Lecture 5.pptx
Document Details
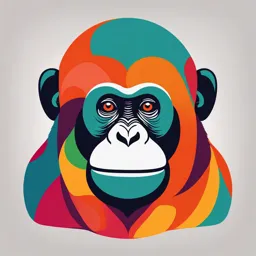
Uploaded by ChampionPyrite
King Khalid University
Full Transcript
Chapter 5 Fractionated Radiation and the Dose-Rate Effect 1 OPERATIONAL CLASSIFICATIONS OF RADIATION • Radiation Damage Categories: 1. 2. 3. DAMAGE Lethal Damage: Irreversible, leads to cell death. Potentially Lethal Damage (PLD): Modifiable by postirradiation conditions. Sublethal Damage (SLD)...
Chapter 5 Fractionated Radiation and the Dose-Rate Effect 1 OPERATIONAL CLASSIFICATIONS OF RADIATION • Radiation Damage Categories: 1. 2. 3. DAMAGE Lethal Damage: Irreversible, leads to cell death. Potentially Lethal Damage (PLD): Modifiable by postirradiation conditions. Sublethal Damage (SLD): Repairable under normal circumstances, may form lethal damage with additional exposure. • Potentially Lethal Damage (PLD) • PLD is potentially lethal; it causes cell death under ordinary circumstances. • PLD repair can occur through varying postirradiation environmental conditions. • PLD repair was studied in density-inhibited stationary-phase cell cultures, a model for in vivo tumor cells. • PLD Repair In Vitro • PLD repair in vitro: Enhanced cell survival observed when cells remained in density-inhibited state for 6 or 12 hours after irradiation. • Drastic treatments, like incubation in a balanced salt solution, aid PLD repair in vitro. • PLD Repair In Vivo • PLD repair in vivo: Enhanced cell survival observed in experimental tumors if time interval allowed between irradiation and removal. • In vivo repair demonstrated in mouse fibrosarcomas. • Relevance to Radiotherapy • PLD repair is crucial in radiotherapy, influencing the fraction of surviving cells after irradiation. • The radioresistance of some human tumors might be linked to their ability to repair PLD. • Mitosis Delay and DNA Repair: • Suboptimal growth conditions delay mitosis, allowing DNA damage repair, contributing to PLD repair. 2 OPERATIONAL CLASSIFICATIONS OF RADIATION Sublethal Damage Repair (SLD) • DAMAGE Definition: Sublethal Damage (SLD) repair refers to the increase in cell survival observed when a given radiation dose is split into two fractions separated by a time interval. • Experimental Evidence of Sublethal Damage Repair o Experimental Setup and Data (Figure 5.3) • Experiment conducted with cultured Chinese hamster cells exposed to split doses of radiation. • Initial dose of 15.58 Gy led to a surviving fraction of 0.005. • When the dose was divided into two fractions with a 30-minute interval, the surviving fraction increased significantly. • Extended time intervals led to further increase until a plateau at about 2 hours (surviving fraction of 0.02), indicating repair of sublethal radiation damage. o Temperature-Dependent Repair (Figure 5.4) • The data shown in Figure 5.3 were obtained with cultured mammalian cells maintained at room temperature (24° C) between the dose fractions to prevent the cells from moving through the cell cycle during this interval. • While Cells maintained at normal growing temperature (37°C) showed a different 3 pattern of repair. o Prompt repair of SLD observed in the first few hours, but longer intervals resulted in decreased surviving fraction due to cell cycle dynamics. OPERATIONAL CLASSIFICATIONS OF RADIATION DAMAGE Sublethal Damage Repair (SLD) ( cont.) • Mechanisms of Sublethal Damage Repair o Factors Influencing Repair • Repair of SLD involves three simultaneous processes: 1. prompt repair, 2, reassortment (cell cycle progression), and 3. repopulation (cell division) (exceeds the length of the cell cycle). o SDL Repair Mechanisms and survival curve • SLD repair is manifested by the increase in cell survival in split-dose experiments. • The extent of repair correlates with the size of the shoulder in the survival curve. o Time Course of Repair (Figure 5.6) • As the time interval between dose fractions increases, there is a rapid increase in cell survival due to prompt repair of SLD. • Cell cycle dynamics may cause a dip in survival in rapidly dividing cells, but 4 there is an increase in survival owing to cell proliferation if the interval exceeds the cell cycle length. OPERATIONAL CLASSIFICATIONS OF RADIATION Dose Fractionation with X-rays DAMAGE • X-ray Fractionation: Dividing the total dose into two equal fractions (4+4 Gy) with a time gap of 1 to 4 hours. • Effect on Cell Survival: X-rays prompt repair of SLD, leading to a significant increase in cell survival when the dose is fractionated. Dose Fractionation with Neutrons • Neutron Fractionation: Dividing the dose into two fractions (1.4 +1.4 Gy) with a time gap. • Limited Repair: Neutrons have little effect on SLD repair; dividing the dose into fractions doesn't significantly impact cell survival. Figure 5.7 : Show the acute survival curve for x-rays and neutrons with and without dose fractionation. 5 THE DOSE-RATE EFFECT The Dose-Rate Effect o Dose rate is a critical factor influencing the biological consequences of absorbed doses from x- or γ-rays. o Lower dose rates and extended exposure times generally result in reduced biological effects and increase the survival Classic Dose-Rate Effect: • Occurs during long radiation exposure. • Results from the repair of Sublethal Damage (SLD). • Experiment illustration: Dose delivered in equal fractions (D2, D3, D4, etc.) with sufficient time intervals for SLD repair. • Survival curve demonstrates the effect: shoulder appears due to repeated shoulders in each fraction. ________________________________________ ________________________________________ Importance in Radiotherapy • Understanding dose-rate effect crucial in radiotherapy planning. • Enhances patient outcomes: tailoring radiation delivery to maximize tumor control while minimizing damage to healthy tissues 6 EXAMPLES OF THE DOSE-RATE EFFECT IN VITRO • Dose-Rate Effect in HeLa Cells • • • AND IN VIVO Detailing the reduction of the survival curve's shoulder as dose rate decreases. Emphasizing the exponential function of the survival curve concerning dose. Highlighting the repair of Sublethal Radiation Damage (SLD) and its effect on the curve. • Variability in Dose-Rate Effects • • • Comparing the dose-rate effects among different cell types. Contrasting HeLa cells' modest dose-rate effect with Chinese hamster cells' significant effect. Linking differences to the importance of apoptosis in cell death following radiation. • Variation in Human Cell Lines • • • • Introducing survival curves for 40 different human cell lines. Discussing the fanning out of survival curves at Low Dose Rates (LDR) due to varied radiosensitivity and repair times of SLD. Exploring the impact of repair times on survival curves at LDR. Dose-Rate Effect in Mouse Jejunum • • • Presenting survival curves for crypt cells in mouse jejunum irradiated with γ-rays at various dose rates. Discussing the dramatic dose-rate effect and its correlation with the repair of sublethal radiation damage. Explaining the dominance of cell division in reducing the number of surviving crypts at lower dose rates. 7 EXAMPLES OF THE DOSE-RATE EFFECT IN VITRO AND IN VIVO • Survival Curves and Dose Rates in Cell Cultures • • • Definition: Survival curves show cell survival rates after exposure to radiation. Variation in Dose Rates: Study involves a wide range of dose rates, from 7.3 Gy/minute to 0.00535 Gy/minute. As shown in the figures, when dose rate decreases, survival curves become shallower; the shoulder tends to disappear, indicating an exponential relationship between dose and survival. • Dose-Rate Effect and Repair of Sublethal Damage (SLD) • • • • • FIGURE 5.9 Survival curves for HeLa cells cultured in vitro and exposed to γrays at high and low dose rates. Dose-Rate Effect Range: Most significant between 0.01 and 1 Gy/minute. Repair of SLD: Dose-rate effect caused by repair of SLD. Cell Variability: Magnitude of dose-rate effect varies significantly among different cell types. Examples: HeLa cells show a moderate change in response to decreasing dose-rate due to their low capacity to repair sublethal radiation damage. In contrast, Chinese hamster cells exhibit a large dose-rate effect with a broad shoulder and high capacity to repair sublethal radiation damage . Importance of Apoptosis in Dose-Rate Effect • • Apoptosis: HeLa cells undergo apoptosis as a significant form of cell death postradiation exposure, whereas for hamster cells, apoptotic death is rarely seen. Cellular Response: Differences in the size of the shoulder due to the acute survival curve and dose-rate effect reflect variations in the importance of apoptosis among different cell types. 8 FIGURE 5.10 Dose–response curves for Chinese hamster cells (CHL-F line) grown in vitro and exposed to cobalt-60 γ-rays at various dose rates. EXAMPLES OF THE DOSE-RATE EFFECT IN VITRO AND IN VIVO • Variation in Human Cell Lines and Repair Times • Human Cell Lines: Survival curves for 40 different human cell lines cultured in vitro show a greater variation in slope at low dose rates (LDR). In contrast, high dose rates (HDR) survival curves show smaller variation in slope. • Repair Times: Differences in inherent radiosensitivity and repair times of SLD contribute to the significant differences in survival curves at low dose rates (LDR) • Dose-Rate Effect in Mouse Jejunum Crypt Cells • • Effect of Dose Rates: Dramatic dose-rate effect observed in mouse jejunum crypt cells from acute exposure (2.74 Gy/minute) to extended exposure at (0.92 cGy/minute). Cell Division Influence: Lower dose rates (0.54 cGy/minute) result in limited reduction in surviving crypts due to cellular proliferation occurs during the long exposure compensating for radiation-induced cell killing. 9 THE INVERSE DOSE-RATE EFFECT • Inverse Dose-Rate Effect in HeLa Cells o The decreasing dose rate from 1.54 to 0.37 Gy per hour leads to an increase in the efficiency of cell killing. • Low Dose Rate vs. Acute Exposure • o Low Dose Rate (LDR) at 0.37 Gy per hour is almost as damaging as acute exposure. Understanding the Inverse Dose-Rate Effect o At higher dose rates (1.54 Gy/hr), cells are “frozen” in different phases of the cycle (G1,S and M) they are in at the start of the irradiation. o While, at low dose rate( 0.37 Gy per hour), cells progress through the cycle and become arrested in G2 phase (a radiosensitive phase). o A further lowering of dose rate below the critical dose rate allows cells to escape the G2 block and divide; cells continue to proliferation. (Figure 5.14): Illustration of cell cycle progression at different dose rates. 1 0 BRACHYTHERAPY OR ENDOCURIETHERAPY • Historical Context: o Alexander Graham Bell's 1901 proposal for implanting radioactive sources in tumors. o Evolution of brachytherapy terminology: "brachy" from Greek for "short range" and "endo" for "within." • Types of Brachytherapy A. Intracavitary Brachytherapy: • It defined as the placement of radioactive sources in body cavities near the tumor. • Examples: Early use of radium and cesium-137; modern use of iridium-192. • Transition from Low Dose Rate (LDR) to High Dose Rate (HDR) intracavitary therapy for specific cases, with focus on cervical carcinoma. o Intracavitary Brachytherapy Techniques • LDR Intracavitary Brachytherapy: o Temporary procedure lasting 1 to 4 days. (with a dose rate of about 50 cGy per hour) (uterine cervix) • Transition to HDR Intracavitary Therapy: Delivered in 3 to 12 dose fractions, overcoming radiobiologic 1 challenges. 1 o Advantages of HDR techniques : Reduced normal tissue exposure for specific cases like cervical carcinoma. BRACHYTHERAPY OR ENDOCURIETHERAPY • Types of Brachytherapy (cont. ) B. Permanent Interstitial Implants: o It is defied as the encapsulated sources with relatively short half-lives can be left in the tumor permanently. o Advantages of Permanent Interstitial Implants: (1) An operation to remove the implant is not needed. (2) The patient can go home with the implant in place. o Common use of iodine-125 with a total prescribed dose of 160 Gy, delivering 80 Gy in the first 60 days. (carcinoma of the prostate) o Advantages of Iodine-125: 1. Low-energy photon emission (about 30 keV) for effective tumor treatment. 2. Rapid dose fall-off outside the treatment volume, reducing exposure to remote body parts. 1 2 • • • • • Summary of Fractionated Radiation and the Dose-Rate Effect Potentially Lethal Damage Repair (PLD): • Definition: Radiation damage modification post-irradiation is known as PLD. • Conditions: PLD repair occurs if cells are prevented from dividing for 6 hours or more after irradiation. • Demonstration: In vitro, cells are kept in saline or plateau phase for 6 hours; in vivo, delayed removal and assay show PLD repair. • Significance: Significant for x-rays, absent after neutron irradiation. • Controversy: Suggested in resistant tumors (e.g., melanoma), still debated. Sublethal Damage Repair (SLD): • Definition: Increase in survival if radiation dose is split into two fractions separated in time. • Timing: Half-time of SLD repair in mammalian cells is about 1 hour, potentially longer in late-responding normal tissues. • Occurrence: Evident in tumors, normal tissues in vivo, and cultured cells in vitro. • Mechanism: Reflects repair of DNA breaks before forming lethal chromosomal aberrations. • Radiation Types: Significant for x-rays, nearly nonexistent for neutrons. Dose-Rate Effect: • Effect: Reduction in dose rate (e.g., from 1 Gy/minute to 0.3 Gy/hour) leads to reduced cell killing due to SLD repair during prolonged exposure. • Survival Curve: Shallower slope (increased D0) and disappearance of the shoulder with reduced dose rates. • Inverse Effect: Some cell lines show an inverse dose-rate effect, where reducing the dose rate increases cell killing, due to cell accumulation in G2 phase. Brachytherapy Techniques: • • Definition: Implanting radioactive sources into or close to tumors. Types: • • • Intracavitary Brachytherapy: Sources placed in body cavities near tumors (e.g., uterine cervix). • LDR: Lasts 1 to 4 days, being replaced by HDR (3 to 12 dose fractions). 1 3 Interstitial Brachytherapy: Implanting sources directly into tumors and adjacent tissues (7 to 10 days). Permanent Implants: Use radionuclides with short half-lives (e.g., iodine-125) or novel sources. Radiation Protection: Low-energy photon emissions simplify radiation protection issues.