Lecture 4 Readings PDF
Document Details
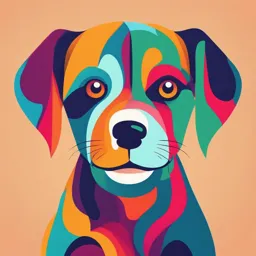
Uploaded by ProperChupacabra
Tags
Related
- Intracellular Membrane Traffic PDF
- Ibn Sina University First Year Biology - Cell Membrane & Transport Lecture Notes PDF
- Trasposto Attraverso Membrane PDF
- General Physiology: Transport Across Cell Membranes - 1 PDF
- Lezione 01 Fisiologia Cellulare - Appunti PDF
- Cell Membrane and Membrane Transport (U3) PDF
Summary
This document discusses diffusion, specifically focusing on the transport of solutes across cell membranes. It explains how the electrical properties of the surrounding environment affect the diffusion process of charged solutes. The document also touches upon the roles of membrane proteins and the hydrophobic nature of cell membranes.
Full Transcript
108 Chapter 5 Figure 5.4 Ionic charge separation Extracellular fluid occurs only within nanometers of –...
108 Chapter 5 Figure 5.4 Ionic charge separation Extracellular fluid occurs only within nanometers of – – + – + + membranes Thus only in the vicinity of Within a few nanometers + + – + – Bulk membranes do conditions exist for the dif- of the membrane on – – + solution – + fusion of charged solutes to be affected by either side, net positive – + + + + + and negative charge con- – – – electrical attraction and repulsion. – centrations may accumu- Farther away, in the late because the lipid + + + + + Cell bulk solution on bilayers in a cell mem- membrane either side, positive brane can maintain sep- (usually 6–8 and negative ions are aration of oppositely – – – – – nm thick) mixed at random. The charged ions. net charge in any + – – + + given region of bulk + + – + solution is zero. – Bulk + – – + – – solution – + + + + + – – – – Cytoplasm Within a few nanometers of a cell membrane or epithelium, the transport through cell membranes takes place through integral solution on one side may have a net positive charge because positive membrane proteins termed ion channels. ions outnumber negative ones. Conversely, within a few nanometers The defining characteristic of ion channels is that they permit of the membrane or epithelium, the solution on the other side may the passive transport of inorganic ions by diffusion through a mem- have a net negative charge because negative ions outnumber posi- brane. This means that the directions and eventual equilibrium tive ones (see Figure 5.4). Under these circumstances the diffusion states of the ion movements through channels are determined not of electrically charged solutes may be greatly affected by electrical by the channels themselves, but by the chemical-concentration and attraction and repulsion. electrical-charge gradients that exist across a membrane. Another key characteristic of channels is that the ions that pass through them Biological aspects of diffusion across do not bind to the channel proteins. Structurally, an ion channel membranes: Some solutes dissolve in consists of one or more protein molecules that extend across the the membrane; others require channels full thickness of a cell membrane or intracellular membrane and Principles we discussed at the start of Chapter 2 return to center that encircle a lipid-free central passageway through the membrane stage when we consider the chemical mechanisms of the diffusion (see Figures 2.4 and 13.16). From this fact and the fact that ions do of solutes through cell membranes or epithelia. The interior of a not bind to channels, one might think that channels are simple cell membrane is hydrophobic because it consists principally of the holes through the membrane. Actually, they are intricate protein hydrocarbon tails of phospholipid molecules composing the lipid structures that are selective in determining which ions can pass bilayer (see Figure 2.1). The ease with which aHill Animal solute diffuse 4E canPhysiology readily through them.6 Some ion channels, for instance, are specific Sinauer Associates directly through the lipid interior of a cell membrane thus depends for Na+. They permit Na+ to move through the cell membrane at a Morales Studio on the hydrophobic or hydrophilic nature of the solute. Figure 05.04 11-05-15 relatively high rate but do not permit rapid passage of other ions. Let’s start by considering lipid solutes, such as steroid hormones Other channels are specific for K+. Still others allow Na+ and K+ to and fatty acids, which are hydrophobic. Molecules of these solutes pass with equal ease. Some channels allow several sorts of ions to dissolve in the lipid interior of a cell membrane. In this dissolved pass, but even the least selective channels discriminate between state, they make their way from the side of the membrane where they anions and cations.7 are more concentrated to the side where they are less concentrated Many ion channels are gated channels, meaning that they can because of molecular agitation and the other principles of simple “open” and “close” because the proteins of which they are composed diffusion we have been discussing. Molecular oxygen (O2), which are able to undergo conformational changes that cause their central is small and nonpolar, is also generally believed to make its way passageways to increase or decrease the ease with which ions pass through cell membranes primarily or exclusively by simple diffu- through (see Figure 12.20). Four categories of gated ion channels, sion through the lipid layers, without the need of channels or other distinguished by the mechanisms of gating, are recognized (Fig- proteins. Certain signaling molecules besides steroid hormones, ure 5.5). Voltage-gated channels open and close in response such as thyroid hormones and nitric oxide, are also believed to cross to changes in the voltage difference across a membrane; they are cell membranes in this way. very important in the generation of nerve impulses. Stretch-gated Inorganic ions present a very different picture because they are (tension-gated) channels open or close in response to stretching hydrophilic and therefore have very low solubilities in membrane or pulling forces that alter the physical tension on a membrane. lipids. Studies using experimental all-phospholipid membranes Phosphorylation-gated channels open or close according to demonstrate that the rates of simple diffusion of ions directly whether the channel proteins are phosphorylated; such channels are through membrane lipids are exceedingly low. However, ions of under the control of protein kinases (see page 55), which are often physiological importance—such as Na+, K+, Ca2+ (calcium), and 6 Cl– —can sometimes move passively through actual cell membranes The ion selectivity of channels is discussed in detail in Chapter 12. at very rapid rates. They are able to do this because their passive 7 Anions are negatively charged ions; cations are positively charged ions. (A) Voltage-gated channel Transport of Solutes and Water 109 Extracellular fluid Closed Open The permeability of a cell membrane to a solute is defined to + + + + – – – – be the ease with which the solute can move through the membrane Change of membrane by diffusion. The concept of permeability applies both to solutes that potential dissolve in the membrane interior and to solutes that pass through channels. When Equation 5.1 is applied to solute diffusion through a membrane, the membrane permeability to the solute of interest helps – – – – + + + + determine D, the diffusion coefficient. For solutes, such as lipids and O2, that dissolve in the lipid bilayer to pass through a membrane, Cytoplasm the permeability of a cell membrane depends on the factors that affect solute diffusion through lipid, such as the molecular size of (B) Stretch-gated (tension-gated) channel the solute. For inorganic ions, the permeability of a cell membrane depends on the number of channels per unit of membrane area and on the proportion of the channels that are open. Cell membranes are often described as selectively permeable because they permit Stretch some solutes to pass through by diffusion with greater ease than others. One explanation for selective permeability is that different sorts of ion channels are often unequally present. For example, if a membrane has a high density of Na+ channels and a low density Cytoskeleton of Ca2+ channels, it is more permeable to Na+ than to Ca2+. Diffusion of ions across cell membranes (C) Phosphorylation-gated channel is determined by simultaneous concentration and electrical effects The final aspect of the mechanism of simple diffusion that we need to consider is the manner in which concentration effects and Phosphorylation electrical effects interact in affecting the diffusion of ions and other charged solutes. We have already seen that, in animal cells, there is a concentration gradient across the cell membrane for each major solute (see Figure 5.1A). The diffusion of each solute is affected 2– PO4 by its own concentration gradient but not by the concentration gradients of other solutes. All cell membranes also exhibit charge separation. That is, there is always an electrical gradient across (D) Ligand-gated channel a cell membrane, defined to be the difference in voltage (electrical Ligand Receptor potential) between the two sides.8 The electrical gradient affects site the diffusion of all charged solutes. It is obvious that the diffusion of an ion or other charged solute must depend on both that solute’s concentration gradient and the electrical gradient. A problem that soon arises in trying to quantify Ligand binding this dual effect is that concentration gradients and electrical gradients are expressed in different units: molarity and volts, respectively. Walther Nernst (1864–1941), an early winner of the Nobel Prize, goes down in history for solving this problem. Using his Nernst equation (discussed in Chapter 12), investigators can directly com- pare concentration and electrical gradients to determine which is Figure 5.5 Gated ion channels Gated ion channels are clas- stronger, and thus predict the diffusion of an ion. sified into four functional categories based on which property must change for them to open and close: (A) transmembrane voltage, (B) Here we take a more informal approach to understanding the stretch or tension, (C) phosphorylation, or (D) noncovalent binding dual effects of concentration and electrical gradients on an ion’s with ligands at receptor sites. diffusion across a cell membrane. We term the influence of the concentration gradient across the membrane on the ion’s diffusion the concentration effect on the ion, and we term the influence of themselves controlled by second messengers (see pages 66–67). the electrical gradient on the ion’s diffusion the electrical effect Hill Animal Physiology 4E Associates channels, discussed in Chapter 2 (see page 63), Ligand-gated Sinauer on the ion. act both Morales as receptors of extracellular signals and as ion channels. Studio Sometimes the electrical charge difference across a membrane They Figure are closed 05.05 to the passage of ions in the absence of a signal, 11-05-15 pulls an ion in the same direction as the ion is moving because of but they open when their receptor sites bind to receptor-specific 8 A truly formal definition of the electrical gradient is that it is the difference ligands (e.g., neurotransmitter molecules), thereby allowing the in voltage divided by the distance between the high and low voltage levels. passage of selected ions. Less formally, the electrical gradient is simply the difference in voltage. 602 Chapter 23 Tidal gas exchange 100 60 O2 partial pressure in environment O2 from each volume of water it pumps and is allowing the other Inhalation Exhalation 67% to flow out with the exhaled water. Fresh medium mixes with stale medium in Tunas are especially efficient in using the O2 in the water they the lung, so the O2 drive over their gills. Whereas rainbow trout use 33%, yellowfin partial pressure of the Wall of lung and skipjack tunas use 50%–60%. medium at the ex- change surface with …the O2 partial pres- the blood is below Medium The O2 partial pressure in blood leaving a that in the environ- sure in blood leaving the lung remains breathing organ depends on the spatial mental medium. lower than that in relation between the flow of the blood and the exhaled medium. the flow of the air or water O2 diffuses into the 60 blood flowing along The O2 partial pressure in the blood leaving a breathing organ can in the exchange surface. 30 50 55 many ways be considered the best single measure of the breathing The O2 partial pressure organ’s effectiveness. This is clear from the oxygen cascade concept in the blood rises, 0 Blood therefore, toward that (see Figure 22.8B): An animal with a high O2 partial pressure in the in the lung, but… blood leaving its breathing organ is particularly well poised to maintain an O2 partial pressure in its mitochondria that is sufficiently high for Figure 23.3 Tidal gas exchange: O2 transfer from the en- aerobic catabolism to proceed without being O2-limited. vironmental medium to the blood in a tidally ventilated Breathing organs with different designs exhibit inherent differences lung Because a tidally ventilated lung is never fully emptied, fresh in the blood O2 partial pressure they can maintain. These differences medium mixes in the lung with stale medium. Numbers are O2 partial depend on the spatial relation between the flow of blood and the flow pressures in arbitrary units: The blood arriving at the breathing organ of the air or water. The differences are not absolute, because in all is arbitrarily assigned a value of 0, whereas the atmosphere is arbi- trarily assigned a value of 100. designs, the blood O2 partial pressure is affected by additional factors besides spatial flow relations. Nonetheless, the flow relations between the blood and the air or water have great importance. To explore the implications of various designs, let’s start by meets fresh, incoming medium, as shown at the left of Figure 23.4A. considering tidally ventilated breathing organs, such as the lungs Then, as the blood and medium flow along the exchange membrane of mammals (Figure 23.3). Tidally ventilated breathing organs are in the same direction, they gradually approach equilibrium with distinguished by the fact that the medium (air in this case) next to each other at an O2 partial pressure that is intermediate between the gas-exchange membrane is never fully fresh. The explanation their respective starting partial pressures. When the blood reaches is that such breathing organs are never entirely emptied between the place where it leaves the exchange membrane, its final exchange breaths. Consequently, when an animal breathes in, the fresh medium of O2 is with medium that has a partial pressure considerably inhaled mixes—inside the breathing organ—with stale medium left below that of Hill Animal the environmental Physiology 4E medium. Cocurrent gas exchange behind by the previous breathing cycle, and because of this mixing, Sinauer Associates therefore resembles tidal exchange, in that the O2 partial pressure Morales Studio the O2 partial pressure of the medium next to the gas-exchange of blood leaving the breathing organ cannot ordinarily rise above Figure 23.03 12-09-15 membrane is lower—often much lower—than the partial pressure the partial pressure of exhaled medium. in the outside environment. The O2 partial pressure in the blood In an organ that exhibits countercurrent gas exchange, when leaving the breathing organ is lower yet (see Figure 23.3), because O2-depleted afferent blood first reaches the gas-exchange mem- a partial-pressure gradient must exist between the medium next to brane, it initially meets medium that has already been substantially the gas-exchange membrane and the blood for O2 to diffuse from deoxygenated, as shown at the right of Figure 23.4B. However, as the medium into the blood. Characteristically, in tidally ventilated the blood flows along the exchange surface in the direction opposite structures, the O2 partial pressure of the blood leaving the breathing to the flow of medium, it steadily encounters medium of higher organ is below the O2 partial pressure of the exhaled medium. and higher O2 partial pressure. Thus, even as the blood picks up When ventilation is unidirectional rather than tidal, the two most O2 and its partial pressure rises, a partial-pressure gradient favor- obvious relations that can exist between the flow of the medium ing further uptake of O2 is maintained. The final exchange of the and the flow of the blood are cocurrent and countercurrent. In the blood is with fresh, incoming medium of high O2 partial pressure. cocurrent arrangement (Figure 23.4A), the medium flows along Countercurrent exchange is thus an intrinsically more effective mode the gas-exchange membrane in the same direction as the blood, of exchange than either tidal or cocurrent exchange. One way to resulting in cocurrent gas exchange. In the countercurrent ar- see this clearly is to note that the blood O2 partial pressure created rangement (Figure 23.4B), the medium and blood flow in opposite by countercurrent exchange is characteristically much higher than directions, and countercurrent gas exchange occurs. Concurrent the partial pressure in exhaled medium; in principle, the O2 partial is sometimes used as a synonym of cocurrent. Thus cocurrent gas pressure of the blood leaving the breathing organ might even ap- exchange may also be called concurrent gas exchange. proach equality with the O2 partial pressure in inhaled medium. In an organ that exhibits cocurrent gas exchange, when O2- Moreover, if you compare Figures 23.4A and B, you will see that depleted afferent3 blood first reaches the gas-exchange membrane, it the O2 partial pressure of the medium falls more—and that of the 3 blood rises more—in 23.4B: Countercurrent exchange achieves a Afferent means “flowing toward”; in this case it refers to blood flowing toward the gas-exchange membrane. Efferent means “flowing away” and more complete transfer of O2 from the medium to the blood than refers to blood flowing away from the gas-exchange membrane. cocurrent (or tidal) exchange under comparable conditions. (A) Cocurrent (concurrent) gas exchange External Respiration 603 Medium 100 70 56 52 Cross-current gas exchange 0 30 44 48 65 Blood 100 As blood and medium 89 77 65 53 41 Medium flow in the same direction, they gradually 100 74 48 35 O2 partial pressure Medium approach equilibrium with each other. 50 0 Blood Blood Figure 23.5 Cross-current gas exchange: A third mode of 0 O2 transfer from the environmental medium to the blood when ventilation is unidirectional Numbers are O2 partial pressures in arbitrary units, as specified in the caption of Figure 23.3. Distance along The afferent blood vessel breaks up into many vessels that “cross” exchange surface the path followed by the medium; each of these vessels makes exchange contact with just a limited part of the structure through (B) Countercurrent gas exchange which the medium is flowing. These vessels then coalesce to form Medium a single efferent vessel. Cross-current exchange is intermediate 100 75 50 25 between cocurrent and countercurrent exchange in its intrinsic gas- 75 50 25 0 transfer efficiency. Blood 100 As blood flows in the direction opposite to the exchange. As already noted, however, this ranking is not absolute medium and picks up O2, O2 partial pressure it steadily encounters because additional factors affect the ways in which real breathing 50 Medium medium of higher O2 organs function in real animals. partial pressure, so that a partial-pressure gradient Arterial CO2 partial pressures are much lower Blood favoring O2 diffusion into the blood is maintained. in water breathers than air breathers 0 Thus far we have discussed only O2 in the blood leaving the gas- exchange surface. What about CO2? The most important pattern in blood CO2 partial pressure is a distinction between water and Distance along exchange surface air breathers. In water breathers, the partial pressure of CO2 in the blood leaving the breathing organs is always similar to the CO2 partial Figure 23.4 Cocurrent and countercurrent gas exchange: pressure in the ambient water, regardless of whether gas exchange Two modes of O2 transfer from the environmental medium Hill Animal Physiology 4E isSinauer tidal, Associates cocurrent, countercurrent, or cross-current. If the ambi- to the blood when ventilation is unidirectional The upper ent water Morales is near equilibrium with the atmosphere and therefore Studio diagram in each case depicts the flow of medium and blood along the gas-exchange membrane. Numbers are O2 partial pressures in near zero Figure in its 23.05 CO2 partial pressure (see Figure 22.1), the blood is 12-09-15 arbitrary units, as specified in the caption of Figure 23.3. The blood also near zero in CO2 partial pressure. For example, in fish in aer- reaches a higher O2 partial pressure when the exchange is counter- ated water, the partial pressure of CO2 in blood leaving the gills current because in that case the blood exchanges with fresh me- is typically about 0.3 kilopascals (kPa) (2 millimeters of mercury dium just before leaving the gas-exchange membrane. [mm Hg]). In sharp contrast, in air breathers the partial pressure of CO2 in the blood leaving the breathing organs is usually far above the When ventilation is unidirectional, a third possibility is cross- CO2 partial pressure in the atmosphere. In mammals and birds, for current gas exchange. In this type of exchange, the blood flow example, the partial pressure of CO2 in blood leaving the lungs is Hill Animal Physiology 4E breaks Sinauer up into multiple streams, each of which undergoes exchange Associates more than ten times the corresponding value in fish; in humans with theStudio Morales medium along just part of the path followed by the medium it is 5.3 kPa (40 mm Hg)! In vertebrates, systemic arterial blood (Figure Figure23.0423.5 ). Some blood therefore undergoes gas exchange 12-09-15 pumped by the heart to supply O2 to the body is derived from the exclusively with O2-rich medium (although other blood exchanges blood leaving the breathing organs. Thus, from what we have said, with O2-poor medium). Cross-current exchange permits the O2 if we survey species in which arterial CO2 partial pressure has been partial pressure of the mixed blood leaving the breathing organ to measured, we can understand why the arterial CO2 partial pres- be higher than that of exhaled medium, but it does not permit as sure is typically only 0.1–0.6 kPa (1–4 mm Hg) in water-breathing high a blood O2 partial pressure as countercurrent exchange under vertebrates, whereas it is far higher, 4.0–5.3 kPa (30–40 mm Hg), comparable circumstances. in air-breathing vertebrates. When vertebrates and other animals The modes of exchange between the blood and the medium can emerged onto land in the course of evolution, the level of CO2 in their be ranked in terms of their intrinsic ability to create a high O2 partial blood and other body fluids shifted dramatically upward. pressure in the blood leaving the breathing organ (corresponding These patterns are explained principally by the differing physical to their intrinsic efficiency in transferring O2 from the medium and chemical properties of water and air. More specifically, they are to the blood): Countercurrent exchange is superior to cross-current explained by the analysis of capacitance coefficients, as explained exchange, and cross-current exchange is superior to cocurrent or tidal in Box 23.1. 604 Chapter 23 BOX Capacitance Coefficients Explain the Relative Hypoxia-inducible factors 1 and 2 (HIF-1 23.1 Partial Pressures of O and CO in Arterial Blood and HIF-2) are the most central players in the 2 2 intracellular responses. They are closely related During breathing, the extent to which medium (water or air), the capacitance transcription factors, which bind with specific the CO2 partial pressure of the respired coefficient is defined to be the change hypoxia-response elements (nucleotide base medium changes relative to its change in total gas concentration in the respired sequences) of DNA to activate gene transcrip- in O2 partial pressure tends to be sharply medium per unit of change in gas par- tion. In the last two decades there has been different between water breathers and tial pressure in the respired medium. Box an explosion of knowledge regarding these air breathers because of a property of Extension 23.1 explains by use of a fig- transcription factors, which are implicated in water and air called the capacitance ure how capacitance coefficients place many disease states as well as playing crucial coefficient. For any particular gas (O2 constraints on animal physiology. roles in the ordinary lives of animals. HIF-1 or CO2) and any particular respired is an ancient molecule, identified in inverte- brates as well as in all groups of vertebrates. HIF-1 and HIF-2 increase in concen- Summary tration in a cell when the cell experiences Principles of Gas Exchange by Active Ventilation hypoxia, and they affect the transcription of dozens or hundreds of target genes, which have been identified through transcription The oxygen utilization coefficient during breathing is the percentage of the O2 in inhaled medium that an animal profiling (transcriptomics) (see Chapter 3). HIF-1 and HIF-2 are removes before exhaling the medium. different in their effectiveness in activating various genes in a tissue. Moreover, their target genes differ to some extent from tissue to tissue The four major types of gas exchange that can occur during directional ventilation can be ranked within a single species, and they also differ among species. Overall, in terms of their inherent ability to establish a high O2 HIF-1 and HIF-2 have extremely broad ranges of potential action, partial pressure in blood exiting the breathing organ. and many of their actions aid cells that are confronting hypoxia. In Countercurrent gas exchange ranks highest. Cross- vertebrates they cause certain types of cells to increase secretion of current gas exchange ranks second. Cocurrent and erythropoietin, which enhances production of red blood cells (see tidal gas exchange rank third. Box 24.2). They also increase intracellular synthesis of several types Air breathers tend to have much higher CO 2 partial of molecules, including enzymes of anaerobic glycolysis and a form pressures in their systemic arterial blood than water of mitochondrial cytochrome oxidase that is particularly efficient in breathers. This difference arises during breathing and is using O2. Besides these effects and many others, they also promote principally a consequence of the differing physical and angiogenesis, the development of new blood capillaries. The increase chemical properties of air and water. in capillaries shortens the average distance between tissue cells and capillaries, thereby aiding O2 diffusion into the cells. Low O2: Detection and Response The basic manner in which the intracellular concentrations of these transcription factors are regulated is exemplified in Figure Most animals are fundamentally aerobic. Thus when the O2 levels 23.6 using HIF-1. A complete HIF-1 molecule consists of α and β in an animal’s tissues become unusually low, the low O2 levels typi- subunits. The β subunits are present regardless of cellular O2 level. cally mean trouble. Animals detect low O2 levels—and respond Moreover, the α subunits are constantly being not only synthesized to them—at two different scales. The mechanisms involved in but also tagged for breakdown by the ubiquitin–proteasome system detection and response are elaborate. Their elaborateness reflects (see Figure 2.23). When the O2 level in a cell decreases, breakdown the extreme importance of O2. of α subunits is inhibited. Therefore the concentration of α subunits One scale of response to low O2 is that of the whole body: the scale of organ systems. In many types of animals, the O2 partial pressure in the circulating blood is monitored continuously, and if the blood β O2 partial pressure falls, the function of the breathing system is Synthesis modulated to reverse this trend. Water breathers, for example, of α subunits Nuclear envelope commonly respond to a decreased blood O2 partial pressure by α α β increasing their rate of gill ventilation. Air breathers may increase α β DNA their rate of lung ventilation by breathing at a higher rate. Responses Breakdown such as these—which we will discuss often in this chapter—help of α subunits HIF-1 protect an animal’s cells from experiencing hypoxia.4 The second scale of response is intracellular, in cells throughout the Hypoxia-response body. The intracellular responses are elicited if the rate at which …promoting element Low intracellular dimerization of the breathing and circulatory systems supply O2 fails to keep pace O2 inhibits break- β subunits with with the rate of mitochondrial O2 utilization—resulting in cellular HIF-1 enters the nucleus down of α sub- α subunits to form and combines with hypoxia- hypoxia. units, thereby… HIF-1. response elements in DNA. 4 Hypoxia, as mentioned in Chapter 8, refers to an especially low level of O2 in tissues or cells. Figure 23.6 Formation and action of HIF-1 3.0 External Respiration 605 Lived steadily in aerated water Experienced hypoxia 2.5 to a major role for the HIF signaling system in allowing these people to succeed. The two scales of response to low O2 that we noted at the start of Relative messenger RNA expression this section in fact interact with each other. As already mentioned, 2.0 for example, in vertebrates HIF-1 and HIF-2 play central roles in controlling the production of red blood cells. In this way, HIF-1 and HIF-2 help control the O2-carrying ability of each unit of volume of 1.5 blood, which is a major factor at the scale of organ systems. Introduction to Vertebrate Breathing 1.0 The vertebrates living today are usually thought of, in a rough way, as representing an evolutionary sequence. In actuality, of course, today’s fish were not the progenitors of today’s amphibians, and 0.5 today’s lizards (or other nonavian reptiles) were not the progenitors of today’s mammals and birds. Thus when we think of the sequence from fish to mammals and birds, caution is always called for in think- 0.0 ing of it as an evolutionary sequence. Comparisons among today’s hif-1 hif-2 phd1 phd2 fih animals nonetheless often provide revealing insights into trends Gene studied that occurred during evolution. Before we start our study of breath- Figure 23.7 A genomic assessment of acclimation in the ing in the various vertebrate groups, an overview of general trends HIF signaling system Expression of five genes that code for will help place the individual vertebrate groups in a larger context. compounds in the HIF signaling system was measured in the heart in One property of breathing organs that is of enormous impor- two groups of epaulette sharks (Hemiscyllium ocellatum). One group lived continuously in aerated water. The other experienced eight tance is the total surface area of the gas-exchange membrane. This brief (2-h) episodes of hypoxic water over a period of 4 days. Expres- area is typically an allometric function of body size among species sion is measured on a relative scale. For each group, the upper and within any one group of phylogenetically related vertebrates. The lower box limits correspond to the 75th and 25th percentiles of the allometric relation differs, however, from one group to another, as data, the line in the box marks the median, and the bars extending seen in Figure 23.8A. To explore Figure 23.8A, let’s first focus on from the box show the data range. The differences between the two the lines for amphibians and reptiles other than birds. These lines groups were statistically significant for the hif-1 gene (which codes for are similar. Moreover, the lines for most groups of fish (e.g., toadfish HIF-1) and the phd2 gene. (After Rytkönen et al. 2012.) and dogfish) are also similar. These similarities tell us two things. First, the total lung area of a nonavian reptile is roughly similar to rises, and more HIF-1 molecules are formed by dimerization of α the total lung area of an amphibian of the same body size. Second, and β subunits. The HIF-1 molecules enter the nucleus, where they the total gill area of a fish of particular body size is roughly similar bind with specific DNA sequences (the hypoxia-response elements), to the total lung area of an amphibian or reptile of that size—sug- which in turn Hill Animal control4E Physiology gene transcription. gesting that when vertebrates emerged onto land, there was not Sinauer SomeAssociates species of animals are well adapted to living in environ- immediately much of a change in the area of the gas-exchange Morales Studio ments Figurewhere 23.07 the ambient1/12/16 12-16-15 O2 level is prone to fall to low levels. For surface in their breathing organs. If we now turn our attention to the example, the epaulette shark (Hemiscyllium ocellatum) lives in places lines for mammals and birds, we notice that those groups, although where it experiences periods of very low environmental O2. In a independently evolved, are similar to each other. Moreover, they recent genomic study, researchers asked whether the HIF signaling exhibit a dramatic step upward in the area of gas-exchange surface system undergoes acclimation when fish that have been living in in their lungs by comparison with amphibians or nonavian reptiles. high-O2 water begin to experience low O2. One can reason that if a In brief, the mammals and birds independently evolved lungs fish that’s been living in high-O2 water is about to confront a period with markedly enhanced gas-exchange surface areas—probably of low environmental O2, the fish might profit by upregulating its in association with their evolution of homeothermy. As we saw in HIF-1 signaling system, recognizing that it will depend on the HIF Chapter 10, homeothermy increases an animal’s metabolic rate by a signaling system to help its tissues cope with the hypoxia they may factor of at least four to ten. Thus lungs with an enhanced ability to soon experience. Using genomic methods, researchers measured take up O2 and void CO2 are required by homeothermic animals. the expression in the heart muscle of five genes that code for HIF-1 Remarkably, tunas—noteworthy for being extremely active fish, as and other compounds in the HIF signaling system. Sharks that discussed at the opening of this chapter—have gill surface areas were exposed to a series of eight brief episodes of low O2 in their that approximate the lung surface areas of equal-sized mammals environment showed upregulation of expression of some of these and birds! genes, compared with sharks that lived in high-O2 water all the The fact that mammals and birds have exceptionally large time (Figure 23.7). gas-exchange surface areas does not mean that they have large Some human cultural groups live at high altitudes, where they lungs compared with reptiles or amphibians. In fact, the opposite steadily experience low atmospheric O2 concentrations. As we will is often true. For example, if a rodent is compared with a like-size discuss later in this chapter (see Box 23.2), recent evidence points lizard, snake, or turtle, the lung volume of the reptile is likely to be (A) Area of the gas-exchange membrane vs. body size (B) Thickness of the gas-exchange membrane vs. body size Dogfish 10 50,000 Rainbow Thickness (µm) on log scale Toadfish 5 trout Crocodile Yellowfin tuna Yellowfin tuna Area (cm2) on log scale 10,000 Monitor Lacerta 1 lizard lizard Turtle 5000 Dogfish 0.5 Mammals Reptiles Birds Mammals other than Snake Teju lizard birds Birds 1000 Amphibians 0.1 10 50 100 500 1000 5000 10,000 500 Rainbow trout Body weight (g) on log scale Ray KEY Toadfish Mammals Amphibians 100 Birds Fish Reptiles other 10 50 100 500 1000 5000 10,000 than birds Body weight (g) on log scale Figure 23.8 Total area and thickness of the gas-exchange membrane in the gills or lungs of vertebrates as functions of body size The lines for mammals and birds in both (A) and (B) and those for amphibians and reptiles other than birds in (A) are for many species (e.g., about 40 species of mammals, ranging in size from shrews to horses). The lines for fish in (A) are for various-sized Brown trout individuals of single species. The thickness in (B) is the average dis- Salmo trutta tance between the blood and the water or air. (After Perry 1990.) Goldfish Carassius carassius Flatfish Pleuronectes platessa at least five times greater than that of the rodent. Yet the surface area of the gas-exchange membrane is likely to be ten times greater European eel Anguilla anguilla in the rodent than in the reptile! The explanation for the high gas- Mudpuppy exchange surface area in the lungs of a mammal or bird is that Necturus maculosus the lungs of these animals are extraordinarily densely filled with Bullfrog (larva) branching and rebranching airways, as we will see in this chapter. Rana catesbeiana In nonavian reptiles and amphibians, in contrast, the lungs typi- Bullfrog (adult) cally have parts that are simply like balloons—little more than a Rana catesbeiana sheet of tissue surrounding an open central cavity—and even the Lungless salamander parts that are subdivided are much less elaborately subdivided than Ensatina eschscholtzii mammalian or avian lungs. Thus, whereas the lungs are large in Boa constrictor a reptile compared with a rodent, they provide the reptile with a Constrictor constrictor comparatively small area of gas-exchange membrane. Southern musk turtle The thickness of the barrier between the blood and the envi- Sternotherus minor ronmental medium also shows significant evolutionary trends in Red-eared turtle Trachemys scripta the major vertebrate groups. In most fish, the sheet of gill tissue between blood and water is roughly 5–10 μm thick. Vertebrate Green lizard Lacerta viridis lungs uniformly have a much reduced barrier between blood and Chuckwalla lizard air (Figure 23.8B). Mammals tend to have a thinner sheet of tissue Sauromalus obesus between blood Hill Animal and air4E Physiology than lizards, crocodilians, or other nonavian Big brown bat Sinauer Associates reptiles. To a dramatic extent, the tissue in birds is thinner yet, Eptesicus fucus O2 Morales Studio roughly 0.2 μm. Tunas, which are highly specialized for O2 uptake Figure 23.08 12-09-15 Human CO2 compared with most fish, have a blood–water barrier in their gills Homo sapiens that is similar in thickness to the blood–air barrier in mammals. 0 10 20 30 40 50 60 70 80 90 100 The skin varies widely in its role as a gas-exchange site in ver- Gas exchange through skin (%) tebrates (Figure 23.9). Some fish and most reptiles have evolved Figure 23.9 The percentage of O2 and CO2 exchange that skins that permit little O2 or CO2 exchange. In contrast, the skin occurs across the skin in vertebrates The exact extent of skin can be responsible for 25% or more of gas exchange in other fish, in breathing within a species often depends on environmental condi- certain snakes and turtles, and in many amphibians; in the lungless tions (e.g., temperature). Some authorities place the bullfrog in the salamanders, which not only lack lungs but have an epidermis that genus Lithobates. (After Feder and Burggren 1985.) In the intact preparation, However, after the prepara- External Respiration 607 rhythmic motor impulses tion was severed into two were detected in all the parts, the nerves posterior nerves studied. to the cut went silent. Figure 23.10 A central pattern generator in the brainstem originates motor-neuron impulses that produce the breathing (A) Intact (B) Severed rhythm An isolated portion of the central Hypoglossal cranial nerve nervous system of a neonatal rat, cut free from all connections with the rest of the animal, was Brainstem used for this study. It included the brainstem and spinal cord. Recording electrodes were Glossopharyngeal cranial nerve attached to two cranial nerves (hypoglossal and glossopharyngeal) and the ventral roots of When the preparation three spinal nerves: C4 and C5 (C = cervical) was severed into two and T6 (T = thoracic). Records show electrical parts, the cut was C4 activity as a function of time. (From Feldman et made here (equivalent al. 1988.) to a high neck fracture). C5 T6 stops instantly, because the neuronal outputs from the brainstem are unable to travel to the breathing muscles by way of spinal nerves. 5 sec Experiments that illustrate these vital points have been carried out on the type of preparation shown at the left Ventral roots of spinal nerves in Figure 23.10, consisting of the brainstem and spinal cord of a young rodent, isolated from the rest of the body. The medulla of the brain, isolated in this preparation from any sensory neuronal input, endogenously generates rhythmic bursts of ventilation-driving motor-neuron impulses that are detectable in multiple cranial and spinal nerves (see Figure 23.10A). However, if the spinal cord is severed (see Figure 23.10B), the spinal nerves posterior to the is vascularized (highly unusual), 100% of gas exchange occurs across injury go silent, paralyzing the ventilatory muscles they service.5 the skin. Most mammals and birds resemble humans (see Figure Humans and most other mammals exhibit continuous breath- 23.9) in relying almost entirely on their lungs. Among terrestrial ing, meaning that each breath is followed promptly by another vertebrates, the role of the skin in breathing is related to the skin’s breath in a regular, uninterrupted rhythm. Birds and most fish desiccation resistance. Groups of terrestrial vertebrates that are well also usually display continuous breathing. Lizards, snakes, turtles, defended against water loss through their skin, such as mammals crocodilians, amphibians, and air-breathing fish, in contrast, usually and birds, tend to exhibit little exchange of O2 or CO2 through exhibit intermittent breathing, or periodic breathing, defined their skin, whereas groups, such as frogs and salamanders, that to be breathing in which breaths or sets of breaths are regularly are poorly defended against cutaneous water loss tend to engage interrupted by extended periods of apnea —that is, periods of in significant cutaneous breathing. Modifications that render the no breathing. During intermittent breathing in these vertebrates, skin poorly permeable to water (see page 771) also make it poorly each period of apnea follows an inspiration. The lungs, therefore, permeable Hill Animal Physiologyto 4EO2 and CO2. are inflated during apnea. The glottis—the opening of the airways Sinauer Associates The control of the active ventilation of the gills or lungs is the into the buccal cavity—is closed during apnea in the groups of Morales Studio final subject that deserves mention in introducing vertebrate breath- vertebrates that display intermittent breathing. Because of this Figure 23.10 12-09-15 ing. Of central importance is the control of the rhythmic muscle glottal closure, the inspiratory muscles can relax during the apnea contractions that produce breathing movements, such as our own without causing air to be expelled from the lungs. inhalation and exhalation movements. The muscles responsible for breathing movements in all vertebrates are skeletal muscles. They Summary therefore require stimulation by motor neurons for each and every Introduction to Vertebrate Breathing contraction they undergo. The breathing rhythm originates in sets of neurons that form central pattern generators (see Chapter 19); The gill surface area of most fish of a given body size in a process called rhythmogenesis, these sets of neurons initiate is similar to the lung surface area of amphibians and rhythmic outputs of nerve impulses (action potentials) that travel nonavian reptiles of the same size. Compared with the to the breathing muscles and stimulate them into rhythmic pat- latter groups, mammals and birds have much more lung surface area—helping to meet their far higher needs terns of contraction. The central pattern generators for breathing for gas exchange. The barrier between the blood and in all vertebrates are believed to be located in the brainstem: in the air or water in the breathing organs is notably thin in the medulla, and sometimes other associated parts, of the brain. mammals and thinnest in birds. Although outside influences routinely play roles in controlling (Continued ) breathing, these central pattern generators are absolutely essential for creating the breathing rhythm. Accordingly, if a vertebrate’s 5 More information on rhythmogenesis is presented later in this chapter in spinal cord is severed just posterior to the brainstem, breathing the discussion of mammals. External Respiration 621 ventilation is also modulated by conscious con- these variables simultaneously because the time needed for one trol, lung mechanosensors, and direct effects of breathing cycle tends to increase as the tidal volume increases. exercise The most obvious type of modulation of ventilation Nonetheless, during vigorous exercise, trained athletes are able to in humans is conscious control. We can temporarily stop breathing maintain a tidal volume of at least 3 L while breathing at least 30 by choosing to stop. There are, in addition, other types of control times per minute. In this way, their respiratory minute volume can besides the chemosensory ones. reach greater than 100 L/min—more than 15 times the resting value. One well-understood set of controls is based on mechanore- The alveolar ventilation rate, typically expressed as the ceptors in the lungs, which sense stretch or tension in the airways. alveolar minute volume, is defined to be the rate at which new air Information from these receptors is relayed via sensory neurons to is brought into the alveoli and other respiratory airways. This rate the brainstem, where signals for inhalation tend to be inhibited by is important because the air that reaches the respiratory airways is lung expansion and excited by lung compression. Certain of these the air that can undergo gas exchange with the blood. The alveolar mechanosensory responses are known as the Hering-Breuer reflexes. minute volume is calculated by subtracting the volume of the It is now well established that during exercise there are controls anatomical dead space, VD , from the tidal volume and multiplying operating in addition to chemosensory ones. Whereas these other by the breathing frequency: controls are important, they are not well understood. As already Alveolar minute volume = (VT – VD ) × f (23.3) stressed, the arterial partial pressures of O2 and CO2 remain little changed from resting values during light to moderate exercise. This Another property of importance relating to the respiratory airways stability results because of adjustments in the ventilation rate, which is the fraction of all inhaled air that reaches them. This fraction— is increased in tandem with the metabolic rate. However, arterial calculated by dividing the alveolar minute volume (Equation 23.3) gas partial pressures are far too stable during light to moderate by the total minute volume (Equation 23.2)—is (VT – VD)/VT. exercise to account for observed increases in ventilation on the basis From the expression just described, you can see that—with VD of the simple chemosensory negative feedback systems we have assumed to be constant—the fraction of air reaching the respiratory discussed up to here; for example, whereas an increase of about airways increases as the tidal volume increases. This fact helps 0.5 kPa (4 mm Hg) in the arterial CO2 partial pressure is required resolve a paradox. When the overall ventilation rate is increased, to bring about a doubling of ventilation rate, the measured CO2 the oxygen utilization coefficient increases. Although humans, for partial pressure during exercise may not be elevated to that extent example, use about 20% of the O2 in the air they breathe when their even when ventilation has reached 10–15 times the resting rate! In tidal volume is 500 mL, they use about 30% when their tidal volume addition to the controls mediated by gas partial pressures, there is is 2000 mL. How is this possible if, as we have often emphasized, increasing evidence for the existence of controls that are initiated in the control systems typically keep the alveolar O2 partial pressure direct association with the muscular movements of exercise. These constant? The paradox is resolved by recognizing two aspects controls are postulated to take two forms: First, parts of the brain of gas exchange. First, air that reaches the respiratory airways that initiate motor signals to the exercising muscles might simultane- always gives up about the same fraction of its O2 (accounting for ously initiate stimulatory signals to the breathing centers. Second, the constancy of alveolar O2 partial pressure). Second, however, a sensors of movement or pressure in the limbs might stimulate the greater proportion of all the air that is breathed actually enters the breathing centers based on the vigor of the limb activity they detect. respiratory airways as the tidal volume increases. One persuasive piece of evidence for these sorts of controls is that when people suddenly begin to exercise at a moderate level, their In species of different sizes, lung volume tends ventilation rate undergoes a marked increase within just one or two to be a constant proportion of body size, but breaths; this response is far too rapid to be mediated by changes breathing frequency varies allometrically in the chemical composition of the body fluids. Another piece of If we look at the full range of mammals, ranging in size from shrews evidence is that in many species of mammals, breathing movements to whales, there is a strong inverse (and allometric) relation between and limb movements are synchronized during running. breathing frequency and body size. Humans breathe about 12 times per minute at rest. A mouse breathes 100 times per minute! both tidal volume and breathing frequency are This dramatic effect is a logical consequence of several facts. First, modulated by control systems The overall rate of lung lung volume tends, on average, to be a relatively constant fraction ventilation depends on two properties: the tidal volume, VT , and the of total body volume: Lung volume in liters averages about 6% frequency of breaths, f, usually expressed as the number of breaths of body weight in kilograms. Second, resting tidal volume tends per minute. The product of these is the respiratory minute volume: consistently to be about one-tenth of lung volume or 0.6% of body weight. Third, related to these points, when mammals of all sizes Respiratory minute volume = VT × f (23.2) are at rest, the amount of O2 they obtain per breath is approximately (mL/min) (mL/breath) (breaths/min) a constant proportion of their body weight. However, as emphasized To illustrate, resting humans have a tidal volume of about 500 mL in Chapter 7, the resting weight-specific rate at which mammals and breathe about 12 times per minute. Thus their respiratory metabolically consume O2 increases allometrically as body size minute volume is about 6 L/min. decreases. If the O2 demand per unit of body weight in a small Both tidal volume and breathing frequency are increased during mammal is greater than that in a large mammal, and yet the small exercise and during other states that increase the rate of metabolism. animal obtains about the same amount of O2 per breath per unit Humans and other mammals, however, cannot maximize both of of weight, then the small animal must breathe more frequently. 622 Chapter 23 Pulmonary surfactant keeps the alveoli to the origins of vertebrate air breathing. The roles of pulmonary from collapsing surfactants in animals other than mammals are incompletely known The alveoli may be thought of as aqueous bubbles because their but are gradually being better understood. gas-exchange surfaces are coated with an exceedingly thin water layer. If the alveoli were composed only of water, they would fol- Summary low the physical laws of simple aqueous bubbles. One such law is Breathing by Mammals that the tendency of a bubble to collapse shut increases as its ra- dius decreases.8 Thus, during exhalation, there is a risk that as the The lungs of mammals consist of dendritically radius of an alveolus decreases, the alveolus might collapse shut branching airways that end blindly in small, thin- walled, well-vascularized outpocketings, the alveoli. by emptying entirely into the airways of the lung. This possibility The airways exhibit 23 levels of branching in the may sound like a remote conjecture from a physics book. In fact, human adult lung, giving rise to 500 million alveoli. however, until this application of physics to biology was appreci- The airways in a mammalian lung are categorized as ated, thousands of human babies died every year because of bubble conducting airways, where little gas exchange with the physics, as we discuss soon. blood occurs, and respiratory airways, where most gas The alveoli in normal lungs do not behave as simple aqueous exchange with the blood takes place. bubbles because of the presence of a complex mixture of metabolically Because of the blind-ended structure of the mammalian produced lipids and proteins called pulmonary surfactant (surfactant, lung, the gas in the alveoli always has a substantially “surface active agent”). About 90% of pulmonary surfactant is lipids, lower O2 partial pressure and higher CO2 partial mostly phospholipids (amphipathic molecules, as seen in Chapter pressure than atmospheric air. 2). Pulmonary surfactant is synthesized by specialized pulmonary Contraction of the diaphragm is a principal force for epithelial cells, which secrete the lipoprotein complex as vesicles. inhalation in mammals, especially large quadrupeds. After secretion, phospholipids from the vesicles associate with the External intercostal muscles may contribute to inhalation; internal intercostal muscles and abdominal surface of the thin water layer that lines each alveolus, where they muscles may contribute to exhalation. Inhalation occurs radically alter the surface-tension properties of the water. Their overall by suction as the lungs are expanded by contraction of effect is to reduce the surface tension below that of pure water. This inspiratory muscles. At rest, exhalation occurs passively effect in itself helps prevent alveoli from collapsing shut because the by elastic rebound of the lungs to their relaxation tendency of bubbles to collapse decreases as their surface tension volume when the inspiratory muscles relax. decreases (explaining why soap bubbles linger longer than bubbles The breathing rhythm in mammals originates in a of pure water). The most profound effect of pulmonary surfactant, central pattern generator in the pre-Bötzinger complex however, is that it gives the alveoli a dynamically variable surface in the medulla of the brainstem. tension. With surfactant present, as an alveolus enlarges, its surface The most potent chemosensory stimulus for increased tension increases, an effect that impedes further enlargement and ventilation in mammals is a rise in blood CO2 partial helps prevent the alveolus from expanding without limit. Conversely, pressure and/or H+ concentration, sensed in the as an alveolus decreases in size, its surface tension decreases, helping medulla. The blood O2 partial pressure, ordinarily a less to prevent any further decrease in size. Surfactant, therefore, helps influential factor in controlling ventilation, is sensed keep all alveoli similar in size. by the carotid bodies along the carotid arteries (humans) or by carotid and aortic bodies (certain other Infants born prematurely sometimes lack adequate amounts of mammals). The control of ventilation during exercise pulmonary surfactant. Their alveoli therefore lack the protections involves stimuli generated in association with limb of surfactant, and many alveoli collapse shut during each exhala- movement as well as chemosensory controls. tion. An affected infant then must inhale with sufficient force to Pulmonary surfactant, a mix of lipids and proteins that reopen the alveoli. The process is tiring and damages the alveoli. affects surface tension, makes a critical contribution to Death rates were very high until therapies based on knowledge of maintaining the proper microscopic conformation of pulmonary surfactant were introduced. the lungs in all air-breathing vertebrates. The use of knockout mice and other genomic methods is leading to rapid advances in understanding of the surfactant proteins. One of them, protein B, is now known to be essential for life, evidently Breathing by Birds because it plays indispensable roles in controlling the distribution The lungs of birds, although they are logical derivatives of the of surfactant lipids. types of lungs thought to exist in the common ancestors of birds Pulmonary surfactants that share basic chemical similarities have and mammals, differ in fundamental structural features from the been reported from the lungs of all groups of terrestrial vertebrates, lungs of mammals and all other modern vertebrates except certain lungfish, and some other air-breathing fish. Thus the pulmonary crocodilian reptiles. The structural difference between avian and surfactants have a long evolutionary history, dating back at least mammalian lungs inevitably invites comparison. Are avian lungs 8 This is an implication of Laplace’s Law, which—applied to bubbles— functionally superior to mammalian lungs? Some authorities states that ΔP = 2T/r, where r is the radius of a bubble, ΔP is the pressure conclude that the designs of the lungs in birds and mammals are difference between the inside and outside of the bubble, and T is the “different but equal”—that is, equal in their gas-exchange ability. tension in the walls of the bubble. If everything is considered constant except ΔP and r, the pressure difference required to keep a bubble open is Other authorities conclude that the lungs of birds are in fact superior seen to increase as the radius r decreases. organs of gas exchange. As evidence, they point to the fact that bird (A) Anatomy Anterior secondary Parabronchi Posterior secondary External Respiration 623 bronchi bronchi Figure 23.24 Airflow in the lungs and air sacs of Anterior birds (A) Basic anatomy of the avian lung and its connec- air sacs tions with the air sacs. In this presentation, the anterior and posterior groups of secondary bronchi are each represent- Posterior ed as a single passageway. The tubes labeled parabronchi air sacs are those of the dominant paleopulmonal system. (B, C) Primary bronchus Mesobronchus Airflow during (B) inhalation (when the air sacs undergo Neopulmonal expansion) and (C) exhalation (when the air sacs undergo parabronchi compression). Air flows through (B) Inhalation the parabronchi from posterior to The anterior air anterior. sacs expand and fill with gas that