lecture 5.docx
Document Details
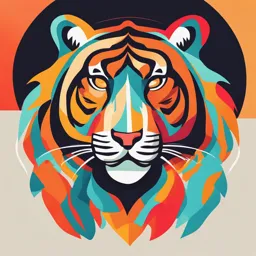
Uploaded by SafeMahoganyObsidian
Full Transcript
Guys hear me? Okay. all right. People actually came amazing. man, that's cruddy weather super. Cruddy weather. so we're going to keep going through. A couple of last slides that were a little bit more review context of some molecular mechanisms. and but hopefully, today will be started going into Ch...
Guys hear me? Okay. all right. People actually came amazing. man, that's cruddy weather super. Cruddy weather. so we're going to keep going through. A couple of last slides that were a little bit more review context of some molecular mechanisms. and but hopefully, today will be started going into Chapter 4, and you're gonna see a little bit more of a cell biology aspect of the development and cell biology or cellular interactions that are relevant to a lot of the demo biology you're gonna learn very soon thereafter. And then Chapter 5, I believe, is probably the last chapter that will be covered for the first midterm. or we'll be talking about some stem cells and cell differentiation. So probably, if today's lecture 6 this Thursday, probably next Thursday is the last lecture relevant for the midterm. Just to give you guys a preview. Okay, lots of cool stuff to get through. And so hopefully, we'll be able to do just that. Does anyone have questions from last week's lecture or anything like that? Okay, so just to connect back to the last example we're covering. On Tuesday we were discussing various different transcriptional post-transcriptional, and in this case translational. So here you see, ribosomes here illustrated at the translational level. Think how molecular mechanisms can be tinkered with. whether it's in a disease context or an evolutionary context to give rise to very different photic consequences in the context of translation. So we think of the ribosome, and we think all the ribosome it's super conserved, you know, even in not even just. You know yeast compared to us. But even if you compared the ribosome of a of a prokaryot to us like bacteria, there's so much in common across hundreds and hundreds and hundreds and hundreds of millions of years of evolution. And it's because it's there's aspects of translation. That's just universal. And how it's conduct and how it unfolds. and the molecules. And in the case of the ribosome, this huge complex that's in charge of meat eating translation. So when you think about that context. You know your first thought might be well. If you think about one cell to another cell in your body, the way that a ribosome works is pretty much the same. and I don't know how I might have talked about that my Macco biology class, but probably gave along the lines of that impression as well. But in fact, different cells, cell types can actually have within them ribosomes interacting with molecules and functioning in different ways. And a lot of that is mediated by the actual molecules that are actually within the ribosome in those certain types of cells. So I think about ribosomal Rna molecules, ribosomal proteins. Not all cell types have the same distribution of those ribosomal proteins and rnas within them. So if you compare one cell to another, there's differences in the actual structure of the ribosome. Although very, very small differences. They're significant enough to have dramatic consequence on things, including, you know, the development of your skeleton, for example, which this example is trying to illustrate. So if you think about this developing mouse, we see it laterally, you can see from the side you can see kind of lit up. Here are different parts of the axial skeleton. So this is supposed to represent Somites, which we'll talk about what they do later in the class. But basically there are these blocks along the anterior to posterior axis, where this particular right ribosomal protein 38 is, is expressed and functions within the ribosomal complex of the cells within those parts of the the developing skeleton skeletal tissue. and if you were to zoom in on. You know those cells and think about some genes that might be differentially translated. Well, they're transcripts, anyway. You can see this example of Rpl. 38, typically RPL. 38 helps direct normal in this case. So wild type. But in this normal context, lots of translation of hawks, messenger, Rnas, and as you're going to see as the class goes on, hawks, genes are very important for setting up your anterior to posterior identity. so notice I didn't say anterior posterior axis, so figuring out like where the beginning of of an animal to the end is, is a whole hierarchy of molecules which we talked about so a little bit at the skimming the surface level so far, but along that axis which segment becomes what is a thoracic segment versus an abdominal segment. For example, along your anterior posterior axis, the identity provided to those segments comes from the activity of things like hox genes. And so these hawks. These hawks, Mrna transcripts are translated at a high level. so lots of ribosomes are coming in. finding those transcripts and translating them quite rapidly to ensure proper identity. From anterior to posterior axes of your. In this case we're looking at the skeleton. If you were to mutate that ribosomal protein so that the ribosomes within those cells that you see you know the regions coming from the regions you see here. If you were to mutate that protein or the gene encoding that protein. Then all of a sudden, the the identity that's supposed to be properly set up along that axis is perturbed such that you can see here extra ribs start to develop off of the axial skeleton. so determining where to end. where ribs will actually emerge from your from your spine, so to speak. thing that's giving identity along those repeated segments from your head to you know, throughout your spine hawk genes are super important, and their protein activity and ribosomes. Differentially translating in a normal context or at very low levels in this perturbed, mutated context, can have profound impact on activity of these genes in a developmental context. So does that everyone get that. You know, ribosomes. Very important. Obviously, they're the. They're the machines for translation. But some cells translate transcripts differently than other cells. That's a very important exception. To our thinking, the classical thinking you might have from earlier classes. Okay. So now, coming back to the context of cleavage that we talked about mostly at the first few lectures of the class. So what you can see here is actually a zebrafish. embryo and zebrafish embryos are the type that divide. I cleave in part of the embryo, so you can see here you have a we'll look at wild type. We'll look at just the first column on the left. let me just make sure my is okay. So you see the first column here the wild type column you can see denoted in color is actually a stain that's staining nuclear material. And so you can see that nuclear material dividing across these different stages. So you have cellulose cleaving at the top here. This is the later stage. This is even much further stage. We have probably a hundred cells at this stage, if not more. And you can see the nuclear material, the nuclei stained within each of those cells. Now on the right side. Here you see similar cleavage patterns. If you look carefully, it's not as obvious how to tell the distinct cells, just because you don't see the same kind of staining in this in this right column. Now, the difference between the left and the right is that when you have this futile cycle mutant in zebrafish, if you mutate this gene. Then the actual fusion and nuclear fusion, fusion, so sperm and egg actually contributing towards the zygotic DNA, so to speak, so pronuclear fusion does not occur. And the point of this example is to show that if you were to figure out a way to stop the zygotic genome from being active and functioning. you still have cleavage events occurring, and some great example perfect. You know evidence to show that zygotic, the zygotic genome, and and the individual itself. Nothing to do with these first cleavage events in this animal. It's all up to the maternal determinants that are being deposited within the egg that facilitate these cleavage events. And so, even though you block pronuclear fusion from happening so sperm and egg, you know, fusing. And then and we'll learn about that extensively in the fertilization lecture very soon. Unknown Speaker 00:09:39 And then the zygotic genome being activated in cells. Rajendhran Rajakumar 00:09:43 So you shut that completely off cleavage of the embryo still happens. Okay, so maternal durammans are super important or mediating these cleavage processes. Way, before our own genomes are activated. So our parental contributions, contributions and molecules from our parents. Those are super critical in influencing how these cleavage patterns were to unfold. not. and we'll come back to in a second some more details about maternal contributions in a few slides, probably from now to give you some other ideas about again molecular regulation and how it impacts development. And of course, later in the class, we'll get more into how maternal determinants, you know, mediate these processes in more detail. So another mechanism. That can influence, you know the connection between transcription and translation of molecules in our cells, and that can lead to the consequence. Be consequential for perturbing developmental processes involves different epigenetic mechanisms. 1, one very important one, especially during early development, is the activity of micro Rnas again went over great, you know, detail in microbiology class for the purposes of just this overview, we'll just skim the surface. But for those of you who aren't familiar with micro Rnas, these are molecules that so these are basically Rna transcripts from genes that don't actually get translated. So they are transcribed. And then they form hairpins on themselves. And then, once they're in this double-stranded Rna confirmation. The cell detects them all. There's a double-stranded Rna. This is not meant to be now shuttled into the cytoplasm to be translated. It's meant to be processed in a very specific way, such that this Rna molecule is, is basically modified into these small little fragments, and the small little fragments actually are complementary to go and bind to the messenger Rna. Transcripts of other genes of the transcripts of other genes. And when that happens, when you have this partial complementarity of this little small Rna molecule, this micro Rna to its target messenger Rna through again. Base pair complementation. Then those are again detected by the cell, and either translations prevented of those transcripts, or they're actually just actively degraded and destroyed. And this is just an an overview of the pathway. Just to give you an idea. You don't have to memorize any of these steps that's more relevant to my macco biology class. Just to give you an overview idea of how you know in the nucleus you have. You know, there are genes that are transcribed and they, instead of being processed as from premature or preliminary messenger, rna or precursor messenger, rna prem messenger, and it's really more classical term, and processed exons introns all that kind of thing, and then shuttle into the set of plasma for translation. Instead. these small messenger rna molecules are folded on themselves. detected by machinery. That actually process this drosia is a very important factor of that. And then it's then shuttled into the cytoplasm. And then there's various steps that's mediated by what's called the risk complex. and the key molecule called dicer. It basically dices up. It chops up this, this hairpin into small pieces, and those small pieces of Rna can actually be. In this case, you know a piece, a key piece of micro Rna from that original transcript can go and bind so that molecule can go and bind to some target messenger. Rna. So in red, here's a micrna, and green is, you know, the messenger Rna of Gen. X. Super important for development. There's a micr and mic rna that can actually base complement. So you know, A's with use Cs with G's kind of is complementation. We're talking here. And when that happens, either translation's repressed or the target messenger Rna is destroyed. It's cleaved up. How exactly those steps are meeting all those kinds of things. It's not important for this class just to give you an overview. This is micro Rnas are another type of molecule that can have profound influence on the developmental process by just just by the fact that it's it's a type of molecule that can mediate gene activity during development. Okay? So now to tie together those last couple of slides, micro Rnas, and also maternal determinants, and regulating how do you go from that early embryo depending on factors that the parents contributed so from the that were deposited into the egg for the mother, and also Paternal determinants are coming from the sperm itself. How do you go from that parental contribution to now, the zygotic genome being activated, and your own genome now taking on the rest of you know your life essentially mediating cell behavior, cell differentiation of yourself. Well, micro earnings play a very important role. So what you see here are in these various different colors. These are messenger, Rnas. and various different molecules that are part of a various set of various different molecules, including proteins, too, that again are deposited by the parent in particular. These are different messenger Rna transcripts that can be translated. and as proteins can have very important functions regulating cleavage. For example, as you saw in that zebrafish mutant sled. Now, how do you go transitioning from these being the driving factors of development to now things coming from your cell's nuclei? Well, micro Rnas, play a vital role. So your nucleus of your various cells at this stage will start to produce micrornas and those micro Rnas can go and target the messenger Rna transcripts that were deposited by the mother and lead to their destruction. And once they're once they're completely depleted or degraded. Now, the only transcripts left in those cells are ones derived from your own genome. Okay. and again, previous to this degradation step, this transitional step. those transcripts can be translated in different regions of those cells, and mediate important early cleavage events and other very early embryonic developmental processes. Well, you see in this graph over here transcript level versus time. Unknown Speaker 00:16:28 You can see that the the maternal determinants are very high Rajendhran Rajakumar 00:16:32 and they're being translate. They're being localized in the cells in different ways. I'm going to show you a slide of that in a second, how transfers can be localized within a cell. and and they're being translated to varying degrees to again mediate key processes early in development. You can see here some kind of staying a little high. and then some are starting to go down. And, for example, if you take the most extreme one, you see this red transcript here that was materially deposited. Unknown Speaker 00:17:00 it gets basically completely shut off. Rajendhran Rajakumar 00:17:02 And that's because Micro Rna 4, 30. So there's hundreds of micro Rnas that are genome encodes. So the 431, or we'll just say, Micro Rna, 4, 30 will actually be able to bind and lead to the lead, the this maternal transcript to degradation and basically depleted. And so during this period of time, this key transition is unfolding, so it's called the maternal to zygotic transition. Pretty key, MZ. Or MZT but that's a little bit more in the classical sense that you know, these parental, determinant factors are only coming from mother being factors being deposited into the egg in. You know, the last couple of decades. We're learning more and more of how. You know, the sperm isn't just this nuclear donor of nuclear material. It also has factors within it, too, including epigenetically related factors. It's just much more poorly investigated and understood any questions about this transition, and how micro Rnas are regulating or playing important role in this transition? Yes. yeah. So the question was, why do we need to degrade those maternal transcripts? Why not just let them sit around and do their thing while the zygotic genome is activated and starts doing its thing. Unknown Speaker 00:18:26 It's basically kind of like, imagine you're watching a relay race Rajendhran Rajakumar 00:18:31 and one person's passing the baton to the next person. Right? That's that's really what it's about, you know, if they didn't pass the baton, if they're trying to do the 4 by 100 meter relay race all by themselves. They're going to be pooped out by the end of the race, right? And the same thing about the functioning of these transcripts. They're in a proper stable state and serve, as you know, templates for translation of various different important proteins in these stages, and they play the role in this very key time point in development. But then it passes the baton. The baton has to be passed to the zygotic genomes, you know role. Essentially. So that's pretty much more or less. Why, yeah. half-life of these molecules. Yes. one more time. Well, so actually, the micro Rnas are coming from the your, your zygotic genome itself. And what's coming from the parental gametes? Are these molecules you see down here? So this is this example is in this maternal kind of paradigm, which honestly, 100 years from now, we investigated much more deeply and with higher resolution. Probably maternal determinants still will be the most profound players in this process, and it could explain why they were detected so early on, with various different experimental approaches, so on and so forth. How much of a role the paternal factors play in these early events still is even to be determined whether it's a minor or major rule but regardless of that of the ratio of those contributions to get at your point these factors that you see kind of here down here, this red, purple, and green factors. That's kind of meant to represent the maternal factors that are deposited within the egg before your genome actually really comes together because of pronuclear fusion of the nuclear DNA from sperm and egg. Okay? So again, micrornas, it's your micrornas. Finally starting to do something for yourself. Basically move, allowing you to essentially move her to the house, so to speak. and and start taking a life of your own by destroying these parental contributions. Okay. Now, I had mentioned a little bit earlier. How these Rna molecules can actually be localized within the cells. They don't have to be equally distributed within each cell. They can actually be shifted to a certain area. Maternal determinants are a great example of factors that by being able to localize them to certain regions of a cell, you can generate lots of opportunities for really cool developmental patterns and processes to emerge. So, for example, think about a newly fertilized egg. So after fertilization you have the the embryo and cleavage events are now going to start to unfold right. Remember, from some of the earlier lectures I had mentioned, that when the first cleavage events are happening, you have Rna transcripts and proteins and various different factors. They can actually be shifted asymmetrically in a cell. And so, after cleavage. one of the data cells has more of some factor than another. and then more cleavage events occur, and this asymmetry just continues to perpetuate. and so imagine in that maternal to zygotic transition. And these key cleavage events happening before that transition occurs. Those maternal, materially contributed factors by being asymmetrically localized within the newly formed embryo. You can facilitate some factors going to some cell lineages and some factors going to others, and then eventually they're destroyed. They've done their role. They're destroyed. And then those cells own genomes start doing their thing. Okay? Well, how can you localize? Say, for example, we're talking about Rna transcripts into different regions of the embryo. So what you see here. is actually a right before fertilization. So in the egg already you have localization of factors, particularly Rna transcripts. So you can see here there's kind of 3 major categories of how you can localize transcripts within the egg. First of all. And again, this is, you know, this is a cell, right? Each of these are cells. And in the various diagrams you have so molecules. Rna molecules being shuttled to some region. So let's let's say, for simplicity, this is the anterior of the egg to the left and the posterior to the right, and the goal is, we need to get factors to the posterior specifically how to do that. So there's diffusion and local anchoring. There's localized protection. And there's active transport along the cytoskeleton. So for the first one diffusion and local anchoring you have transcripts flown around so they're diffusing in the cell. That is this egg and then at the posterior pool. There are specific anchor molecules that when the that was transcript, that transcript and highlighted in these blue kind of rectangles here, when they once some start to contact this posterior region, they adhere to these anchor molecules, and they stay there. And so it starts to get more and more enriched quickly, those transcripts being bound to the posterior pole. So that's one way. There's some anchor in one part of the cell, some molecule, say protein complex in one part of the cell that has an affinity for some Rna transcripts. and as time proceeds through just simple diffusion, method. Processes, those molecules will eventually start to get enriched in the regions where there are these anchoring complexes. That's one way. another way. So actually, just to give you some detail for for curiosity, curiosity's sake. So these actual transcripts. You know, there's various different molecules transcripts like this. But Nanos Mrna is probably the best understood as a molecule that gets anchored in this kind of fashion in the developing egg of fruit flies, drosophila, Montagasto. And so these transcripts of Nanos, very important for for the formation of the posterior of the embryo later, and also for the formation of the germ cells. the sperm and egg cells of the progeny. So they're flowing around through diffusion. And then, you have anchor proteins that actually bind to those nanos transcripts over at the posterior pool. Okay, another example in this case the messenger Rna transcripts that's really well understood, for this category are heat, chuck, protein transcripts. So this particular one, Hsp, 83. It's kind of like our Hsp, 90. Very important heat shock protein in our cells. you see here transcripts are floating around again. This category is called localized protection, and you'll see why in a second. So they're floating around. and they're getting chomped up by these little Pac-man looking things, these little red. you know, cartoons that are kind of chomping away at those freely diffusing transcripts. but at the posterior pole, if some of those transcripts were able to diffuse in that region. there happens to be these factors, these protein complexes that actually protect them from degradation. So it's more about localized degradation prevention rather than localized anchoring physical holding them in that place. Okay? And then the third situation, active transport along the cytoskeleton. So these egg cells, like many of our cells, have very complex cytoskeletal elements, actin and actin filaments. For example, among many other things. and and microtubules. Think about your cell biology classes. For a second you could kind of see them represent in these kind of spaghetti strands. Here these are various different microtubule structures. and along those microtubule structures, these big protein complexes, like kinesin and dining can walk along them. Okay, in a very directed manner. And as they're walking along towards this posterior pole, they're carrying with them transcripts of this model of molecules called Oscar and Bitcoin Bitcoin. We talked about a lot in a couple of lectures ago is very important for telling what's the head and what's the butt of a fruit fly? And of course there's some analogous molecules and processes like that for us as well. But in any case, for the specific example, these molecules, Oscar and Bitcoin, very important transcripts that will eventually be translated in the embryo. They're localized specifically to the posterior. By these by this transportation. Yes, actually. just to be more acurate, the Oscars goes to the posterior. And actually, Bitcoin is to the anterior. Just as I was saying, I was remembering actually examples from the previous lecture. Bitcoin is the anterior defining factor. It's what tells what the head will become as opposed to caudal, which is what tells what the tail, but will become in those examples earlier. So again, microtubule molecules moving Bitcoin to the anterior, you can see the arrow kind of moving to the left. and then you've got Oscar molecules again. Oscar is also very important for posterior formation, and particularly germ cell formation in the posterior microtubules, a different set of microtubules are actively transporting. using. in this case kinesin molecules specifically to the posterior pole, so that the transcripts are localized there any questions about those 3 different categories of transport? Or I guess localization, maybe, is more better way to phrase it. Okay. okay. So now I'll get into the territory of mentioning some various different techniques that we'll probably cover to varying different degrees during the class, just to bring them to speed of them, so that I don't have to re-explain them, and great in in introductory like detail. Every time we instead, we can talk about some of the more interesting details of the experiments. When we get to certain case studies or examples of how to study different developmental processes. So you see this embryo, it's a fruit fly embryo here. And you can see these stripes. It's actually an image very similar to some of the introductory lecture images I showed you earlier. So these are segments of the embryo. And actually, they're kind of wrapped around the the entire egg, you know, embryo that you see embryo body that you see here. And it goes from the anterior all the way to the posterior again. It doesn't look like it's the posterior in the sense that it's the end of something. But again, imagine this is a wrapped structure, and we'll visualize it in 3D. And time and videos and stuff much better in a later lecture. So you have these stripes along the anterior posterior gradient. and they're actually representing the trend. There are actually transcripts of a key segment. Segment formation gene called oddscript. Unknown Speaker 00:29:33 So every odd segment has odd skipped being expressed. Rajendhran Rajakumar 00:29:37 and every even segment. you see, are blank kind of like whitish cells. They don't have a color that's that's actually reflecting where odd skip transcripts are. Now, what's happening in this image? How does this technique unfold? But what's the general protocol. That's what I'm going to take a moment to give you guys an idea. So that way, using just this as an example more broadly when you're seeing different images during the class. Very soon it'll go from you, just taking my word for it. What? You're visualizing and actually understanding how. Yeah, how it was visualized. What was the experimental approach to visualize things? Because in devontal biology there's just as many methods to see things happening as there are experimental, cool experimental methods to tinker with the things that you see processes, to see. What's the consequence. Both the descriptive and the actual perturbation type, experimental manipulation type approaches in developmental biology. Okay, so what's happening? So imagine you have this self here. Imagine this kind of rectangular like thing is is a cell membrane. And inside it. You kind of have this dashed line nucleus, nuclear membrane. And inside that you have this little rectangle that's representing the gene odd-skipped. And you kind of have this little kind of line and suppression it's to, and it's in red is denoting that gene is not being transcribed in that cell. All right. Now you have this cell that's just neighboring it, just adjacent to it. If if anything, if even more details the fact that it's even contacting that cell in this cell, you again, you have cell membrane, and you have nuclear membrane. And within the nucleus you have again the gene oddskipped that's represented by kind of the gray rectangle. You have this weird olive colored like. So you know, oval. That's probably meant to represent polymerase to our Rna Polymerase 2 as transactively transcribing odd skipped in this nucleus. and that's represented by both. I guess this green arrow gene on, I guess. And this kind of green spaghetti noodle that's actually, the are a messenger Rna transcript of the oddskip gene emerging. So once it's transcribed in the nucleus. some transcripts play a lot, you know, spend a lot of time in the nucleus. A lot of transcripts can also localize or spend most of the time in the cytoplasm, particularly in the cytoplasm. That's where they're going to eventually get translated by ribosomes and turned into proteins. But here, you see again transcripts. They're localized it. They're they're being shuttled out into the cytoplasm. There's all these green lines that you see here. But then another thing you'll notice is that attached to these green lines are these weird structures here. So now I'm going to break down to you what those structures are. And ultimately the point of those structures are. Those are, those are synthetically, you know, produced generated molecules that can actually go in very specifically bind to. In this, let's use this example specifically bind to odd skipped transcripts, and after they bind to them in that very specific manner. You can treat the cells to certain with certain chemical reagents, such that those synthetically made factors will enable a color reaction to unfold. or they have some kind of fluorophore, some kind of fluorescent, like molecule that will actually be something that you can visualize using fluorescent microscopy as well. So either a color reaction will unfold. So something in this case turns the purplish. You can see with just regular white light microscopy. Or instead of this being like all this, purplish like coloring here, it can actually be some fluorescent image. We've seen many fluorescent images so far in the class. You can use visualize using fluorescent microscopy. Okay, so that's the goal. So how? What's what are these molecules? What's actually happening? So let's start off with the red bars here the red rectangles that are throughout the structure in the cell. So if you were to zoom in, that's Panel B at the top here. So the red is actually and the anti-sense of your transcript of interest. So you go through the process of actually synthesizing nuclear molecules. Sorry nucleic acids. So in this case, Rna molecules an Rna Strand, they can actually base pair can complement through base complementation your transcript of interest. So that's how it specifically targets your your Ge, your transcript of interest through base complementation. So imagine this is like this red Rna structure here represents like something that's like 500 base pairs, long bases, long, sorry, or or a thousand bases long, of an Rna molecule, and those 500 to a thousand nucleotides long. That specific sequence can go and base complement a part of a messenger. Rna. Transcript of your gene of interest. It's like 5 to a thousand bases long as well. Okay, so imagine this is the sense, and this is sense. You know, your messenger, Rna transcript is in the sense orientation, and then the red is entice sense of that, so it can base comple. Now, when you synthetically made this antisense, rna molecule part of the process of making that molecule is again putting A's use G's and C's, and again use. Because this is Rna we're talking about here and using those as building blocks to synthesize this antisense structure, right? But while you're doing that in the inio, in a tube, so in the lab, as you're synthesizing this artificially, the A's and g's, and C's are totally normal building blocks the use. On the other hand, the Uraciles. about like 3 quarters of them, are normal uraciles and a good quarter to a third are specially modified Uraciles, and these Uraciles have this molecule called dig or degoxagenin, that's actually conjugated to those uracil structures. The actual uracil molecules themselves. So there's a small fraction of bases in this in this antisense structure that you've made synthetically, that have these dig conjugated molecules attached to it. So that's what these yellow lollipops are throughout this whole thing. Now you'll notice there's if you were to really squint for a second here, there's these other things attached to those yellow lollipops, yellow lollipops. And that's what you see at the bottom of this slide. A little bit more detail to visualize. So you have, you'll see here the yellow lollipops that's the dig conjugated. What probe essentially, that's attached to this anti-sense Rna molecule that can bind to the sense Transcript messenger Rna transcript of interest to you. In this case we're talking about odd skipped. Of course there's many genes, of course, that could be interesting instead. Now what the heck is attached to it. So this is actually an antibody. and this antibody has been engineered such that it can, with incredible high specificity bind to anything that has dig oxygen and molecules attached to it in the cell, and the only thing that has oxygen and attached to it are these synthetically made ropes. Okay? So in a very specific way, once you treat, you take cells of a say, you have an embryo. you fix the embryo using formaldehyde based molecules. So it fixes the tissues, makes sure that all there's good tissue integrity when you're manipulating it in subsequent steps of the protocol and also basically to kind of freeze. Frame all the messenger, all the Rna molecules and proteins, and so on and so forth in the tissues. So now that you have this kind of snapshot of this exact moment of the cells within this embryo you permalize the cell, the the cells in this embryo such a way that it doesn't destroy them, but allows these probes. these that you see at the top here to go into the cells. You see, these are, and they're actually in the cytoplasm and the cells, right? So they go can go into the cells. And now they can actually base complement. Those green transcript that you see cartoon described here. Now, once that happens, you then. and I believe the probably the next slide describes it a little better. Why is this slide? It's not changing. Ok. So then, once the probe is attached to your transcripts, you then treat the cells, the tissues that you're working with with that antibody that can actually bind to Degoxagenin. and it'll with very high affinity binds to any molecules within a cell that has that deoxygen and structure conjugated to to it. So that's what you see as kind of denoting the kind of orange-ish color. Sorry the kind of forked structure here the antibody, and then a part of the antibody has this little orange extra piece attached to it. so these antibodies that have a hyphen you to Degoxagenin. They have attached to them a molecule called alkaline phosphatase. Now, that's one type of antibody against oxygen. And there are other types, too. But in this case this alkaline phosphatase is is an enzyme that if you were to treat it with a combination of the 2 particular molecules called Mbt. And BC. That you see listed here, then together. It'll form a chemical reaction and turn those 2 substrates purple. And that's why the cells will appear purplish as opposed to no color that you can see in this cell here, and that's why you can imagine cells that are purple in the whole embryo. Where are regions of the embryo that are purple versus regions that are clear. Essentially so. To recap your messenger, Rna transcript is, in a sense, orientation. You've since you artificially engineered an antisense version of it, or at least part of it that can come and base complement to it in a very specific manner. Part of that of that antisense structure is the conjugation of this deoxygen and molecule. and together are what are called a dig conjugated probe. So it's an antisense. Dig conjugated. Probe this once it's bound to your target transcript. You then can treat the cells with an antibody that has a high affinity to this probe. and once they bind you, treat the all the tissues with that Mbt. BC. IP. I had mentioned here, but the the exact names of them are not important just to give you. And just to think of. You treat it with some chemicals. And those chemicals are basically provide a substrate to this chemical reaction to change color. And so you can now visualize it with your eyes in regular white light. Now there are other antibodies that instead of being conjugated with alkaline phosphatase that can take this, these chemicals and turn them purple instead. The antibodies conjugate it to some fluorophore. Say, imagine fluorocene, for example, and that you can visualize using fluorescence microscopy. So rather than in. You needing this extra chemical reaction step. you can instead just visualize the fluorescent molecules that are attached to the antibodies wherever they're being localized. Okay, so that's to give you an idea in detail. Kind of the the key elements, the structures and the steps involved in doing what's called in situ hybridization, which ultimately is to look at gene expression patterns within the embryo. Because a very important thing to think of, if you haven't already in the class moving forward is again the fact that if you look at all the cells across an embryo, they don't all express the same transcripts. different cells in different regions and in different time points express transcripts to varying different degrees. Okay, how do you visualize that using techniques like this? How does the embryo do that? Various different mechanisms. We're going to learn during the class how some tissues will spatially or in a temporal context, express transcripts or not. And how do you study their function. Various different techniques to perturb those transcripts prevent them from being transcribed, or lead to their degradation or mutate to the genome. So the transcripts are screwed up when they're transcribed. So you can study their function. Okay? So ways to visualize things. understanding the mechanisms which distribute things in space and time in an embryo and then methods to study their function. Okay. another method, just to give you an idea of some interesting things people can do to study developmental processes is a method called chromatin immunoprecipitation, sequencing or chip seek. So basically, what happens is you fix the embryo in the same way that you did for the in situ hybridization technique on the previous slide. And so now again. rna molecules, proteins! They're all locked in in certain place in the cell where they were. That's what fixation molecules like formaldehyde effectively do. Now, in the previous slide, we're really interested in, you know, where certain Rna molecules were and targeting Rna molecules. In this example, we're actually targeting proteins and not just any proteins, but proteins that are very important for mediating or regulating gene activity during development. So you can imagine they can be so proteins that are very broadly relevant to cells like, say, for example, histone proteins, or they could be very, very specific proteins to certain specific subset of cells like, imagine a transcription factor you're really interested in and what the transcription factor is targeting in a very specific subset of cells. But ultimately it's about protein. You're interested in what nucleic acid molecules are they interacting with within the cell? So again, transfusion factor, which part of the genome. Is it binding to would be probably the best thing to imagine. So you have. You know, illustrated here. You know the cell DNA with interacting proteins. So imagine those interacting proteins are transcription factors. So you have your genome transcription factors are interacting with it to mediate their transcription. But if you fix the cells they're stuck in that position. Those transfusion vectors are now totally stuck, too. The DNA regulatory elements they're bound to, whether they're enhancer elements or repressor elements. then you can isolate all of that DNA material along with the proteins and transition factors bound to that DNA material. and you can put it through a protein column which you might have learned about in some of your biochemistry classes. But if you haven't, just imagine you have a tube inside the tube are these small little beads and these small little beads can have an affinity, some kind, through some kind of chemical physical affinity, to different types of classes of protein structures, protein, chemistry. And so when you run through this column. you can, using now either some kind of probe, or even an antibody, for that matter, like in the previous slide, a little bit that has a high affinity to your protein of interest. So imagine you're interested in Pax 6 or eyeless. The gene we were talking about the other day regulated, you know, retina development, the development of the eye and the pancreas right, and you want to know what are the exact enhancer sequences. eyeless or pax, 6 binds to across the entire genome. So what you can do is you do this, this fixation process just as I described. And when you take the when you, when you isolate all the nuclear information that's bound with by. You know, these transcription factors in the column, you can actually put antibody against that specific transcription factor, Pax. 6. And so all the Pax 6 proteins will be bound by this antibody and all the DNA that that protein was bound to will be all in this kind of complex together. and then you wash the column through multiple times to rinse off any things that weren't bound to that antibody. and after that you can then elute the antibody a different, you know fluid to wash through the column, and what you have at that point is all of the parts of your of the genome of the cells that you're isolating the genome from the nuclear material. From that the pack 6 transition factor was bound to. And you can then take that DNA sequence. It do, DNA sequencing. and all of the sequences you get back are sequences of DNA that that pack 6 transition factor bound to as opposed to all the rest of the genome, so it'll give you a very specific subset of sequences. And then so once you know all these sequences of DNA, that that transition bound to you can align them. You can use bioinformatic methods to take. Say, we're talking about humans here, the entire human genome, and you can align. Where are those sequences in our whole genome? And once you and once you figure out where the locations are. you can ask yourself what are cool genes that are near those enhancer elements that now, Pax, 6 might actually regulate. So there's all kinds of cool things you can actually derive from this kind of experiment, you can figure out what parts of the genome your transcription factor of interest actually binds to. So what are the regulatory regions? And then, when you look, what are our nearby genes close to those regulatory regions that can give you clues. lead you to start developing some hypotheses about genes that actually are regulated by your transcription factor of interest. So make sense so far for everyone. Anyone have questions about that process. Yes. yes, yeah. Yeah. So it depends on how the column's structured, basically. But basically And typically, but for chip-seq, you know methodology that you see here, it's such that anything that's bound to your that antibody that you're specifically using to target your your protein of interest. They're not able to flow through using certain again solvents to actually pass through this column. Unknown Speaker 00:48:03 But then, once you do that, once you rinse off everything else. Rajendhran Rajakumar 00:48:06 and the antibody's keeping things together within the column, then you can run through that column other fluids, other solvents that can actually allow it to elute to come through the column. Basically, yeah, nice. so yeah, again, chromatin isolated from cell nuclei chromatin proteins. They're cross-linked through fixation methods. I was mentioning to you to the DNA binding sites that they they bind to. They kind of in a snapshot. They're kind of frozen in time. Essentially, antibodies bind to the specific proteins. In this example. They're talking about chromatin proteins. That's another thing. You can imagine you made an antibody against specifically you know the 20 seventh like positioned Lysine of the third Histon's tail. Okay, so H. 3 k. 27, and not just that. Only when it's an antibody that only binds to it when it's acidillated. And what happens when H. 3 k. 27 is acetylated, it allows for Euclorman information, active confirmation of of chromatin like we're talking about in perhaps the previous lecture. Maybe it was 2 lectures ago. I don't remember exactly. But if you're interested, what parts of the genome are in an active state in certain cell, re certain tissue at a certain time in development, which part of the genomes are being turned on, you can actually use antibodies to specifically target modified amino acid residues of histone tails. To see, to use as as a marker, to know what regions of the genome are in an active state. and again use one. You fill them through these columns, and then in the end, you isolate just sequences of DNA that were nearby, that were part of the nucleosomes that were that so the DNA was wrapped around where that specific H. Histone 3 was actually acetylide. Of course there are antibodies against methylated H. 3 k. 27 as well, and antibodies against all kinds of other different histone tail modifications, and knowing that some of these marks denote repression of genomic regions, some of these marks denote active or gene activity or activation. You can actually do a bunch of these chip seek experiments. So together, you kind of have. Well, before I say it together individually, you have. These are all the parts of the genome that are repressed actively. These are the parts of the genome that are are actively being, you know, accessible for transcription, and then together you get this big dynamic picture of here is at that in that particular tissue, particular time in development. This is the dynamics of the genome. This is what's being turned regions that are being turned on or turned off effectively. So that's it. Say, the antibody was against chromatin protein modifications. But, as I said to my first example, it could be antibodies against certain transcription factors. You want to know where they bind in the genome. So there's all kinds of cool things you can do with Chipsek. okay. so speaking of sequencing. So that was, that was chip chromatin, reader, precipitation and DNA sequencing. In this case you have Rna sequencing methods that are used extensively to study developmental processes. So imagine here you have a developing chick embryo. It's at the stage 33Â h. Lots of action has happened by this time. You have a chick embryo here that's 3 and a half days old, and chick embryo here. That's 6 days old. You can kind of see its eye. This is just its face taking up this whole panel right? This is its head, whole head region, and a huge part of its trunk here. This might be yoke over here. I'm not totally sure. And then this whole thing is like the entire anterior half of the embryo posterior tail would kind of be off the picture here. And so in each of these diagrams you see here, you have a box that's actually kind of highlighting. homologous developmental regions. So the developing eye region essentially at these different stages and say, you wanted to know what genes are being turned on are being actively transcribed in the developing eye at this early stage, this middle stage of eye development, or this late stage of eye development. How would you go about doing that? So what you can do is you can actually take embryos like this. cut the tissue of interest out. and then you can isolate all the Rna transcripts from the cells of that tissue, and then you can sequence them. And when you compare the sequencing results of these 3 sequencing runs so sequencing Rna from this region, this stage, this stage, and that stage. You can get an idea of what is the activity of the genome of developing cells that are trying to start to form an eye during eye development and at the kind of the end of eye formation. Essentially to again start to piece together gene regulatory networks, like I had mentioned earlier in a couple of lectures ago, how various different genes interact with each other to activate and repress one another, such that you can actually have this thing that looks kind of ambiguous. What this is going to become to looking something I like to being a full-blown functional eye. How do you get through that process? Well, if you're interested in what transcripts are playing a role in in these time points. Rna, sequencing is a very good go to method. just to give you an idea. A little bit of a breakdown of the steps of Rna, sequencing not that you need to know it in great detail whatsoever, but just to give you again an idea of not just naming the methods, but give you a chance to understand the process. So say comparative samples up here. Imagine it's the 3 different stages of chick embryo that you isolated eye tissue. That's what's going in here. Unknown Speaker 00:53:50 You strat, you extract messenger rna. Rajendhran Rajakumar 00:53:53 and then you have these messenger rna molecules. You reverse. Transcribe them into DNA molecules, because DNA molecules are what you use as templates for sequencing reactions. So this is now the DNA version of all these messenger Rna transcripts and then you put adapters on all of these DNA fragment molecules, and those adapters have specific primer sequences attached to them. They can use to sequence all of these Rna transcript fragments, thousands and thousands and thousands coming from these cells that you've isolated. And then, after you get the data, the sequencing data, you can assemble it all together, map it to the known genome to know where these transcripts are coming from, these pieces of of nucleic acids, what they're coming from which genes are corresponding to. And then you can start using bioinformatic workflows or pipelines to basically describe as a whole and also great. You know, specific detail which genes are essentially turned on or turned off. and those specific stages of development in that specific region of the developing embryo in this, in that case in the developing eye on the previous slide. That's kind of the the overview of the process of doing rna-seq like that. Another cool method. So in this case not descriptive, like some of the examples we just went over, but a method that can allow you to test the function of some gene that you think has a important role during the developmental process. So many of you might be familiar if it's varying to some of different degrees. Crispr cast 9 to Gene editing, talked about it extensively in my microbiology class. If you haven't taken that, it's all good. There's no need to understand the intricacies of Crispr. Cast 9 mediated gene, genomic engineering or modification. It's just again to tell you this is a very go to method, a key method that people use a lot Unknown Speaker 00:55:46 to study Rajendhran Rajakumar 00:55:47 developmental gene function or gene function during development. So basically, you have a guide. Rna, you have this big castline protein. Again, I'm going to try not to. I could spend a whole like 20Â min just talking about this to explain you the steps. I'm going to try to avoid going my molecular biology mindset right now, and just think about it as a devontobiologist. So again, you have this cast iron protein. It's actually derived from bacteria again. I won't go on a tangent here. And you have this guide. Rna, that is actually designed such that it can go and bind to a specific region of the genome that you want to edit or modify. So this rna protein complex will go into cells. identify the region where the guide Rna can actually base complement. And then, once that happens, the cast 9 inherently in the way it functions in bacteria to fight off viral infections. Again, I'm going to refrain from going on a tangent here about why, that is allows for the cutting of DNA at that specific region. and when DNA is cut again. I'm not going to get the microbiology of homologous recombination or non-homalous endrunning, or something like that. But just take my word for it, and DNA is cut, it can induce DNA repair processes, and when that happens, it can. The result could be a deletion in that specific region of your of the genome. And that deletion. If in the right place of the of that gene, can lead to the loss of function of that gene, you can study. Basically, you're creating mutants using this kind of technique also through this method, instead of just deleting some parts of the genome, you can actually insert things into the genome the first being so, deletions being the result of non-homogeneous and joining repair the second one, inserting things being the result of homologous recombination, but I'll just name them and leave it at that. But that's kind of what you're seeing through these 2 processes here. You know, doing modifications versus swapping in whole regions of of nuclear information into DNA. So just understanding the overview of this technique for this class. that's really what is the point. Why, I'm bringing up a lot of these techniques. You don't need to know the nitty-gritty mechanisms at play here. If you're interested, take my microbiology class but yes, of course, these notes are meant to give you a better understanding of some of things. I just waxed on about. Okay. Another cool technique to test function of potential developmental genes is the Galfour Uas system. It's a beautiful technique. It was. It's, you know. first started in the 1980 S. PA. Yeast. Geneticists, molecular biologists, and biochemists that use yeast to study various different molecular processes. Started to describe the gal for gene, its regulatory elements in incredible, unbelievable detail how it works, what binds to it all kinds of different things, the the activating sequence that the galphor protein actually binds to to regulate transcription of various different things. So basically, you have 2 key components here that are derived from yeast genomics, yeast genetics. the Galvor gene which encodes a protein, a transcription. Very again, probably one of the most well-studied transcription factors ever the galphor protein or transcription factor that combine to what's called an upstream activation sequence or uas. So this is just an enhancer sequence. Okay, and that transition factor when it binds to upstream activating sequence can facilitate gene activation of whatever genes are nearby, so they influence their transcription factors. They influence gene activity like we talked about in earlier lectures. Now that's in yeast. But so but you can make transgenic animals that have these things inserted into their genomes. And that's just what fly drosophila melanogaster biologists did in the early 90 S. So they engineered flies such that if you had one fly it can have in its genome the galph 4 gene that encodes the gal. 4 protein transcription factor you can have in another flight. The gal for binding site or the upstream activating sequence. Okay, so if you cross the fly that has this structure and this structure in their genomes. Unknown Speaker 01:00:21 the progeny will have in their genome Rajendhran Rajakumar 01:00:23 both the galphor, protein gene and the upstream activation sequence. Okay, so when gal. 4 is transcribed. the protein will go and bind to wherever the heck, the act upstream activation sequence is right? Okay? But where? When? What like? What what can you use that that phenomenon transcription factor? You can actually insert it in a genome. the gene that encodes it, and it can bind to something that you know with high affinity and super specificity. What can you do with that phenomenon? Well. in the fly that has the gal for Gene. you can insert it specifically right nearby or downstream, some particular enhancer sequence you're really interested in. You think this enhancer sequence is super important for mediating the development of a very specific type of structure organ cell type. What have you? so in this particular example that we're going to explain in a second. this enhancer region is bound by transcription factors that are made in cells specifically called imaginal discs. These are populations of cells that pattern and grow together to make the adult structures in a fly. Okay. so imagine in only those tissues and only the cells of those tissues. There are transcription factors that combine to this. Okay? So you've inserted this gal 4 protein right downstream of this regulatory thing. Okay, so it should only be active. This Galfour protein or the Gulf war protein coming from this gene should only be present in those tissues and not in any other tissues. Okay? So now, already, you're getting a tissue specificity, you're you're basically able to make this transcription factor only in specific tissues of interest to you. So this this structure over here, I said it was only related to maginal tissue to make the adult appendages or organs of the fly. This enhancer sequence, or the region of the genome. The insert this golf or gene into this nearby some enhancer sequence. You're interested. Whatever enhancer sequence you could imagine, whatever part of the genome you're curious about, you can insert this structure into, okay, they can allow you to have tissue specificity. Now, the other, the other individual that had that the binding sites for the transcription factor to bind to it's upstream of a gene you want to turn on like crazy. Okay? And how does that happen? Well, in the progeny? In the cells that make Galfour protein. It'll bind to this upstream. Activating sequence is gal, 4 binding sites. And when that happens it'll turn whatever gene is. That's right near it on like crazy. Okay, it it up regulates it. So Crispr methods. I was kind of alluding to earlier. Typically people think about like deletions. And and in general, we talk about mutants all the time in genetics. You mutate a gene what it leads to a loss of function. What's the consequence on phenotype? Right? So say we're talking about an I gene, you mutate it what happens to the eye development. So, therefore, that gene is necessary for that aspect of I develop right. But what about turning things up? Increasing an expression of something Unknown Speaker 01:03:34 and talk about gain of function experiments? So that's what this experiment did for the first time Rajendhran Rajakumar 01:03:39 in all organisms to be honest with you. Animal! All multi! So all animals, for that matter, gain a function. Experiments. So it was published in 90 93 in the journal development, actually, by drosophila monogastrobiologists. So again. specific enhancer of interest to you only cells that make transcription factors that bind to that enhancer, will it be active? And when it's active, galf 4. Gene is turned on makes gal. 4. Protein. This gal, 4. Protein only binds to a very, very specific upstream activated sequence that you've inserted in the other individual, the other parent. So these are 2 parent flies. Imagine there's a female as a male, for example. and it bites to that, and when it happens it will up regulate this gene. So it doesn't happen in this fly. It doesn't happen in this flight. It only happens when you cross them and the F one progeny have both this structure and this structure. then you can have these little. I don't even know what macaroni pieces coming on binding on this upstream, activating sequence and up regulating that gene. Now, this gene in this particular example is Pax. 6, and when you operate, pack 6 in any tissue it will induce eye formation. You'll get eyes being made in this particular example. You see eyes in a normal location, and basically eyes popping out of where the antenna is supposed to be of the fly. So they have, like 4 eyes popping another face. And the reason why that happens specifies is because the enhancer that the galphor protein was being made in just so happen to be the antenna imaginal tissue in the developing antenna tissue. And so in developing an internal tissue, you up regulated this, I inducing Gene what the heck happens this and we'll get into more examples of that later. Actually, I don't even know if it's antenna they're saying mouth parts here could be, it could be actually specifically tissue giving rise to the mouth parts of the fly, whichever it is you could do say, for example, this sequence is only active in developing wings. Okay, you'll have eyes popping out of the wings, developing legs. only cells. You have eyes popping out of the legs. That's that's what this gain of function kind of experiment can do, especially when you're manipulating important developmental genes like Pack 6, that transcription factor that you see in this example. But we'll get into more details of this as we go through the class. Unknown Speaker 01:06:06 we doing for that Rajendhran Rajakumar 01:06:08 another important method, predominantly more in the mammalian vertebrate systems is called the Craylocks technique. So basically what you have here. So in most cells, no recombination. you have the enhancer for something. And this particular example you talked about in the textbook is an enhancer for albumin. Okay, this enhancer for right near this enhancer for albumin protein. you have a create what's called a cray recombinase gene. So a gene that encodes a molecule, a protein called Cree recombinase. It's allows for recombination, you'll see in a second. So that's inserted. Since artificially into the genome of this animal, and it's near this enhancer for album albumin. Then you have another allele that has. you know, eggs, as you see, eggs on 1, 2, and 3 here. These are exons of this particular gene called H and F. 4, a could be XYZ. It doesn't matter what the gene is. Okay. Point is that you have these factors called these little purple bars that are so inserted around this exon tube. they could have been inserted around. Exon one exon 3 whatever. Just in this particular example, they're inserted around this particular exon. 2. So you have these 2. So 2 structures here. an enhancer for albumin that's right near this crater recombination, Gene, that you've inserted near it. And then you have. What are what are these little purple bars here around this exon, too. These little purple bars are called locks. P sight, binding sites. And what these locks P binding sites are, they're basically landing strips for the enzyme that this gene recombinase gene encodes. So when you have an animal that has both of these in their genome, okay, both of these structures you have described here the query recombinations in their genome near some enhancement of interest. And the Locksp site binding sites flanking some coding sequence material. What happens is what you see over here. So basically, when the crater in combinance enzyme is made, it can it go and specifically bind to those locks? P sites? It causes the bending of that DNA, and then pops up, pops off everything in between. So it removes it. So this is actually a loss of function experiment. It's how you can induce mutations in the genome of animals. So that's what you see here. So why, the enhancer for albumin near the career accommodates what was special about that. Well, so now, in most cells, there's no recombination. So the animal has both of these things. Okay. But there's nothing really happening. Prayer recombinase isn't being made because cells. you know, there's no expression of albumin. Okay, in liver cells. On the other hand, where there is the expression of algorithm, this enhancer is active and craver combinations made this protein here that will combine to those locks. P sites by bending the DNA. And once it's bent. it basically pops off that sequence. It's long gone, and all you have left is exon one and 3 next to each other. So you basically have a mutant form of this gene. Now, this isn't just when you see the surface, just a technique to mutate something of interest to you. Right? It's something that it's it's a method that you can mutate the specific gene only in a specific subset of cells while leaving the gene totally Ok, everywhere else in the developing embryo. Okay, also, there are cool modifications of this technique, such that you can even influence when during development in those specific cells things are, you know, things are happening unfolding. Okay? So you can control space and time. How you actually mutate a particular gene in the genome of an animal. So in this case, you know a man. Now, why is that advantageous? Many demental genes? If you mutate it in an animal it won't develop. It might be completely, embryonically lethal. Maybe cleavage doesn't happen properly. They die, or whatever they have 2 heads, and they don't survive whatever it is. The developable consequence might be profound enough that they don't survive to the point where you can study the phenotypes, the phenotypic consequence. And so, instead of mutating thatyl gene in all tissues. you can use this kind of technique to mutate them in a specific subset of tissues of interest to you, then make sure you're not having deleterious effects on the rest of that body of the animal, and you're only perturbing your tissue of interest to know what that gene does in that particular tissue. So that's the Craylocks technique that's used extensively in many animals. Any questions about that technique? maybe I'll just skim this, and we'll start with the slide next lecture. But just to give you a heads up. So this is actually, as the title denotes, figure 4.1. We're now getting to Chapter 4 of the book. I think we I don't know what chapters we covered so far, but the point is, we're now going to Chapter 4 and it's going to get a little bit more into this nitty gritty of the cell biology of development. To give you an idea. Imagine, if we were just to compare the way that cells communicate with each other. To give you kind of a foreshadowing of what next lecture is going to be. You can have autocrine signaling, which is actually not even shown in this slide where a cell can make a molecule that signals to itself. Okay. you can have juxtapint signaling, which is where you have a cell. communicate with another cell, because at their membranes are molecules that can actually touch each other and interact with each other. That would be juxtapressing. You can have paracrine signaling where you have these short-range trends, distribution of molecules from one cell to a very close nearby cell, so they're not in direct contact with each other. but they're molecules that could be expressed from one cell and perceived by the other. The other one might have a receptor being made. So a receptor located at their membrane, while the other cell signaling to it is actually secreting these paracrine fractures. And they're signaling molecules or ligands that influence this receiving cell. And then, of course, you can have endocrine signaling. We can have long-range distribution of molecules. In this case. We know them more commonly as hormones that can be secreted from one gland. For example, when tissue. and perceived by something way more distant and influenced their physiology or development. And so autocrine, juxtacrine, paracrine, endocrine. These are different forms of cellular tissue communication. It's very important for meeting the adultal process, and we'll get into some of that next class. So I'll leave it at that for today. all right, and we'll start from here next class.