Lecture 4: Exoskeletons Robots PDF
Document Details
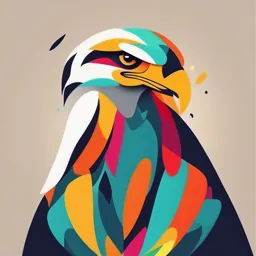
Uploaded by ProductiveGreekArt8850
Dr.Hagar El-Hadidy
Tags
Summary
This lecture provides an overview of exoskeletons robots used in rehabilitation therapy. It outlines the objectives of the lecture and discusses the different types of rehabilitation treatments. The lecture also discusses benefits of robot-assisted therapy (active assisting therapy).
Full Transcript
Lecture 4 EXOSKLETONS ROBOTS Dr.Hagar El-Hadidy Technology and Rehabilitation (ELE303) Objectives: At the end of this lecture, each student will be able to: 1- List the types of rehabilitation treatments and their differences. 2- Define the term of rehabilitation robots and how it...
Lecture 4 EXOSKLETONS ROBOTS Dr.Hagar El-Hadidy Technology and Rehabilitation (ELE303) Objectives: At the end of this lecture, each student will be able to: 1- List the types of rehabilitation treatments and their differences. 2- Define the term of rehabilitation robots and how it is classified. 3- Draw \examples of rehabilitation robots and their clinical results 4- Explain the electromyogram (EMG)-driven exoskeleton robots, The development of robotic devices for rehabilitation therapy can be seen as the logical progression of a stream of technological development activity begun by therapists themselves. Rehabilitation professionals have long taken an active interest in developing and using technology to assist in rehabilitation Implicit in the development of this technology was the idea of partial automation; that is, the technology might allow patients to practice some of the repetitive aspects of rehabilitation therapy on their own, without the continuous presence of the rehabilitation therapist. Some clinicians expressed unbelief toward the idea that robots could help them meet rehabilitation goals. Skeptical clinicians had good reasons to be skeptical that included the following points: 1) Robots cannot match therapists’ expertise and skill. Therapy involves manual skills that are learned over the course of years by experience under the guidance of expert mentors. Some of these skills require sophisticated manual manipulations of complex joints (e.g. mobilizing the patient’s scapula). An alert and perceptive therapist alters her therapy goals and assistance based on a complex, ongoing consideration of the patient’s state and progress. In brief: hands-on therapy requires expertise and is complex; it seems doubtful that a robot could replicate hands-on therapy effectively. 2) Robots are unsafe: robots are dangerous because they can move patient’s limbs but are not intelligent and sensitive to contra-indications to imposed movement like human therapists. They could move a patient in a harmful way. 3) Robots might replace therapists: Also implicit in the dubious reception by some therapists was a concern that robots might replace therapists Most engineers interested in robotic therapy probably never assumed that a robot could replace a therapist, because the job of a therapist is multifaceted and interpersonal, involving much more than just moving limbs. Rather, the goal in the mind of most engineers was consistent with that of therapists’ own previous technological developments : to provide a means for patients to practice therapy on their own so that they could get more therapy at less cost (i.e. partial automation). Motivation to continue the development of robotic devices for rehabilitation therapy : The researchers were intrigued by the potential of using robotic tools to improve understanding of motor control after neurologic injury and motor plasticity. Amid a growing need for stroke rehabilitation due to aging populations and increased stroke survival rates in industrialized countries, these devices offered a promising solution. Despite reductions in repetitive therapy due to cost-saving measures in healthcare, research showed that recovery could be positively influenced by activity and training. Robotic therapy devices were seen as a cost-effective way to provide more repetitive therapy to stroke survivors, opening up potential new business opportunities in the process. A Logical Target: Active Assist Exercise Despite uncertainties in the field, the research teams for MIT-MANUS, MIME, and the ARM Guide all chose active assist exercise as their therapeutic target for robotic therapy devices, a trend that continues. In this technique, patients actively attempt to move while therapists provide necessary assistance for them to perform the desired movements. This method was selected because it is a common element in many therapy schools and is easily observed in various rehabilitation clinics. Benefits of "robot-assisted therapy (active assist therapy): 1. Flexibility Enhancement: at the lowest level of motor control of biomechanics and reflexes, active assist exercise stretches soft tissue and muscles, which might be helpful for preventing contracture and reducing spasticity. 2. Plasticity Enhancement: at a middle level of motor control, active assist exercise provides the patient’s motor system with somatosensory stimulation that would normally not be available because the patient is paretic. Somatosensory input had recently been shown to drive cortical plasticity. 3. Motivation Enhancement: at a high level of the motor system, active assist exercise may motivate patients to exercise. If a patient cannot move well on his own, he are she may be disinclined to try to move. Active assist exercise allows the patient to be successful in achieving a desired movement, presumably motivating practice and effort.However, it should be noted, assisting too much with a robot may decrease effort. Robot-assisted rehabilitation provides a standardized environment, in which both therapy intensity and dose can be increased. In a conventional setting, hemiparetic patients typically perform about 30 movement repetitions with their affected upper limb in a 45-min session , whereas robot-assisted therapy has achieved over 1000 repetitions per session. Active physical and cognitive engagement of patients during therapy are crucial for recovery. This can be promoted through adaptive assistance , in a way to avoid slacking of the patient , as well as through cognitive challenge , automated task difficulty adaptation and motivating feedback. Feedback about movement performance can not only enhance motivation but also facilitate plasticity in the motor cortex if it arrives synchronously with motor output. But what about joints? A decision also had to be made about which joints of the upper extremity to focus on, as development of a robotic exoskeleton that can assist in all joint movements of the upper extremity was and remains an unsolved problem, especially for the hand and shoulder complex. The first robotic therapy devices for the upper extremity that were clinically tested (i.e. MIT- MANUS, MIME, and the ARM Guide, ) focused on providing active assist exercise for elbow flexion/extension and for limited shoulder movements (e.g. shoulder flexion below 90 degrees and limited external rotation). Three reasons for this choice were: 1) Simplicity: these joints were viewed as simpler than the hand, wrist, and complex shoulder movements. 2) Availability of tools: robots had already been developed to study motor control at these joints. 3) Pragmatism: the hand often appears to be hopelessly impaired following stroke, and shoulder problems such as subluxation are governed by complex biomechanical and neurological mechanisms which would be very difficult for a robot to address. Reaching movements with the arm are needed for a lot of functional activities. Rehabilitation treatment 1. Passive Therapy - Passive therapy requires no effort from the patient and is usually applied at the early stages of post-stroke symptoms, specifically when there is no response from the impaired limb. - Passive therapy is usually prescribed for hemiplegia patients that are suffering from one-side paralysis. It involves moving the impaired limb in a specific trajectory for a number of times during the session, which is usually performed by a rehabilitation robot (Figure ). The direction of the movement is preplanned carefully to avoid any harm that could affect the patient. Figure. Shows an example of passive therapy, where the impaired limb is moved according to the planned movement (flexion/extension of the upper limb at the elbow joint) by the rehabilitation robot. This kind of treatment focuses on stretching and contracting the impaired upper limb. It is also used to assess the range of motion of the limb. Exoskeleton robots are used in this treatment to provide repetitive motions according to the range of motion. A clinical study was carried out on passive therapy; three subjects were involved in the study and obtained a training session for 40 min. Passive therapy showed an effectiveness in reducing the spasms and the stiffness of the impaired limbs. 2. Active Therapy - This kind of treatment is prescribed for patients who are able to move their impaired limb to some limit. - The active term refers to the ability of moving the impaired limb but not efficiently. - Active therapy can be classified as active-assistive therapy or active- resistive therapy. - Active-assistive therapy involves applying an external force by a therapist or robot to help the patient fulfil the appointed task. It is also used to improve the range of motion. - Active-assistive therapy was applied to a patient with an impaired shoulder and elbow, where the patient was asked to reach a specific target, and the attached robot helped the patient complete the task (Figure ). The attached robot intervened once the patient was not able to perform the full task correctly and efficiently. - Active-resistive treatment, however, involves applying an opposing force on the impaired limbs. The opposing force can be applied by a therapist or robot (Figure ). Studies showed that the performances of patients become better gradually where the opposing force can be increased gradually. The opposing force is determined by an algorithm according to the ability of the patient. Figure. Represents patient trying to perform the abduction and the adduction of the upper limb. The weight helps the patient to perform the abduction which represents the Active-Assistive therapy. Also, the weight prevents the patient performing the adduction which represents active Resistive therapy. 3. Bilateral Therapy - Bilateral therapy refers to the mirroring principle in performing rehabilitation. Where the impaired limb copies the movement of the functional limb (Figure ), this gives the user whole control of the affected limb. - Mirror image movement enabler (MIME and a few other exoskeletons employ bilateral therapy. Figure. Shows an example of bilateral therapy, where one of the limbs is functional, and the other is impaired. The patient has the ability to freely move the functional limb, and the impaired one follows this movement. Table 1. Summary of the therapy types in terms of targeted patient, therapy procedure and limitations. Type of Treatment Targeted Patient Therapy Procedure Limitations Passive therapy hemiplegia patient Flexing and extending Beneficial for upper limb the impaired limb extremities Active-assisstive therapy Patient with some degree force is provided to help Beneficial for shoulder of moving ability the patient compete a and elbow task exercises Patient with some degree force is applied against Beneficial for shoulder Active resisted therapy of moving ability the desired and elbow movement exercises Bilateral therapy Patient has one Impaired limb copies the Beneficial for upper Functional limb trajectory of the limb extremities functional limb Classification of Rehabilitation Robots 1- According to Robot Treatment Approaches -Rehabilitation robots can be classified into two types, according to their treatment approaches. -The first approach is continuous passive movement (CPM). CPM requires no voluntary effort performed by the patient where the limb is controlled and moved by the robot. - PM treatment reduces muscle tone, which eventually improves the mobility of muscles, joints and tendons. Furthermore, PM activates the cortical area that has the corresponding sensorimotor and leads to an action similar to normal movement. Second approach is active-assisted movement, where the robot requires a signal from the patient to perform the movement. This signal could be an electromyogram (EMG) and follows the patient's intention to move the limb. Rehabilitation robots with active-assisted movement require voluntary effort from the patient and, consequently, provide significant motor improvement when they are compared to CPM rehabilitation. - The EMG-driven robots use an EMG signal an as "on-off" controller, which means that, once the patient voluntarily initiates the robot, the robot will repeat its action for a period of time. Then, the patient will be allowed another. 2-According to the Robot Structures - Rehabilitation robots are also classified as end-effectors and exoskeleton robots. - End-effectors are simple robots that have a distal movable handle, and the patient attaches his/her hand to this handle and follows a specific trajectory. This kind of robot is characterized by its ability to adapt to different sizes and shapes of movements, as the rehabilitation process requires. - End-effector robots have the disadvantage of not providing a rotation movement, which makes it not suitable for pronation and supination movements. - End-effectors have been developed recently to provide bilateral rehabilitation training, where the impaired limb copies the movement of the unimpaired limb in a synchronized behavior. Bilateral rehabilitation has the feature of activating the impaired hemisphere by making the left side and the right side of the body follow the same trajectory. - Exoskeleton robots are characterized by encapsulating the limb with a splint or bionic (electronic) structure. - Exoskeleton robots calculate the required torque for each joint and control the limb movements. - In comparison with end-effector robots, exoskeletons require a smaller working environment. - Exoskeleton robots, however, comprise the limb joint axes as they provide a very specific movement. In addition, exoskeleton robots are not feasible for bilateral rehabilitation, as the right limb exoskeleton cannot be used for the left limb, and eventually, it is expensive to design right and left exoskeleton robots to perform bilateral rehabilitation training. Examples of Rehabilitation Robots - Electromechanical implementation is one of the challenges to design robot, which refers to that the robot has to be light, durable, flexible and have the mechanism to perform a normal muscle motion. - The interpretation of user intent is another challenge, where the human-robot interaction plays an important role in the rehabilitation process. A neural muscle signal is one of the tools that is used to extract the user's intent. In addition, a robot's degrees of freedom (DOF) and the safety precautions should be taken into account in designing the rehabilitation robot; otherwise, the safety and the efficiency of the robot will be compromised. 1-MIT-MANUS- 1 -MIT-MANUS is an interactive workstation where the patient has to visually interact with a PC game to follow specific movements. The MANUS workstation provides five degrees of freedom, two translation degrees of freedom for the elbow and the forearm, represented by flexion/extension and pronation/supination, respectively. In addition, three degrees of freedom for the wrist, represented by flexion/extension, pronation/supination and abduction/adduction. A magnet safety lock is used to attach the patient's hand to the device; this technique provides an easy procedure to free the patient from the device. -is a PM robotic system - Furthermore, the MANUS workstation provides low torques. Therapy sessions, however, have to be monitored by a therapist to ensure the patient's safety. The MANUS workstation is characterized by its impedance control, which provides a specific movement to the limbs. -The MANUS workstation records the patient's performance (specifically, the force, velocity and the position); then, the performance data are sent to the control computer to adapt the robot according to the patient's requirements. -The MANUS workstation has been experimentally proven to provide therapeutic effects for upper limb impairment. 2-MIME- 2 - Mirror image movement enabler (MIME) is a PM robotic system that consists of a wheelchair and height-adjustable table, where the patient sits on the wheelchair and puts his/her affected limb on the adjustable table. The affected limb is strapped into the forearm splint that restricts the movements of the wrist and the hand. - A Puma 560 (Programmable Universal Machine for Assembly) robot was used as the manipulator, where the robot attached to the splint and applied force to the affected limb during its movements. The Puma 560 robot provided six degrees of freedom, which provided a large range of movement positions in a 3D space. - MIME, provides the three treatments modes that are the passive, active-assistive/active-resistive and bilateral modes. In addition, MIME is commonly used in the passive and active-resistive modes. In the passive mode, the robot moves the limb in a specific trajectory towards the target. The active-resistive mode involves prohibiting the limb's movements in the identified trajectory by providing a viscous resistance, and the patient has to provide maximal effort to reach the target. MIME is used to strengthen the muscles and improve the limb's motions. 3-The Assisted Rehabilitation and Measurement guide (ARM guide) The Assisted Rehabilitation and Measurement guide (ARM guide) is also an example of a PM rehabilitation robot and is used for the training and evaluating of upper limb functions. The ARM guide, shown in Figure , uses the reaching principle as a therapy technique, where the patient's arm is attached to a splint, and the patient is advised to reach for things. The ARM guide provides one degree of freedom on a linear constraint rather than multiple active degrees of freedom. Therefore, the ARM guide is considered as an inexpensive and a simple rehabilitation robot. The orientation of the ARM guide can be changed vertically or horizontally. 4-ARMin - ARM in is an upper limb rehabilitation system and is characterized by providing seven degrees of freedom allowing shoulder rotation in three dimensions; flexion/extension of the elbow, supination/pronation of the forearm, and flexion/extension of the hand. - ARMin consists of a chair and robotic arm, where the seated patient attaches his/her hand to the robotic arm and adjusts its length to its optimum, as shown in figure. - ARMin has three levels of safety, 1. first where the device is equipped by many sensors that work as a system to detect any malfunction that could arise. 2. Secondly, the ARMin has no sharp edges, and no joint will move out of the range of a human limb. 3. Thirdly, a unique algorithm was developed to monitor the repeated motions, and immediate shutdown happens in case of malware being found. Figure. ARMin rehabilitation robot. Source 5- Cable Actuated Dexterous Exoskeleton Cable Actuated Dexterous Exoskeleton for Neuro-Rehabilitation with Seven Degrees of Freedom (CADEN-7) - Cable actuated dexterous exoskeleton for neuro-rehabilitation (CADEN-7) provides seven degrees of freedom. CADEN-7 is considered an active-assisted robot that uses surface electromyography to control the upper limb. CADEN-7 is also equipped with three levels of safety, taking into consideration the electrical aspects, mechanical aspects and software aspects. -The unique design of CADEN-7 makes it suitable to avoid hyperextension and erratic motions. - CADEN-7, however, has to be fixed on external forms and cannot be portable due its size and weight. 6-L-EXOS -The Light Exoskeleton (L-EXOS) system focuses on providing repetitive movements and gives a simultaneous measurement for the therapeutic progress. L-EXOS, incorporates visual reality to guide the patient to a specific trajectory. L-Exos (Light Exoskeleton) is a force feedback exoskeleton for the right human arm. -In terms of safety concerns, L-EXOS provides a -velocity equal to 10 rpm (revolutions/min), and the maximum torque cannot exceed 120 Nm. In addition, L-EXOS provides five degrees of freedom, and each movement is equipped by a sensor. The L-Exos device was integrated with a projector used to display on a wide screen placed in front of the patient different virtual scenarios in which to perform rehabilitation exercises. The VR display is therefore a mono screen in which a 3D scene is rendered. The system is allowing different joint configurations corresponding to the same end- effector position, which is fundamental for chronic stroke patients. 7-Therapy Wilmington Exoskeleton (T-Wrex) - The therapy Wilmington exoskeleton (T-Wrex) is an active upper limb rehabilitation robot with five degrees of freedom. It is dedicated for individuals with significant arm weakness by providing intensive training. - The T-Wrex uses the feature of gravity compensation or antigravity for the entire arm, where the patient experiences the sense of a floating arm in space. In addition, the T-Wrex is characterized by providing a large 3D movement that naturalizes the upper limb movements. - The T-Wrex consists of two links attached to the forearm and upper arm. In terms of safety, position sensors and custom grip sensors are integrated to the robot, which provide movement measurements for the upper limb. 8-REHAROB The Rehabilitation Robot (REHAROB) therapeutic system is a passive rehabilitation robot with three degrees of freedom dedicated for the shoulder and the elbow. The movement trajectory is pre-programmed by the therapist and followed by repetitive movement for the impaired limb. The exercises performed by REHAROB are different than those performed by MIT-MANUS and MIME by providing slow repetitive movements with a constant velocity. These kinds of exercises are efficient in reducing spasticity and increasing the range of mobility for the shoulder and elbow joints. The patient's upper arm and forearm are attached to the robot's arm. The REHAROB is equipped by sensors to control and monitor the generated forces. 9-(BONES)Exoskeleton Biomimetic Orthosis for Neurorehabilitation. -The Biomimetic Orthosis for Neurorehabilitation (BONES) is an active rehabilitation robot with four degrees of freedom The BONES is characterized by its ability to provide arm internal/external rotations without the need to bring or use any bearing element. The unique design was inspired by human biomechanics. In addition, the BONES occupies five actuators to provide a wide range of motion for the upper limb and further measurements for safety issues. Table 2. Characteristics of the rehabilitation robots. DOF: degrees of freedom, MIME: mirror image movement enabler, ARM: Assisted Rehabilitation and Measurement, CADEN-7: cable actuate dexterous exoskeleton for neuro-rehabilitation with seven degrees of freedom, T-Wrex: therapy Wilmington exoskeleton and BONES: Biomimetic Orthosis for Neurorehabilitation. Upper panel: Evolution of upper extremity rehabilitation robots. From stiff (high impedance) industrial manipulators to dedicated rehabilitation robots providing control at the distal effector or over each joint, including the rendering of virtual object dynamics resulting in somatosensory feedback. Further evolution of the technology will see wearable systems providing support not only during therapy sessions, but also during activities of daily living in the home environment, allowing physical interaction with real objects. Lower panel: Task-specific design of hand rehabilitation robots. Functional hand movement training should focus not only on unimanual, i.e. reach and grasp tasks (left), but should also include bimanual separate tasks (middle), as well as cooperative movement tasks that are employed, e.g., when opening a bottle (right)