na+] out, 2 [K+] in OUTSIDE is an electrogenic pump - > generates potential generates a -10 mV potential all cells anionic proteins generate a -5 mV potential LEAST-IS mV potential # INSIDE in all cells. 10 The Additional Potential in Neurons What about the other -55 mV in neurons/muscle? by passive diffusion of K+ through an open K+ channel Chemical gradient for K+ - no electrical gradient ATPase and the leak channels together create an electrochemical gradient 11 Resting Potential of Neurons voltage controlled-gated very low channel RMP permeability AP AP Na+/K+ active transport pump Sets up concentration gradients of Na+ ions (higher outside) and K+ ions (higher inside) Open channel (leak channel) allows K+ to flow out freely Negatively charged molecules (proteins) inside cell can’t pass through membrane ANIONS -10 mV + -5 mV + -55 mV = - 70 mV - Standard pump + ATPaSe Membrane Ion Channels are very specific for each ion leak channels are always open Yet still very specific - HOW ? e.g. the K+ channel at rest Shape & · Size other channels are regulated (gated) differences has to change in molecules to open. (controlled) shape ↓ in neurons, channels are often voltage- so protiens shoped gated are accordingly. ion movement depends on the concentration gradient once opened. closed-gradient doesn't matter 13 Polarization in Cells The cell is now polarized (negative inside) But cells can become depolarized (more +ve inside) (positive) … or hyperpolarized (more -ve inside) (negative) Happens during electrotonic potentials (EP) or the action potential (AP) EP: small changes in membrane potentials AP: large & rapid changes in membrane potentials less & back t G & BASELINE more ② Fig 42.6 14 Graded Potentials Changes in membrane potential due to changes in membrane permeability to ions are called graded potentials. - channels - open con de- / hyper-polarize. In neurons, graded potentials are part of the integration that takes place in dendrites and cell bodies. Electrotonic Potentials are one type of graded potential not enough to Cause ACTION potential. Electrotonic Potentials current (ions) travels along surface of membrane small (only a few mV) can depolarize or hyperpolarize only travel a short distance along membrane - used to initiate an action potential (AP) in axon hillock - also to conduct AP along axon 16 period after Action Potentials max - peak is reached. > - same region initiated at axon hillock region cannot gered if in be trig- refractory found only in axons period. PASS carries the signal from axon hillock to threshold terminals potential - > opens Special features of APs: gates - depolarizes membrane (from -70 to +35 mV) - turnspositive ; rapidly BUT briefly. - are all or nothing but transient - once started, conducted along entire axon - rely on ion currents through membrane via voltage-gated ion channels Fig 42.7 17 Generation of an Action Potential Generated when stimulus pushes resting potential to threshold value Voltage-gated Na+ and K+ channels open in the plasma membrane Inward flow of Na+ changes membrane potential from negative to positive peak Potential falls to resting value as gated K+ channels allow ion to flow out Depolarization Rising Phase of AP AP depends on ion currents & voltage- gated channels channels Na+v = voltage gated sodium channel Fig 42.8 At t=0 Na+v & K+v Na+v channel opens Na+v channel closes K+v = voltage gated Channels closed (EP). Na+ flows in. and inactivates. potassium channel Stimulus opens them. depolarization depolarized peak depolarization NOTE: K+ leak channels are always open. 19 The Rest of the AP Falling Phase of AP AP depends on ion currents & voltage- gated channels Fig 42.8 K+v channel opens K+v channel still K+v channel closes K+ flows out. open at RMP Na+v still inactivated K+ still flows out hyperpolarization repolarization (briefly) Na+/K+ pump returns RMP concentration gradient Refractory period NOTE: K+ leak channels are always open. 20 The Hodgkin-Huxley Cycle AP rise phase is Positive Feedback Initial depolarization Further Opening of Na+v membrane channels increases depolarization permeability to Na+ Increased Na+ flow 21 AP Propagation Along Axon AP initiated in axon hillock (concentration of Na+v Conducted unchanged along axon membrane channels) to terminals Dendrites & cell body – concentration of K+v channels reduces backpropagation into soma 22 Propagation of Action Potential Action potentials move along an axon as the ion flows generated in one segment depolarize the potential in the next segment. Will look at this for both Unmyelinated axons and Myelinated axons AP Conduction in UNMYELINATED Axon Fig 42.9 Reduced threshold at axon hillock (spike initiating zone) Concentration of Na+v channels Current spreads along membrane toward terminals (new AP) 24 AP Conduction in UNMYELINATED Axon Fig 42.9 Adjacent (downstream) Na+v channels reach threshold - from large depolarization (new AP) Refractory period prevents backpropagation 25 AP Conduction in UNMYELINATED Axon Fig 42.9 Next adjacent (downstream) Na+v channels reach threshold etc. Axon diameter determines speed of conduction (larger = faster) – up to 40 m/s Typical of most invertebrates 26 Saltatory Conduction In myelinated axons, ions can flow across the plasma membrane only at nodes where the myelin sheath is interrupted. Action potentials skip rapidly from node to node. Saltatory conduction allows thousands to millions of fast- transmitting axons to be packed into a relatively small diameter. AP Conduction in MYELINATED Axon Fig 42.10 Myelin (protein and lipid) insulation prevents ions from crossing the membrane – reduces current loss Concentration of Na+v and K+v at nodes – allows ions to cross membrane 28 AP Conduction in MYELINATED Axon Fig 42.10 29 AP Conduction in MYELINATED Axon Fig 42.10 Axon hillock similar to unmyelinated neuron Similar conduction process but current spreads quickly between nodes 30 AP Conduction in MYELINATED Axon Fig 42.10 Saltatory (jumping) conduction from node to node to reach terminals Higher conduction velocities (up to 100 m/s) – Vertebrates 31 Something to Think About Why might vertebrates require higher action potential conduction velocities? 32