LECTURE 5.docx
Document Details
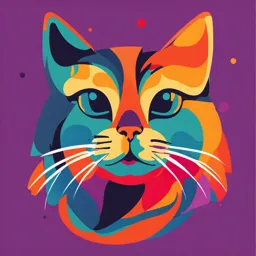
Uploaded by UsefulPrologue5110
Tags
Full Transcript
**INTRODUCTION** Every day 180 L of glomerular filtrate is formed with large quantity of water. If this much of water is excreted in urine, body will face serious threats. So the concentration of urine is very essential. Osmolarity of glomerular filtrate is same as that of plasma and it is 300 mOs...
**INTRODUCTION** Every day 180 L of glomerular filtrate is formed with large quantity of water. If this much of water is excreted in urine, body will face serious threats. So the concentration of urine is very essential. Osmolarity of glomerular filtrate is same as that of plasma and it is 300 mOsm/L. But, normally urine is concentrated and its osmolarity is four times more than that of plasma, i.e. 1,200 mOsm/L. Osmolarity of urine depends upon two factors: 1\. Water content in the body 2\. Antidiuretic hormone (ADH). **MECHANISM OF URINE FORMATION** Mechanism of urine formation is the same for dilute urine and concentrated urine till the fluid reaches the distal convoluted tubule. However, dilution or concentration of urine depends upon water content of the body. Figure 1: Water diuresis in a human after ingestion of 1 liter of water. Note that after water ingestion, urine volume increases and urine osmolarity decreases, causing the excretion of a large volume of dilute urine; however, the total amount of solute excreted by the kidneys remains relatively constant. These responses of the kidneys prevent plasma osmolarity from decreasing markedly during excess water ingestion. Image Credit: Guyton & Hall, Textbook of Medical Physiology, 12^th^ Edition. The maximal concentrating ability of the kidney dictates how much urine volume must be excreted each day to rid the body of waste products of metabolism and ions that are ingested. A normal 70-kilogram human must excrete about 600 milliosmoles of solute each day. If maximal urine concentrating ability is 1200 mOsm/L, the *minimal* volume of urine that must be excreted, called the *obligatory urine volume,* can be calculated as: **[600 mOsm/day]** **1200 mOsm/L** = **0.5L/day** **FORMATION OF DILUTE URINE** When, water content in the body increases, kidney excretes dilute urine. This is achieved by inhibition of ADH secretion from posterior pituitary. So water reabsorption from renal tubules does not take place leading to excretion of large amount of water. This makes the urine dilute. Figure 2: Mechanism for the formation of dilute urine. Numerical indicate osmolarity (mOsm/L) Image Credit: K & Prema Sembulingam, Essentials of medical physiology, 6^th^ Edition. **FORMATION OF CONCENTRATED URINE** When the water content in body decreases, kidney retains water and excretes concentrated urine. Formation of concentrated urine is not as simple as that of dilute urine. It involves two processes: 1\. Development and maintenance of medullary gradient by countercurrent system 2\. Secretion of ADH. **MEDULLARY GRADIENT** **MEDULLARY HYPEROSMOLARITY** Cortical interstitial fluid is isotonic to plasma with the osmolarity of 300 mOsm/L. Osmolarity of medullary interstitial fluid near the cortex is also 300 mOsm/L. However, while proceeding from outer part towards the inner part of medulla, the osmolarity increases gradually and reaches the maximum at the inner most part of medulla near renal sinus. Here, the interstitial fluid is hypertonic with osmolarity of 1,200 mOsm/L. This type of gradual increase in the osmolarity of the medullary interstitial fluid is called the medullary gradient. It plays an important role in the concentration of urine. **DEVELOPMENT AND MAINTENANCE OF MEDULLARY GRADIENT** Kidney has some unique mechanism called countercurrent mechanism, which is responsible for the development and maintenance of medullary gradient and hyperosmolarity of interstitial fluid in the inner medulla. **COUNTERCURRENT MECHANISM** **COUNTERCURRENT FLOW** A countercurrent system is a system of 'U'shaped tubules (tubes) in which, the flow of fluid is in opposite direction in two limbs of the 'U'shaped tubules. ***Divisions of Countercurrent System*** Countercurrent system has two divisions: 1\. Countercurrent multiplier formed by loop of Henle 2\. Countercurrent exchanger formed by vasa recta. **COUNTERCURRENT MULTIPLIER** **Figure 3:** Countercurrent multiplier. Numerical indicate osmolarity (mOsm/L) Image Credit: K & Prema Sembulingam, Essentials of Medical Physiology, 6^th^ Edition. ***Loop of Henle*** Loop of Henle functions as countercurrent multiplier. It is responsible for development of hyperosmolarity of medullary interstitial fluid and medullary gradient. ***Role of Loop of Henle in Development of Medullary Gradient*** Loop of Henle of juxtamedullary nephrons plays a major role as countercurrent multiplier because loop of these nephrons is long and extends upto the deeper parts of medulla. Main reason for the hyperosmolarity of medullary interstitial fluid is the active reabsorption of sodium chloride and other solutes from ascending limb of Henle loop into the medullary interstitium. These solutes accumulate in the medullary interstitium and increase the osmolarity. Now, due to the concentration gradient, the sodium and chlorine ions diffuse from medullary interstitium into the descending limb of Henle loop and reach the ascending limb again via hairpin bend. Thus, the sodium and chlorine ions are repeatedly recirculated between the descending limb and ascending limb of Henle loop through medullary interstitial fluid leaving a small portion to be excreted in the urine. Apart from this there is regular addition of more and more new sodium and chlorine ions into descending limb by constant filtration. Thus, the reabsorption of sodium chloride from ascending limb and addition of new sodium chlorine ions into the filtrate increase or multiply the osmolarity of medullary interstitial fluid and medullary gradient. Hence, it is called countercurrent multiplier. ***Other Factors Responsible for Hyperosmolarity of Medullary Interstitial Fluid*** In addition to countercurrent multiplier action provided by the loop of Henle, two more factors are involved in hyperosmolarity of medullary interstitial fluid. i\. *Reabsorption of sodium from collecting duct* Reabsorption of sodium from medullary part of collecting duct into the medullary interstitium, adds to the osmolarity of inner medulla. ii\. *Recirculation of urea*  Figure 4: Recirculation of urea absorbed from the medullary collecting duct into the interstitial fluid. (Numerical values are in milliosmoles per liter of urea during antidiuresis, when large amounts of antidiuretic hormone are present. Percentages of the filtered load of urea that remain in the tubules are indicated in the blue boxes.) Image Credit: Guyton and Hall, Textbook of Medical Physiology, 12^th^ Edition. Fifty percent of urea filtered in glomeruli is reabsorbed in proximal convoluted tubule. Almost an equal amount of urea is secreted in the loop of Henle. So the fluid in distal convoluted tubule has as much urea as amount filtered. Collecting duct is impermeable to urea. However, due to the water reabsorption from distal convoluted tubule and collecting duct in the presence of ADH, urea concentration increases in collecting duct. Now due to concentration gradient, urea diffuses from inner medullary part of collecting duct into medullary interstitium. Due to continuous diffusion, the concentration of urea increases in the inner medulla resulting in hyperosmolarity of interstitium in inner medulla. Again, by concentration gradient, urea enters the ascending limb. From here, it passes through distal convoluted tubule and reaches the collecting duct. Urea enters the medullary interstitium from collecting duct. By this way urea **recirculates** repeatedly and helps to maintain the hyperosmolarity of inner medullary interstitium. Only a small amount of urea is excreted in urine. Urea recirculation accounts for 50% of hyperosmolarity in inner medulla. Diffusion of urea from collecting duct into medullary interstitium is carried out by **urea transporters,** UTA1 and UTA3, which are activated by ADH. The passive secretion of urea into the thin loops of Henle is facilitated by the urea transporter *UT-A2.* **COUNTERCURRENT EXCHANGER** ***Vasa Recta*** Vasa recta functions as countercurrent exchanger. It is responsible for the maintenance of medullary gradient, which is developed by countercurrent multiplier. Figure 5: Countercurrent exchanger. Numerical indicate osmolarity (mOsm/L) Image Credit: K & Prema SEmbulingam, Essentials of Medical Physiology, 6^th^ Edition ***Role of Vasa Recta in the Maintenance of Medullary Gradient*** Vasa recta acts like countercurrent exchanger because of its position. It is also 'U'shaped tubule with a descending limb, hairpin bend and an ascending limb. Vasa recta runs parallel to loop of Henle. Its descending limb runs along the ascending limb of Henle loop and its ascending limb runs along with descending limb of Henle loop. The sodium chloride reabsorbed from ascending limb of Henle loop enters the medullary interstitium. From here it enters the descending limb of vasa recta. Simultaneously water diffuses from descending limb of vasa recta into medullary interstitium. The blood flows very slowly through vasa recta. So, a large quantity of sodium chloride accumulates in descending limb of vasa recta and flows slowly towards ascending limb. By the time the blood reaches the ascending limb of vasa recta, the concentration of sodium chloride increases very much. This causes diffusion of sodium chloride into the medullary interstitium. Simultaneously, water from medullary interstitium enters the ascending limb of vasa recta. And the cycle is repeated. If the vasa recta would be a straight vessel without hairpin arrangement, blood would leave the kidney quickly at renal papillary level. In that case, the blood would remove all the sodium chloride from medullary interstitium and thereby the hyperosmolarity will be decreased. However, this does not happen, since the vasa recta has a hairpin bend. Therefore, when blood passes through the ascending limb of vasa recta, sodium chloride diffuses out of blood and enters the interstitial fluid of medulla and, water diffuses into the blood. Thus, vasa recta retains sodium chloride in the medullary interstitium and removes water from it. So, the hyperosmolarity of medullary interstitium is maintained. The blood passing through the ascending limb of vasa recta may carry very little amount of sodium chloride from the medulla. Recycling of urea also occurs through vasa recta. From medullary interstitium, along with sodium chloride, urea also enters the descending limb of vasa recta. When blood passes through ascending limb of vasa recta, urea diffuses back into the medullary interstitium along with sodium chloride. Thus, sodium chloride and urea are exchanged for water between the ascending and descending limbs of vasa recta, hence this system is called countercurrent exchanger. **ROLE OF ADH** Figure 6: Formation of a concentrated urine when antidiuretic hormone (ADH) levels are high. Note that the fluid leaving the loop of Henle is dilute but becomes concentrated as water is absorbed from the distal tubules and collecting tubules. With high ADH levels, the osmolarity of the urine is about the same as the osmolarity of the renal medullary interstitial fluid in the papilla, which is about 1200 mOsm/L. (Numerical values are in milliosmoles per liter.) **Image Credit: Guyton & Hall, Textbook of Medical Physiology, 12^th^ Edition** Final concentration of urine is achieved by the action of ADH. Normally, the distal convoluted tubule and collecting duct are not permeable to water. But the presence of ADH makes them permeable, resulting in water reabsorption. Water reabsorption induced by ADH is called **facultative reabsorption of water** A large quantity of water is removed from the fluid while passing through distal convoluted tubule and collecting duct. So, the urine becomes hypertonic with an osmolarity of 1,200 mOsm/L **SUMMARY OF URINE CONCENTRATION** When the glomerular filtrate passes through renal tubule, its osmolarity is altered in different segments as described below. **1. BOWMAN CAPSULE** Glomerular filtrate collected at the Bowman capsule is **isotonic to plasma**. This is because it contains all the substances of plasma except proteins. Osmolarity of the filtrate at Bowman capsule is 300 mOsm/L. **2. PROXIMAL CONVOLUTED TUBULE** When the filtrate flows through proximal convoluted tubule, there is active reabsorption of sodium and chloride followed by **obligatory reabsorption of water** So, the osmolarity of fluid remains the same as in the case of Bowman capsule, i.e. 300 mOsm/L. Thus, in proximal convoluted tubules, the fluid is **isotonic to plasma.** **3. THICK DESCENDING SEGMENT** When the fluid passes from proximal convoluted tubule into the thick descending segment, water is reabsorbed from tubule into outer medullary interstitium by means of osmosis. It is due to the increased osmolarity in the medullary interstitium, i.e. outside the thick descending tubule. The osmolarity of the fluid inside this segment is between 450 and 600 mOsm/L. That means the fluid is slightly **hypertonic to plasma.** **4. THIN DESCENDING SEGMENT OF HENLE LOOP** As the thin descending segment of Henle loop passes through the inner medullary interstitium (which is increasingly hypertonic) more water is reabsorbed. This segment is highly permeable to water and so the osmolarity of tubular fluid becomes equal to that of the surrounding medullary interstitium. In the short loops of cortical nephrons, the osmolarity of fluid at the hairpin bend of loop becomes 600 mOsm/L. And, in the long loops of juxtamedullary nephrons, at the hairpin bend, the osmolarity is 1,200 mOsm/L. Thus in this segment the fluid is **hypertonic to plasma.** **5. THIN ASCENDING SEGMENT OF HENLE LOOP** When the thin ascending segment of the loop ascends upwards through the medullary region, osmolarity decreases gradually. Due to concentration gradient, sodium chloride diffuses out of tubular fluid and osmolarity decreases to 400 mOsm/L. The fluid in this segment is slightly **hypertonic to plasma.** **6. THICK ASCENDING SEGMENT** This segment is impermeable to water. But there is active reabsorption of sodium and chloride from this. Reabsorption of sodium decreases the osmolarity of tubular fluid to a greater extent. The osmolarity is between 150 and 200 mOsm/L. The fluid inside becomes **hypotonic to plasma.** **7. DISTAL CONVOLUTED TUBULE AND COLLECTING DUCT** In the presence of ADH, distal convoluted tubule and collecting duct become permeable to water resulting in water reabsorption and final concentration of urine. It is found that in the collecting duct, Principal (P) cells are responsible for ADH induced water reabsorption. Reabsorption of large quantity of water increases the osmolarity to 1,200 mOsm/L. The urine becomes **hypertonic to plasma.** **APPLIED PHYSIOLOGY** **1. *Osmotic Diuresis*** Diuresis is the excretion of large quantity of water through urine. Osmotic diuresis is the diuresis induced by the osmotic effects of solutes like glucose. It is common in **diabetes mellitus**. **2. *Polyuria*** Polyuria is the increased urinary output with frequent voiding. It is common in **diabetes insipidus.** In this disorder, the renal tubules fail to reabsorb water because of ADH deficiency. **3. *Syndrome of Inappropriate Hypersecretion of ADH (SIADH)*** It is a pituitary disorder characterized by hypersecretion of ADH is the SIADH. Excess ADH causes water retention, which decreases osmolarity of ECF. **4. *Nephrogenic Diabetes Insipidus*** Sometimes, ADH secretion is normal but the renal tubules fail to give response to ADH resulting in polyuria. This condition is called nephrogenic diabetes insipidus. **5. *Bartter Syndrome*** Bartter syndrome is a genetic disorder characterized by defect in the thick ascending segment. This causes decreased sodium and water reabsorption resulting in loss of sodium and water through urine.