Lecture 4 - Synaptic Communication PDF
Document Details
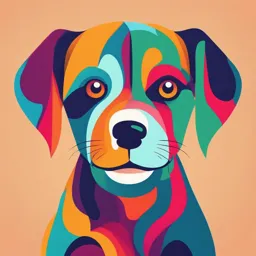
Uploaded by MomentousCurl7980
McGill University
Jonathan Britt
Tags
Related
- Week 7 - The Chemistry of Behaviour PSY1BNA PDF
- PSYC 215: Introductory Cognitive and Behavioural Neuroscience Lecture 1 PDF
- PSYC 215: Introductory Cognitive and Behavioural Neuroscience Lecture 1 - PDF
- PSYC 215 Lecture 1: Overview of Neurons PDF
- Lecture 16 - Cellular Learning and Memory (PSYC 211) PDF
- Neuroscience: Exploring the Brain PDF
Summary
These notes cover Lecture 4 of a 24-part course on introduction to behavioral neuroscience, PSYC 211. Professor Jonathan Britt's lecture focuses on synaptic communication and includes diagrams of neuron and ion channel structure. The material includes detailed molecular biology relating to ion channels.
Full Transcript
Introduction to Behavioral Neuroscience PSYC 211 Lecture 4 of 24 – Synaptic Communication (Chapter 3 in the textbook) Professor Jonathan Britt Questions? Concerns? Please write to [email protected] 1 MEMBRANE OF A NEURON...
Introduction to Behavioral Neuroscience PSYC 211 Lecture 4 of 24 – Synaptic Communication (Chapter 3 in the textbook) Professor Jonathan Britt Questions? Concerns? Please write to [email protected] 1 MEMBRANE OF A NEURON ++ ++ 2 ION CHANNEL SELECTIVITY In the last class, I introduced three voltage-gated ion channels: 1) Voltage-gated sodium channel (used to initiate and propagate the action potential) 2) Voltage-gated potassium channel (to quickly restore the resting membrane potential) 3) Voltage-gated calcium channel (to trigger the release of neurotransmitter) Each of these ion channels is highly selective to one type of ion: Na+, K+, or Ca+. How is it that an ion channel can be permeable to K+ but not Na+? K+ is bigger in every way (more protons, neutrons, and electrons). And it has the same charge as Na+ when dissolved in water (+1). How do potassium ion channels only let in the bigger element? 3 MODERN MOLECULAR BIOLOGY We know a lot about ion channels. We know the DNA letters (the exact sequence of nucleic acids) that encode these proteins in numerous species. Accordingly, we know the exact string of amino acids that form these proteins. We also know their precise 3-dimensional shape, the arrangement of all the atoms in them (via X-ray crystallography). 4 MODERN MOLECULAR BIOLOGY Imagine this segment of DNA encodes the voltage-gated potassium channel TCAGGCCCG These 3 letters encode the amino acid glycine To test whether that glycine amino acid is important for the selectivity of this ion channel (K+ versus Na+), we can alter the DNA sequence and change the protein. TCAGCCCCG By synthesizing this strand of DNA in the lab and then injecting it into a cell, we can get that cell to make our modified version of this protein (one where that glycine amino acid is swapped out for an alanine amino acid). This experiment will allow us to test if that glycine amino acid (in the folded-up protein in the membrane) is responsible for the selectivity of this ion channel. 5 MODERN MOLECULAR BIOLOGY Researchers routinely synthesize DNA and inject it into cells. Cells will read foreign DNA and make the corresponding protein if we attached a gene promoter region to the start of the DNA to tell the cell this gene should be read. Gene promoters are regions of DNA that initiate gene transcription. Gene promoters indicate which cells should read the gene and when. Different gene promoters are found just before every protein-encoding gene in the genome. gene encoding the gene promoter for the TCAGGCCCG Normal section of DNA: potassium ion channel potassium ion channel (in our genome) gene encoding a modified gene promoter for the TCAGCCCCG Man-made strand of DNA: potassium ion channel potassium ion channel 6 HYDRATED IONS When dissolved in water, ions get surrounded by water molecules. We say they become hydrated (i.e., encased by a hydration shell). Ion channel selectivity filters are precisely designed to replace the hydration shell of a particular ion. K+ ions are equally happy when inside the pore of a potassium ion channel or when surrounded by water. Na+ ions are too small to comfortably fit (unhydrated) in the pore of a potassium ion channel, so they prefer to stay outside of those ion channels with their hydration shell intact. 7 VOLTAGE-GATED POTASSIUM CHANNEL 8 THE HUMAN GENOME CONTAINS 40 DISTINCT GENES FOR THE VOLTAGE-GATED POTASSIUM CHANNEL The first one appeared over a billion years ago, before the evolution of multicellular organisms. Most of these gene variants have been conserved for hundreds of millions of years, meaning they haven’t changed much in that time. There is no perfect voltage- gated potassium channel. There are 40. Each cell can choose to express one or any combination of them to optimize cell function. 9 CELLS TYPES IN THE CENTRAL NERVOUS SYSTEM There are two types of cells in the central nervous system (CNS): neurons and glia. Neurons are responsible for the electrical signals (action potentials) that communicate information about sensations and movements. Glial cells serve a variety of support functions for neurons. Estimates of the number of neurons and glial cells in the human brain vary widely, but recent estimates suggest there 85 billion of each. 10 TYPES OF GLIAL CELLS IN THE CNS There are 4 types of glial cells in the central nervous system (CNS). 1. Astrocytes provide a structural matrix. They physically surround synapses and blood vessels, regulate the ionic composition of the extracellular solution, help with neurotransmitter clearance, and regulate blood flow and nutrient distribution in response to changes in neural activity. 2. Ependymal cells line the fluid filled ventricles at the center of the brain and spinal cord. They circulate cerebrospinal fluid: the extracellular solution of the central nervous system. 3. Microglia are the smallest glial cells. They are the brain’s clean-up crew, removing dead cells and other debris. They also serve an immune function and protect the brain from invading microorganisms. 4. Oligodendrocytes produce the myelin sheath, which encapsulates axons to speed up the action potential. 11 OLIGODENDROCYTES During development, oligodendrocytes extend branches of their cell membrane. Each branch wraps many times around a nearby axon to form one 0.2-mm-long segment of myelin. Myelin is a wrapping of fat (glial cell membrane) that electrically insulates the axon and speeds up conduction of the action potential. Most but not all axons in the brain are myelinated. Myelin sheaths are precisely organized. Each segment of myelin is separated by 1 micron of exposed axon. The exposed segments of myelinated axons are called the nodes of Ranvier. They are the only places where myelinated axons feel a charge difference between inside and out. 12 CROSS SECTION OF OPTIC NERVE axon myelin 13 14 THE DISTRIBUTION OF IONS WITHIN AN AXON cell membrane 15 +40 mV +20 mV 0 mV -20 mV +40 mV +20 mV 0 mV -20 mV 16 THE IMPACT OF MYELINATION Myelination speeds up conduction of the action potential 20x. Action potentials in myelinated axons appear to jump from one node of Ranvier to the next. This property is called saltatory conduction. The amplitude of the action potential (+40 mV) is regenerated at each node of Ranvier because this is the only place where myelinated axons can access extracellular fluid. All the voltage-gated ion channels in a myelinated axon are concentrated at the nodes of Ranvier. The speed of the action potential also depends on the thickness of the axon. Thick, myelinated axons have the fastest action potentials (100 meters/second). Thin, unmyelinated axons have the slowest action potentials (1 meter/second). 17 Synaptic Communication A synapse is a junction between the axon terminal (terminal button) of the sending neuron and the cell membrane of the receiving neuron. Communication across the synapse is mediated by the release of a signaling molecule (a neurotransmitter) from the axon terminal. When a neurotransmitter activates a receptor on the receiving neuron, the consequence can be excitatory, inhibitory, or modulatory (i.e., depolarization, hyperpolarization, or something more complicated). 18 The Synapse The axon terminal (terminal button) of the sending neuron is called presynaptic membrane. It is where neurotransmitter is released from. Synaptic vesicles contain molecules of neurotransmitter. They dock at presynaptic membrane and release neurotransmitter into the synaptic cleft. Synaptic cleft is the space between the pre- and postsynaptic membrane. Postsynaptic membrane is the membrane of the receiving cell that is opposite the axon terminal. Neurotransmitters released from presynaptic membrane flow across the synapse to postsynaptic membrane, where they bind and activate receptors. 19 PHOTO OF A REAL SYNAPSE Electron microscopy allows us to see small anatomical structures such as synaptic vesicles. It was used to create this photo of a synapse between a motor neuron and muscle cell in a common frog. dense core vesicle presynaptic membrane (axon terminal) The diameter of things 0.001 mm = 1 um = 1000 nm postsynaptic small synaptic vesicle = 30nm membrane (it can hold >5,000 molecules) (muscle cell) typical protein = 3nm mitochondria = 1um 20 21 NEUROTRANSMITTERS ARE SIGNALING MOLECULES A signaling molecules that binds to a receptor is called a ligand. Signaling within and between cells occurs through ligand-receptor interactions. There are two categories of neurotransmitter receptors. ionotropic receptors are ion channels. metabotropic receptors are not ion channels; they mostly mediate their effects through g protein signaling cascades. Receptors can be found anywhere, so we often specify their location. Intracellular receptors are located inside the cell. Surface receptors are located on the cell membrane. Surface receptors are further specified as being: Postsynaptic receptors - located on postsynaptic membrane Presynaptic receptors - located on presynaptic membrane Extrasynaptic receptors - located near but outside a synapse 22 TERMINOLOGY Ligand General term for a signaling molecule that binds to a receptor. Neurotransmitters are ligands. Binding site The place on a receptor where a ligand binds Postsynaptic A receptor located on postsynaptic membrane. Receptor Can be ionotropic or metabotropic (most synapses contain both). Ionotropic A ligand-gated ion channel. It is an ion channel that opens receptor in response to ligand binding. Its effects on the membrane potential are very brief and peak within a few milliseconds. Metabotropic A receptor that is not an ion channel. Ligand binding typically triggers receptor an intracellular g protein signaling cascade, which can have diverse effects on cell function. These signaling cascades take time, and effects are usually not evident for at least 100ms if not much longer. 23 24 NEUROTRANSMITTER CLEARANCE Neurotransmitter signaling in the synapse is kept brief by three mechanisms: diffusion, enzymatic deactivation and reuptake. Diffusion Passive movement from areas of high concentration to areas of low concentration Enzymatic Destruction of a neurotransmitter by an enzyme deactivation For example, acetylcholine is broken down in the synaptic cleft by the enzyme acetylcholinesterase Reuptake Reuptake transporters recycle neurotransmitters by pulling them back into the cell that just released them (e.g., the serotonin reuptake transporter). 25 26 POSTSYNAPTIC RECEPTOR ACTIVATION Postsynaptic When a neurotransmitter binds to a postsynaptic receptor and changes potential the membrane potential of the postsynaptic cell. - Ionotropic receptors produce rapid postsynaptic potentials (1 to 5 ms). - Metabotropic receptors do not always produce postsynaptic potentials, but when they do, they are relatively slow/delayed (~100ms to 10s). Beyond being slow or fast, postsynaptic potentials are also excitatory or inhibitory. Excitatory postsynaptic potentials (EPSPs) are the result of positive sodium ions entering the postsynaptic cell, causing membrane depolarization and perhaps an action potential. Inhibitory postsynaptic potentials (IPSPs) are often the result of negative chloride ions entering the cell, causing membrane hyperpolarization and fewer action potentials. 27 CHANGES IN MEMBRANE POTENTIAL Depolarization When the membrane potential of a cell becomes less negative than it normally is at rest. The opening of Na+ ion channels will depolarize a neuron, making it more likely to spike. Hyperpolarization When the membrane potential of a cell becomes more negative than it normally is at rest. The opening of Cl- ion channels can hyperpolarize a neuron, making it less likely to spike. Ionotropic receptors are classified as excitatory or inhibitory based on whether they let in Na+ or Cl- ions and thus cause EPSPs or IPSPs. Metabotropic receptors are similarly classified, based on whether they cause the opening of Na+ or Cl- (g protein-gated) ion channels. Note that some metabotropic receptors open potassium ion channels. Can you guess whether this will have an excitatory or inhibitory influence on the membrane potential? 28 ACTION POTENTIAL GENERATION If only a couple EPSPs occur at the same time, the influx of sodium ions will probably not cause an action potential because anytime sodium ions come in and depolarize the membrane, it causes potassium ions to want to leave. Thus, membrane depolarization (caused by sodium ions entering the cell) is quickly counteracted by an outflow of potassium ions through leak channels. Typically, many EPSPs must occur at nearly the same time to depolarize the membrane to the threshold of activation. Sodium ions must enter the cell at a faster rate than potassium ions leave. Action potentials start at the initial segment of the axon hillock (the beginning of the axon), where there are tons of voltage-gated sodium channels. This is the where the membrane must reach the threshold of activation to trigger an action potential. 29 NEURAL INTEGRATION The interaction of the excitatory and inhibitory synapses on a particular neuron is called neural integration IPSPs decrease the likelihood that the cell will fire. When EPSPs and IPSPs occur at the same time, the influx of negatively charged chloride ions diminish the impact of the positively charged sodium ions. 30 EXCITATION VS INHIBITION Each type of neurotransmitter can activate multiple types of receptors. For example, there are 14 different kinds of serotonin receptors encoded in our genome, even though there is only one kind of serotonin molecule. Most serotonin receptors are inhibitory (i.e., they generate IPSPs), but some are excitatory (i.e., they generate EPSPs). Some cells express excitatory serotonin receptors, while other cells express inhibitory serotonin receptors. Thus, it is the receptor that is expressed by the postsynaptic cell that determines whether a neurotransmitter will be excitatory or inhibitory, not the neurotransmitter itself. However, since we generally know where the different types of receptors are in the brain, we often describe neurons as being excitatory or inhibitory because we know they reliably cause EPSPs or IPSPs in the downstream cells they connect to. 31 NEURAL CIRCUITS When a sensory neuron spikes, it sends this electrical message down its axon to the terminal buttons located in the spinal cord. The release of neurotransmitter from the sensory neuron will depolarize and cause an action potential in an excitatory interneuron, which in turn will active a motor neuron and cause a withdrawal reflex. 32 STRUCTURE AND FUNCTIONS OF CELLS OF THE NERVOUS SYSTEM However, a neuron in the cerebral cortex at the top of the brain may have other plans. And it can send an action potential into the spinal cord to excite an inhibitory interneuron. Release of neurotransmitter from this inhibitory interneuron will hyperpolarize the motor neuron and block (counteract) the withdrawal reflex. This circuit provides an example of a contest between two competing drives: one to drop the hot pan and another to keep holding it. 33 In June of 1963, the Vietnamese Mahayana Buddhist monk Thích Quang Duc burned himself to death at a busy intersection in Saigon. He reportedly burned for 10 minutes without moving or making a sound before collapsing to the ground. 34 NEURAL EXCITATION VERSUS BEHAVIOURAL EXCITATION An inhibitory neuron is a neuron that reliably causes IPSCs in downstream neurons (i.e., it hyperpolarizes and reduces the spiking activity of downstream neurons). Keep in mind that the firing of an inhibitory neuron does not always inhibit behaviour. It can cause a movement. Consider an inhibitory neuron that synapses on other inhibitory neurons. When the first one fires, it will inhibit the firing of downstream inhibitory neurons. Inhibition of inhibitory neurons can generate motor behaviour. The big picture: For every neuron trying to change behaviour in one way, there are other neurons trying to do the opposite. Both types of neurons will often be regulated by inhibitory neurons. And those inhibitory neurons may be controlled by other inhibitory neurons. In fact, we often see long chains of inhibitory neurons in a row, which can make it hard to determine which ones are trying to cause or prevent a behaviour. The take home point is that: The firing of excitatory neurons deep in the brain does not necessarily cause movement, and the firing of inhibitory neurons does not necessarily inhibit movement. For example, a couple of alcoholic drinks often disinhibits behaviour by reducing neural activity in areas of the brain that regulate anxiety and self-control. 35